Abstract
Background:
Mesenchymal stem cells (MSCs) survival decreased following in vivo injection and its application has been limited in stem cell therapy. Preconditioning was established as a strategy to increase the cells’ efficiency, but more studies are necessary to determine its safety and mechanisms.Objectives:
The aim of this study was to evaluate the effect of preconditioning mesenchymal stem cells with low serum and H2O2 on in vitro cell survival.Methods:
Mesenchymal stem cells were cultured and preconditioned with low serum and H2O2 for 6, 12, 24 and 48 hours in six groups. Control, group I with 5 μM H2O2, group II with 10 μM H2O2, group III with 0.5% fetal bovine serum (FBS), group IV: 5 μM H2O2 + 0.5% FBS and V: 10 μM H2O2 + 0.5% FBS. Cell proliferation was evaluated with the MTT assay.Results:
Cell proliferation in groups IV and V increased significantly compared to the other groups after 6, 12 and 24 hours of treatment (P < 0.05). Also, after 48 hours, cell treatment with 5 μM H2O2+ 0.5% FBS led to a significant increase in cell proliferation (P < 0.05).Conclusions:
Our data suggest that preconditioning with 5 μM H2O2 + 0.5% FBS and 10 μM H2O2 + 5% FBS improved cell proliferation and viability. However, the mechanisms related to protective properties of preconditioning and using this strategy in stem cell therapy requires more research.Keywords
Mesenchymal Stem Cell Proliferation Cell Survival Preconditioning
1. Background
Mesenchymal stem cells (MSCs) are multi-potent cells, which can differentiate into mesenchymal and trans-differentiate to non-mesenchymal tissues in vitro and in vivo (1). They are found in specialized tissues of the body like the brain, bone marrow, Wharton jelly, pulp and umbilical cord blood (2). Stem cells and especially MSCs produce all types of multicellular tissues in the body via proliferation and differentiation in controlled in vivo environments. These cells are characterized by their easy access, expanding potential in vitro and features that are not easily lost (3). Bone-marrow mesenchymal stem cells (BMSCs) are non-hematopoietic stromal cells with powerful proliferative and differential potential and represent interesting modalities for the establishment of efficient stem cell-based therapy in various diseases. In the recent years, there has been growing interest in MSC application in cell-based therapy (4-6). However, MSCs-based therapy faces some limitations such as low proliferation and survival rate (7-9) after replacement in an in vivo environment (10-12). Following MSCs transplantation, the cells are faced with factors such as low serum, hypoxia, heat shock, and oxygen free radicals. This environment-induced cell death and apoptosis hours after transplantation (13). There are several strategies to improve survival rate, potency, migration and targeting of MSCs. Some studies have reported that preconditioning of MSCs by cultivation under H2O2, hypoxic and serum deprivation as well as sub-lethal stress environment improves the survival and proliferation rates, differentiation and migration capacities (14, 15). Preconditioning using H2O2 is the most practical and important protocol applied in preclinical studies. It has been suggested that preconditioning exerts its effects through altering intrinsic signaling pathways of growth factors, cytokines, and chemokines and expression level of their receptors and consequently improves stem cells survival and proliferation rate (16, 17). Treatment of MSCs with low concentrations of H2O2 for a short period of time adapts them to harmful conditions. It protects MSCs against oxidative damage upon their exposure to high concentrations of reactive oxygen species (ROS) and prevents apoptosis induction as well (18). Enhancement of heart function and decrease of myocardial fibrosis have been reported following transplantation of H2O2 preconditioned MSCs to infracted myocardium in mice (19). Interestingly, H2O2 preconditioning decreases serum deprivation (SD)-induced apoptosis.
2. Objectives
In the present study, protective properties of co-preconditioning of MSCs with low serum and H2O2 were investigated in in vitro.
3. Methods
3.1. Mesenchymal Cells Isolation and Culture
Mesenchymal stem cells (MSCs) were obtained from the femoral and tibial bones of six-week-old Wistar Albino male rats. These cells were suspended in low glucose Dulbecco’s modified eagle medium (DMEM) medium with 10% fetal bovine serum (FBS) (Gibco, Invitrogen, CA, USA) and incubated at 37°C in a humidified chamber with 5% CO2. The culture medium was completely replaced every three days and non-adherent cells were removed. Mesenchymal Stem Cells were recognized by their capability to proliferate in culture with an attached well-spread morphology. Once cells were more than 80% confluent, adherent cells were trypsinized and re-plated 1: 3 (passage1). The differentiation potency of MSC was confirmed by induction of osteogenic, chondrogenic and adipogenic differentiation using specific media.
