Abstract
Background:
Today, wireless communication systems are destructive with increased lipid peroxidation and oxidation state and have adverse biological effects on human health.Objectives:
In this study, we examined the effects of exposure to WiFi wireless frequency (2.4 GHz) on histopathological changes in the cardiovascular system of rats.Methods:
The experimental groups included 32 adult male rats divided into control (not exposed to heat and WiFi), WiFi (exposed to 2.45 GHz for 52 consecutive days (2 h/day)), heat (water bath of 43°C for 52 consecutive days (10 min/day)), and heat+WiFi groups (exposed to 2.45 GHz then water bath of 43°C). On the 52nd day, the heart was removed, and its total volume and weight were determined using stereological techniques. The number of cardiomyocytes nuclei and the volume of the myocardium were determined. Blood samples were collected to measure reduced glutathione (GSH) content, Total Antioxidant Capacity (TAC), and malondialdehyde level (MDA). Data were analyzed by ANOVA, Kruskal-Wallis, and Mann-Whitney U tests.Results:
The heart weight and volume density of the myocardium increased in the WiFi-irradiated group compared to the control group (P < 0.05). Also, exposure to WiFi increased MDA levels and decreased TAC and GSH compared to the control group (P < 0.05).Conclusions:
This study indicated that RFW might cause structural changes and oxidative stress in the heart. Also, exposure to radiofrequency decreased total antioxidant activity in heart tissue with histological changes, including myocardium hypertrophy and decreased number of myocytes.Keywords
1. Background
Exposure to WiFi has rapidly increased due to the increasing number of wireless devices. Microwaves were introduced as an eventual carcinogen in 2011 by the International Agency for Research on Cancer (IARC). Despite that, the health effects of MW exposure concerning cancer or other diseases remain disputed (1). Radiofrequency radiation, a non-ionizing part of the Electromagnetic Field (EMR) ranging from 300 MHz to 300 GHz, has made communication possible between billions of wireless users worldwide (2-4). There is an increase in the use of WiFi operation at 2.45 GHz by local networks at home, work, and hotspots (3). The evolution of this technology has been accompanied by great concern regarding its biological effect on human health, specifically Radiofrequency Field (RF) exposure (5, 6). These effects were mainly related to WiFi heating, which could produce various molecular transformation types (7).
Several publications reported the adverse health effects of EMR, including cancer, leukemia, brain tumors, immune system deregulation, immunologic perturbation, blood-brain barrier defects, neurological effects, and some cardiovascular effects (8, 9). The EMR changes the energy level of electrons and increases the activity, half-life, and concentration of Reactive Oxygen Species (ROS) (10). The mechanism for the increased ROS generation is the depletion rate of ATP synthesis, the generation of proteolytic enzymes, DNA damage, and cardiac remodeling, leading to hypertrophic signaling, necrosis, and apoptosis (11, 12). The researcher reviewed the epidemiological studies with the general conclusion that exposure to EMR could directly (non-thermal) or indirectly (thermal) alter the cardiovascular system (13). Recently, studies have shown that the MDA levels were significantly higher in WiFi-exposed (2.4 GHz) rats than in controls (14).
2. Objectives
For this purpose, we evaluated and compared the effects of heat, WiFi radiation, and WiFi-heat exposure on the cardiovascular system of rats.
3. Methods
3.1. Experimental Design and Exposure System
In this study, we selected 32 male Sprague-Dawley rats weighing 210 - 240 g. All animal procedures were carried out per the standard rules established by the University Animal Care and Ethics Committee. The rats were first randomly divided into four groups: group I: rats not exposed to heat or WiFi, group II: rats exposed to WiFi at 2.45 GHz for 52 consecutive days (2 h/day), group III: rats exposed to heat in a water bath at 43°C for 52 consecutive days (10 min/day), group IV: rats exposed to WiFi at 2.45 GHz and then a water bath at 43°C (14).