3.2. Differentiation of Bone Marrow Mesenchymal Stem Cells (BMMSC)
The multi-potency of bone marrow mesenchymal stem cells (BMMSC) was confirmed by induction of osteogenic, chondrogenic and adipogenic differentiation using specific differentiation media.
3.3. Osteogenic Differentiation
For osteogenic differentiation, BMMSCs were seeded at density of 2 × 104 cells/cm2 to a 24-well plate and then induced to differentiate in an osteogenic induction medium composed of DMEM with 10% FBS (Gibco, Invitrogen, CA, USA), 100 units/mL penicillin and 100 g/mL streptomycin, 10 nM dexamethasone, 50 µg/mL ascorbic acid, 10 mM -glycerophosphate. The cells were incubated for 21 days in 5% CO2 at 37°C. After 21 days, osteoblast differentiation was evaluated by 2% Alizarin Red (Sigma) staining. Briefly, Cells were washed three times with Phosphate Buffer Saline (PBS) (Gibco, Invitrogen, CA, USA) and then fixed for 15-30 minutes with 4% formaldehyde. After rinsing three times with distilled water, they were stained with Alizarin red for three minutes. The cells were washed with distilled water several times and examined under the microscope.
3.4. Adipogenic Differentiation
The cells were seeded at a density of 2 × 104 cells/cm2 in 24-well plates. After 80% confluency, the cells were exposed to adipogenic induction medium, consisting of DMEM supplemented with 10% FBS, 100 units/mL penicillin and 100 g/mL streptomycin, 100 nM dexamethasone, 50 µg/mL indomethacin for 10 days and fat droplets were examined under the microscope.
3.5. Chondrogenic Differentiation
The cells were cultured at a density of 2 × 104 cells/cm2 in 24-well plates. Chondrogenic induction medium was added to cells for 21 days. Medium was changed twice a week. After 21 days, the cells were stained with Alcian blue (Sigma). Briefly, the wells were washed with PBS and fixed with 4% paraformaldehyde for 15 - 30 minutes. Then, the cells were stained with Alcian blue for 30 minutes and washed with HCL 0/1 N. The plate was examined under a light microscope.
3.6. Preconditioning of Mesenchymal Stem Cells
The cells were cultured in 96-well plates at concentration of 104 cells/well. The experiment was designed with six groups. The control group received DMEM with 10% FBS, group I received 5 μM H2O2, group II received 10 μM H2O2, group IV received 0.5% serum and group V received 5 μM H2O2 + 0.5% serum. All groups were cultured and incubated for 6, 12, 24 and 48 hours in 37°C with 5% CO2. Then, all groups were exposed to a lethal dose of 300 μM of H2O2 for 24 hours followed by 24 hours of incubation with fresh medium composed of DMEM and 10% FBS as a recovery period. Finally, MTT assay was performed to detect proliferation of the cells in different groups.
3.7. MTT Assay
Cell proliferation evaluation was confirmed by MTT assay. Briefly, 100 µL of the culture medium was removed and 15 μL MTT 3-(4, 5-dimethylthiazol-2-yl)-2, 5-diphenyltetrazolium bromide (5mg/ml) was added. After three hours, the wells were replaced with 200 μL of dimethyl sulfoxide (DMSO). The wells were pipetted and read with a spectrophotometer (ELISA reader, TECAN/ sunrise, Magellan program, Austria) at optical density of λ570. Percentage of viable cells was measured by:

3.8. Statistical Analysis
All results were expressed as mean ± standard deviation. Statistical analyses were performed by one-way analysis of variance (ANOVA) using SPSS software version 20. The differences between means was considered statistically significant when the probability level (P value) was less than 0.05 (P < 0.05).
4. Results
4.1. Morphology of Mesenchymal Stem Cells
After one week, the attached cells were found in the flasks. The cells were attached to plastic and their morphology was fibroblast like (Figure 1).