3.2. WiFi 2.45 Exposure System
Four antennas (2.45 GHz) were placed on each side of the room. Rat's Plexiglas cages (40 cm × 40 cm × 20 cm) were placed in the middle of the room while the animals had movement freedom.
3.3. Stereological Study
For stereological evaluation, the heart was removed, and the primary volume of the heart "V (Heart)" was measured using the immersion method according to the Scherle method (1970). The degree of shrinkage "d (shr)" was determined based on the Cavalieri principle. Estimating "d (shr)" and tubule length requires isotropic random slices. These slices were based on the "orientator method." Ultimately, 8 - 12 slices were collected from each heart. Then, the slices and circular pieces were processed, sectioned (4 and 25 µm thicknesses), and stained with Hematoxylin-Eosin (Figure 1).
The method of point-counting for determining the volume of the myocardium and the number of points hitting the structure
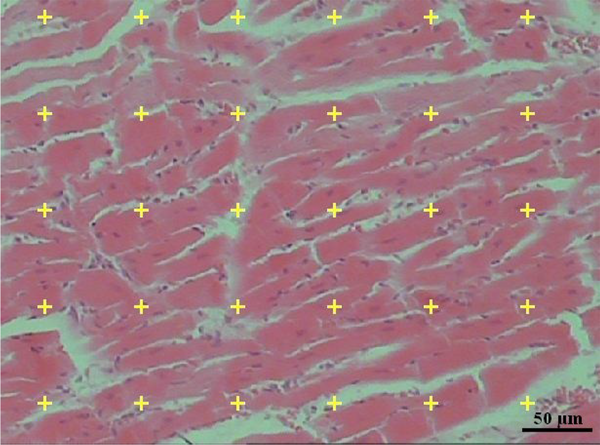
d (shr)= 1 - (AA/AB)1.5
where AA and AB represent the area of the circular piece after and before processing and staining, respectively. Sections were analyzed using a video microscopy system. In doing so, the microscopic fields were sampled using a systematic random sampling procedure. Then, the stereological test grids (point grid and unbiased counting frame) were superimposed on the microscopic images on a monitor utilizing the stereology software designed at the university (Figure 1).
3.3.1. Estimating Heart Volume and Myocardium
The heart's primary volume and the myocardium's volume were determined based on the Cavalieri method. The volume was obtained using the following formula:
V(primary)= ΣP(tissue)×a(p)×T
where "ΣP" is the points on the surface of the heart, "(a/p)" is the area relative to each point, and "T" is the thickness of the slice.
The mean heart shrinkage was determined by:
d(shri) =1- (AA/AB)1.5
The final volume was obtained using the following formula:
V(final)=V(primary)×[1−d(shri)]
3.3.2. Estimation of the Numerical Density of Cardiomyocytes Nuclei
The numerical density of cardiomyocytes nuclei, NV (nuclei/heart), in 25-µm-thick paraffin sections was determined using the following formula:
NV (nuclei/heart)= [ΣQ−/(ΣP. (a/f). h)]. (t/BA)
"ΣQ−": Total number of nuclei
"ΣP": Total points putting on the myocardium tissue
"a/f": The area of the frame
"h": The height of the dissector
"t": The mean section thickness (Figure 2)
The dissector method for numerical density of cardiomyocytes nuclei
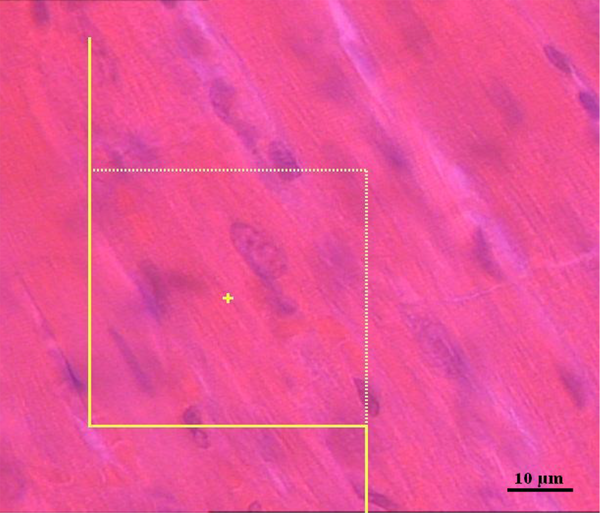
3.4. Oxidant/Antioxidant Status Assessment
3.4.1. Determination of Lipid Peroxidation
The MDA levels in heart tissue were determined by the reaction of thiobarbituric acid with lipid peroxidation breakdown products as described previously by Devrim et al. (15). First, 0.5 mL tissue samples were added to 2 mL thiobarbituric acid. Then, the mixture was placed at 95°C for one hour in a Ben Marie and then for 15 min in ice, centrifuged for about 10 min at 4000 rpm; then, butanol pyridine (15:1, V/V) (1 mL) was added. After centrifugation, the supernatant was measured spectrophotometrically at 535 nm. The malondialdehyde concentration in micro-molar was obtained from the standard curve prepared with 1 and 3 tetraethoxypropane.
3.4.2. Total Antioxidant Capacity (TAC) Measurement
The TAC levels were measured by the FRAP assay described by Benzie and Strain (16) with slight changes. In this method, the colorless Fe3+ of ferric tripyridyltriazine (Fe3+-TPTZ) complex was reduced to the ferrous ion (Fe2+). At low pH, this reduction forms an instance blue color with a maximum absorbance of 593 nm. The working FRAP reagent included (A): 10 mM TPTZ in 40 mM HCl and (B) Acetate buffer solution: 300 mM (pH 3.6). First, A and B reagents were mixed; then, a 100 µL sample was added to the working FRAP reagent, and a spectrophotometer determined the color absorbance at 593 nm.
3.4.3. Reduced Glutathione (GSH) Content Determination
The reduced glutathione content in the tissue was spectrophotometrically measured using the procedure described by Gaitanaki et al. (17). In general, to determine GSH content, the protein supernatants were mixed with sodium phosphate buffer 0.02 M and DTNB (5,5′-Dithiobis-2-nitrobenzoic acid) 10 mM. They stayed for 2 min at room temperature. After five minutes, the absorbance was determined against a non-homogenized blanch.
3.5. Statistical Analysis
Data were analyzed with Mann-Whitney U, Kruskal Wallis, and ANOVA tests.
4. Result
4.1. Heart Weight
According to Figure 3A, heart weight increased in the heat+WiFi-irradiated and WiFi-irradiated groups compared to the control group (P < 0.04). Also, heart weight increased in the heat+WiFi-irradiated group compared to the WiFi-irradiated group (P < 0.04).
Mean ± SEM of the heart weight (A), heart volume (B), volume density of myocardium (C), and the total number of myocytes (D) in the study groups (* P < 0.05 and # P < 0.03).
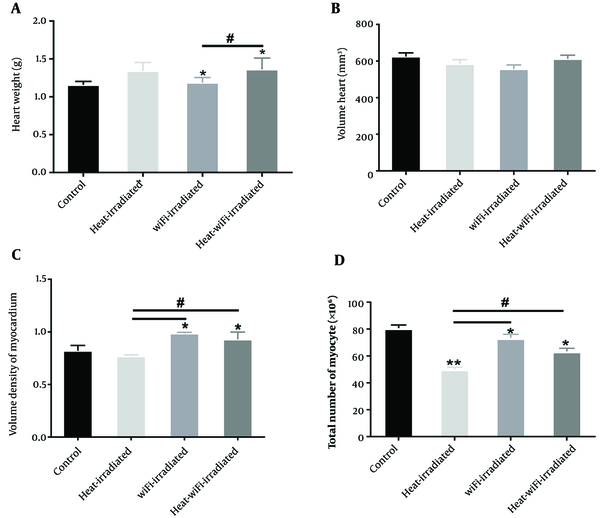
4.2. Heart Volume
There were no significant differences in the heart volume between all the groups (Figure 3B).