A, The Primary Culture of the Cells After one week and B, at Passage 4
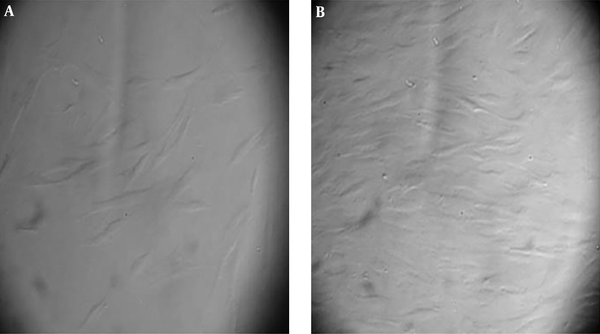
4.2. Differentiation of Mesenchymal Stem Cells
The cells were cultured with differentiation media for 21 days (osteogenesis and chondrogenesis) and 10 days for adipogenesis. As it is illustrated in Figure 2 the MSCs differentiated to bone, adipose and cartilage tissue (Figure 2 A-C).
A, The Cells Were Cultured in Differentiation Media for Adipogenesis for Ten Days, B, Osteogenesis for 21 Days and C, Chondrogenesis for 21 Days
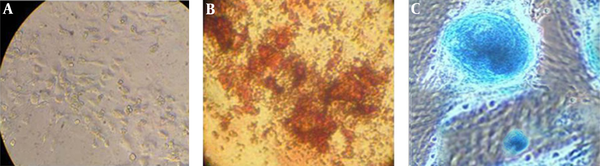
4.3. Improvement of Cell Proliferation After Preconditioning
Cell proliferation rate of different groups after preconditioning is shown in Figure 3. It is clear that cell proliferation increased in all preconditioned groups compared to the control. Also, it is indicated that cell proliferation in groups IV (5 μM H2O2 + 5% FBS) and V (10 μM H2O2 + 5% FBS) increased significantly compared to the other groups after 6, 12 and 24 hours of treatment (P < 0.05). Moreover, significant increase in cell proliferation was observed only in group IV (5 μM H2O2+ 5% FBS) towards the other groups after 48 hours (P < 0.05) (Figure 3).
The Cells Were Cultured in 96-Well Plates to Analyze Their Proliferation by the MTT Assay
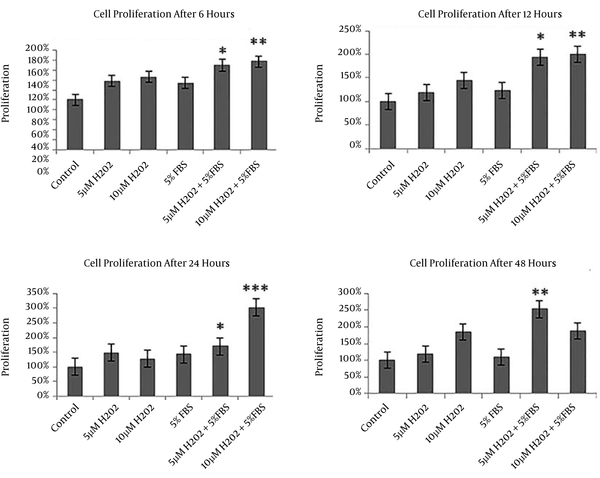
5. Discussion
In spite of its benefits, cell therapy for treatment of different diseases has been limited by poor survival ability of MSCs and their cell death due to in vivo ischemic environment (8, 17, 20), therefore, it is essential to discover a novel strategy to improve the efficiency of MSCs for successful stem cell transplantation. In this regard, the present study investigated the MSCs survival ability following preconditioning with cultivation under hypoxic and serum deprivation conditions. The major finding of this study was the improvement of MSCs proliferation after preconditioning with H2O2 and serum deprivation. Preconditioning with low concentrations of H2O2 and FBS deprivation effectively enhanced the proliferation of MSCs in a dose and time-dependent manner. Several in vitro and in vivo studies reported increase of MSCs survival after preconditioning under hypoxic and serum deprivation conditions (15, 21). Indeed, some studies have shown that rate of successful engraftment and differentiation capacity of the pretreated MSCs was higher than non-preconditioned controls (14, 22). In some studies, higher viability rate and clonogenic capacity was reported after serum deprivation-treated MSCs consequently cultivated in presence of FBS (16, 23). Also, it has been stated that MSCs cultured in serum deprivation conditions show normal morphology (24). However, the detailed mechanisms involved in MSCs protection following preconditioning are not fully determined. It is suggested that, alteration of intrinsic signaling pathways of some growth factors, cytokines, and chemokines and the expression levels of their receptors (especially CXCR4) due to preconditioning might increase their efficiency (25). Preconditioning with H2O2 facilitates stem cell differentiation, heart regeneration, and enhancement of early cardiac development by activating the Notch and Wnt signaling pathways, and it also prevents induction of apoptosis in MSCs (26). Low H2O2 concentration enhances expression of CXCR4 at gene and protein levels and also facilitates the interaction between CXCR4 and its ligand, and stromal cell derived factor-1α (SDF-1α) promotes migration capability of MSCs (27).