4.3. Volume Density of the Myocardium
The volume density of the myocardium increased in the heat+WiFi-irradiated and WiFi-irradiated groups compared to the control group (P < 0.04). The volume density of the myocardium increased in the heat+WiFi-irradiated and WiFi-irradiated groups compared to the heat-irradiated group (P < 0.04) (Figure 3C).
4.4. Cardiomyocytes Nuclei Numbers
The total cardiomyocyte nuclei decreased in the heat+WiFi-irradiated, WiFi-irradiated, and heat-irradiated groups compared to the control group (P < 0.04). The total cardiomyocyte nuclei increased in the heat+WiFi-irradiated and WiFi-irradiated groups compared to the heat-irradiated group (P < 0.03) (Figure 3D).
The results also revealed that the GSH level decreased in the heat+WiFi-irradiated, WiFi-irradiated, and heat-irradiated groups (P < 0.05) (Figure 4A).
Mean ± SEM of the GSH level (A), MDA level (B), and TAC level (C) in the study groups (* and # : P < 0.05).
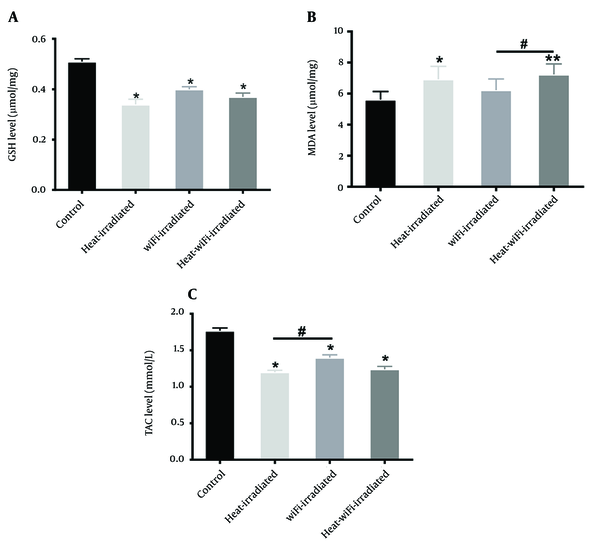
Data analysis showed that MDA levels increased in the heat+WiFi-irradiated and heat-irradiated groups compared to the control group (P < 0.01). Also, the MDA level increased in the heat+WiFi-irradiated-group compared to the WiFi-irradiated group (P < 0.05) (Figure 4B).
The TAC levels decreased in the heat+WiFi irradiated and heat-irradiated groups compared to the control group (P < 0.05). Also, TAC levels increased in the WiFi-exposed group compared to the heat-irradiated group (P < 0.05) (Figure 4C).
5. Discussion
The current study evaluated oxidant-antioxidant status and the structural changes of the heart in WiFi and heat-exposed animal models using experimental and stereological techniques. The results showed that the effects of RFR on oxidant-antioxidant status in rat hearts induced oxidative stress with increased lipid peroxidation and decreased glutathione concentration and total antioxidant capacity. The results showed a statistically significant difference in the levels of GSH and TAC between WiFi -irradiated and heat-WiFi-irradiated groups and the control group. These findings align with the results of Turker et al. (14), showing that antioxidant activity in rat hearts decreased after exposure to radiofrequency radiation. In one study, exposure to 40 Hz for two weeks did not significantly change GSH and TAC. By contrast, two-month exposure to the same frequency decreased GSH and TAC levels. They concluded that the effect of EMF exposure on antioxidant capacity in the heart is related to exposure duration (18).
An increase in the MDA concentration was reported in the heart tissues of male rats exposed to WiFi radiation for one hour for 28 days (14). Previous studies by Devrim et al. (15) and Ozguner et al. (16) reported that lipid peroxidation increased in a 900 MHz exposed group compared to the control group. The inconsistency between our study and other studies could be due to different exposure parameters. The current study showed a significant difference in the MDA level between the heat-irradiated and control groups. Gaitanaki et al. (17) reported that hyperthermia stimulates lipid peroxidation by cellular disorders. Malondialdehyde is a lipid peroxidation product that causes ROS-dependent tissue damage (19).
The heart weight significantly increased in the heat-WiFi-irradiated and WiFi-irradiated groups compared to the control group, suggesting hypertrophic changes. In an exciting study, Mohamed et al. (20) reported that whole heart weight was increased in rats exposed to cell phone EMF, particularly in groups exposed to EMF for longer. The results showed that heart weight did not change significantly in the heat-irradiated group compared to the control group. In another study, heat severely damaged the heart tissue. This study demonstrated that chronic heat stress caused right atrium and ventricular hypertrophy. The reason for these different results might be exposure duration in our study.
The present study showed that the total heart volume did not significantly differ between WiFi-irradiated and control groups. In a stereological study, Monsefi and Khosravanifard (21) reported that short time exposure to low-intensity EMF causes blood supply growth of heart muscle, but animals exposed to the same condition for a longer time adapted to EMF. The last findings of structural changes in the heart determined the volume density of the myocardium and the total number of myocyte nuclei. Previous studies showed an increased percentage of apoptotic cells and congestion of blood vessels without apparent affection of cardiac myocyte, which was inconsistent with our findings (22, 23). Previous studies determined that ROS activates many signaling kinases of hypertrophy and apoptosis factors. Low levels of ROS are essential for kinase regulation signal and protein modulation; however, higher levels of ROS induce apoptosis (23). The study showed a reduction in the total number of myocyte nuclei after exposure to heat. The study by Kwiecien et al. (24) reported the histological alterations in the heart tissue after exposure to 45°C temperature. Lushnikova et al. (25) reported that high temperature decreased the total number of myocytes and concluded that myocyte reduction was probably related to the apoptosis process. The most important things are understanding the risks of using power transmission devices in the workplace and how to deal with them, designing different solutions for the necessary preparations, and maintaining the physical health of people due to injuries with different WiFi frequencies. We suggest conducting necessary studies to reduce the environmental impacts when transmitting the waves of different wavelengths by relevant centers to reduce the potential harm to different age groups in society.
5.1. Conclusions
The current study indicated that exposure to radiofrequency radiation (2.4 GHz) decreased total antioxidant activity in heart tissue with histological changes, including myocardium hypertrophy and decreased number of myocytes.
References
-
1.
Sagar S, Dongus S, Schoeni A, Roser K, Eeftens M, Struchen B, et al. Radiofrequency electromagnetic field exposure in everyday microenvironments in Europe: A systematic literature review. J Expo Sci Environ Epidemiol. 2018;28(2):147-60. [PubMed ID: 28766560]. https://doi.org/10.1038/jes.2017.13.
-
2.
Cheng Y, Kang K. Microwave Biological Effects and Research Progress. International Conference on Materials Science and Biological Engineering. Francis Academic Press; 2017.
-
3.
Saili L, Hanini A, Smirani C, Azzouz I, Azzouz A, Sakly M, et al. Effects of acute exposure to WIFI signals (2.45GHz) on heart variability and blood pressure in Albinos rabbit. Environ Toxicol Pharmacol. 2015;40(2):600-5. [PubMed ID: 26356390]. https://doi.org/10.1016/j.etap.2015.08.015.
-
4.
Shahin S, Singh VP, Shukla RK, Dhawan A, Gangwar RK, Singh SP, et al. 2.45 GHz microwave irradiation-induced oxidative stress affects implantation or pregnancy in mice, Mus musculus. Appl Biochem Biotechnol. 2013;169(5):1727-51. [PubMed ID: 23334843]. https://doi.org/10.1007/s12010-012-0079-9.
-
5.