In spite of its efficiency in preclinical studies, its application in clinical trials needs more attention in regards to safety of preconditioning.
5.1. Conclusions
Our findings confirmed that preconditioning with H2O2 as well as serum deprivation improves MSCs proliferation ability.
Acknowledgements
References
-
1.
da Silva Meirelles L, Chagastelles PC, Nardi NB. Mesenchymal stem cells reside in virtually all post-natal organs and tissues. J Cell Sci. 2006;119(Pt 11):2204-13. [PubMed ID: 16684817]. https://doi.org/10.1242/jcs.02932.
-
2.
Kolf CM, Cho E, Tuan RS. Mesenchymal stromal cells. Biology of adult mesenchymal stem cells: regulation of niche, self-renewal and differentiation. Arthritis Res Ther. 2007;9(1):204. [PubMed ID: 17316462]. https://doi.org/10.1186/ar2116.
-
3.
Nussler A, Konig S, Ott M, Sokal E, Christ B, Thasler W, et al. Present status and perspectives of cell-based therapies for liver diseases. J Hepatol. 2006;45(1):144-59. [PubMed ID: 16730092]. https://doi.org/10.1016/j.jhep.2006.04.002.
-
4.
Law S, Chaudhuri S. Mesenchymal stem cell and regenerative medicine: regeneration versus immunomodulatory challenges. Am J Stem Cells. 2013;2(1):22-38. [PubMed ID: 23671814].
-
5.
Wei X, Yang X, Han ZP, Qu FF, Shao L, Shi YF. Mesenchymal stem cells: a new trend for cell therapy. Acta Pharmacol Sin. 2013;34(6):747-54. [PubMed ID: 23736003]. https://doi.org/10.1038/aps.2013.50.
-
6.
Murphy MB, Moncivais K, Caplan AI. Mesenchymal stem cells: environmentally responsive therapeutics for regenerative medicine. Exp Mol Med. 2013;45:54. [PubMed ID: 24232253]. https://doi.org/10.1038/emm.2013.94.
-
7.
Han SM, Han SH, Coh YR, Jang G, Chan Ra J, Kang SK, et al. Enhanced proliferation and differentiation of Oct4- and Sox2-overexpressing human adipose tissue mesenchymal stem cells. Exp Mol Med. 2014;46:101. [PubMed ID: 24946789]. https://doi.org/10.1038/emm.2014.28.
-
8.
Liu XB, Wang JA, Ogle ME, Wei L. Prolyl hydroxylase inhibitor dimethyloxalylglycine enhances mesenchymal stem cell survival. J Cell Biochem. 2009;106(5):903-11. [PubMed ID: 19229863]. https://doi.org/10.1002/jcb.22064.
-
9.
Yoon DS, Kim YH, Jung HS, Paik S, Lee JW. Importance of Sox2 in maintenance of cell proliferation and multipotency of mesenchymal stem cells in low-density culture. Cell Prolif. 2011;44(5):428-40. [PubMed ID: 21951286]. https://doi.org/10.1111/j.1365-2184.2011.00770.x.
-
10.
Li Q, Wang Y, Deng Z. Pre-conditioned mesenchymal stem cells: a better way for cell-based therapy. Stem Cell Res Ther. 2013;4(3):63. [PubMed ID: 23739590]. https://doi.org/10.1186/scrt213.
-
11.
Francois S, Mouiseddine M, Allenet-Lepage B, Voswinke LJ, Douay L, Benderitter M. Human mesenchymal stem cells provide protection against radiation-induced liver injury by antioxidative process, vasculature protection, hepatocyte differentiation, and trophic effects. BioMed research international; 2013.
-
12.
Xu J, Qian J, Xie X, Lin L, Zou Y, Fu M, et al. High density lipoprotein protects mesenchymal stem cells from oxidative stress-induced apoptosis via activation of the PI3K/Akt pathway and suppression of reactive oxygen species. Int J Mol Sci. 2012;13(12):17104-20. [PubMed ID: 23443132]. https://doi.org/10.3390/ijms131217104.
-
13.
Liu NS, Du X, Lu J, He BP. Diva reduces cell death in response to oxidative stress and cytotoxicity. PLoS One. 2012;7(8):43180. [PubMed ID: 22905226]. https://doi.org/10.1371/journal.pone.0043180.
-
14.
Jaderstad J, Brismar H, Herlenius E. Hypoxic preconditioning increases gap-junctional graft and host communication. Neuroreport. 2010;21(17):1126-32. [PubMed ID: 20938361]. https://doi.org/10.1097/WNR.0b013e328340a77b.
-
15.
Oh JS, Ha Y, An SS, Khan M, Pennant WA, Kim HJ, et al. Hypoxia-preconditioned adipose tissue-derived mesenchymal stem cell increase the survival and gene expression of engineered neural stem cells in a spinal cord injury model. Neurosci Lett. 2010;472(3):215-9. [PubMed ID: 20153400]. https://doi.org/10.1016/j.neulet.2010.02.008.
-
16.
Amiri F, Jahanian-Najafabadi A, Roudkenar MH. In vitro augmentation of mesenchymal stem cells viability in stressful microenvironments. Cell Stress Chaperones. 2014;20(2):1-15.
-
17.
Lu H, Li Y, Sheng Z, Wang Y. Preconditioning of stem cells for the treatment of myocardial infarction. Chinese Med J. 2012;125(2):378-84.
-
18.
Li D, Xu Y, Gao C, Zhai Y. Adaptive protection against damage of preconditioning human umbilical cord-derived mesenchymal stem cells with hydrogen peroxide. Genetics Molecular Res. 2014;13.
-
19.
Pendergrass KD, Boopathy AV, Seshadri G, Maiellaro-Rafferty K, Che PL, Brown ME, et al. Acute preconditioning of cardiac progenitor cells with hydrogen peroxide enhances angiogenic pathways following ischemia-reperfusion injury. Stem Cells Dev. 2013;22(17):2414-24. [PubMed ID: 23544670]. https://doi.org/10.1089/scd.2012.0673.
-
20.
Swanger SA, Neuhuber B, Himes BT, Bakshi A, Fischer I. Analysis of allogeneic and syngeneic bone marrow stromal cell graft survival in the spinal cord. Cell Transplant. 2005;14(10):775-86. [PubMed ID: 16454352].
-
21.
He A, Jiang Y, Gui C, Sun Y, Li J, Wang JA. The antiapoptotic effect of mesenchymal stem cell transplantation on ischemic myocardium is enhanced by anoxic preconditioning. Canadian J Cardiol. 2009;25(6):353-8.
-
22.
Ren H, Cao Y, Zhao Q, Li J, Zhou C, Liao L, et al. Proliferation and differentiation of bone marrow stromal cells under hypoxic conditions. Biochem Biophys Res Commun. 2006;347(1):12-21. [PubMed ID: 16814746]. https://doi.org/10.1016/j.bbrc.2006.05.169.
-
23.
Pochampally RR, Smith JR, Ylostalo J, Prockop DJ. Serum deprivation of human marrow stromal cells (hMSCs) selects for a subpopulation of early progenitor cells with enhanced expression of OCT-4 and other embryonic genes. Blood. 2004;103(5):1647-52. [PubMed ID: 14630823]. https://doi.org/10.1182/blood-2003-06-1967.
-
24.
Fu WL, Jia ZQ,, Wang WP, Zhang JY, Fu X, Duan XN. Proliferation and apoptosis property of mesenchymal stem cells derived from peripheral blood under the culture conditions of hypoxia and serum deprivation. Chinese Med J Beijing. 2011;124(23):3959.
-
25.
Lu HH, Li YF, Sheng ZQ,, Wang Y. Preconditioning of stem cells for the treatment of myocardial infarction. Chinese Med J. 2012;125(2):378-84.
-
26.
Boopathy AV, Pendergrass KD, Che PL, Yoon YS, Davis ME. Oxidative stress-induced Notch1 signaling promotes cardiogenic gene expression in mesenchymal stem cells. Stem Cell Res Ther. 2013;4(2):43. [PubMed ID: 23597145]. https://doi.org/10.1186/scrt190.
-
27.
Li S, Deng Y, Feng J, Ye W. Oxidative preconditioning promotes bone marrow mesenchymal stem cells migration and prevents apoptosis. Cell Biol Int. 2009;33(3):411-8. [PubMed ID: 19356708]. https://doi.org/10.1016/j.cellbi.2009.01.012.