M Fahmy H, Mohammed FF. Effect of Radiofrequency Waves Emitted From Conventional WIFI Devices on Some Oxidative Stress Parameters in Rat Kidney. J Drug Metab Toxicol. 2015;6(5):1-6. https://doi.org/10.4172/2157-7609.1000195.
-
6.
Ghodbane S, Lahbib A, Ammari M, Sakly M, Abdelmelek H. Does static magnetic field-exposure induced oxidative stress and apoptosis in rat kidney and muscle? Effect of vitamin E and selenium supplementations. Gen Physiol Biophys. 2015;34(1):23-32. [PubMed ID: 25395602]. https://doi.org/10.4149/gpb_2014027.
-
7.
Thielens A, Bell D, Mortimore DB, Greco MK, Martens L, Joseph W. Exposure of Insects to Radio-Frequency Electromagnetic Fields from 2 to 120 GHz. Sci Rep. 2018;8(1):3924. [PubMed ID: 29500425]. [PubMed Central ID: PMC5834628]. https://doi.org/10.1038/s41598-018-22271-3.
-
8.
Aynali G, Naziroglu M, Celik O, Dogan M, Yariktas M, Yasan H. Modulation of wireless (2.45 GHz)-induced oxidative toxicity in laryngotracheal mucosa of rat by melatonin. Eur Arch Otorhinolaryngol. 2013;270(5):1695-700. [PubMed ID: 23479077]. https://doi.org/10.1007/s00405-013-2425-0.
-
9.
Tok L, Naziroglu M, Dogan S, Kahya MC, Tok O. Effects of melatonin on Wi-Fi-induced oxidative stress in lens of rats. Indian J Ophthalmol. 2014;62(1):12-6. [PubMed ID: 24492496]. [PubMed Central ID: PMC3955064]. https://doi.org/10.4103/0301-4738.126166.
-
10.
Cig B, Naziroglu M. Investigation of the effects of distance from sources on apoptosis, oxidative stress and cytosolic calcium accumulation via TRPV1 channels induced by mobile phones and Wi-Fi in breast cancer cells. Biochim Biophys Acta. 2015;1848(10 Pt B):2756-65. [PubMed ID: 25703814]. https://doi.org/10.1016/j.bbamem.2015.02.013.
-
11.
Nickel AG, von Hardenberg A, Hohl M, Loffler JR, Kohlhaas M, Becker J, et al. Reversal of Mitochondrial Transhydrogenase Causes Oxidative Stress in Heart Failure. Cell Metab. 2015;22(3):472-84. [PubMed ID: 26256392]. https://doi.org/10.1016/j.cmet.2015.07.008.
-
12.
Munzel T, Camici GG, Maack C, Bonetti NR, Fuster V, Kovacic JC. Impact of Oxidative Stress on the Heart and Vasculature: Part 2 of a 3-Part Series. J Am Coll Cardiol. 2017;70(2):212-29. [PubMed ID: 28683969]. [PubMed Central ID: PMC5663297]. https://doi.org/10.1016/j.jacc.2017.05.035.
-
13.
Jauchem JR. Exposure to extremely-low-frequency electromagnetic fields and radiofrequency radiation: cardiovascular effects in humans. Int Arch Occup Environ Health. 1997;70(1):9-21. [PubMed ID: 9258703]. https://doi.org/10.1007/s004200050181.
-
14.
Turker Y, Naziroglu M, Gumral N, Celik O, Saygin M, Comlekci S, et al. Selenium and L-carnitine reduce oxidative stress in the heart of rat induced by 2.45-GHz radiation from wireless devices. Biol Trace Elem Res. 2011;143(3):1640-50. [PubMed ID: 21360060]. https://doi.org/10.1007/s12011-011-8994-0.
-
15.
Devrim E, Erguder IB, Kilicoglu B, Yaykasli E, Cetin R, Durak I. Effects of Electromagnetic Radiation Use on Oxidant/Antioxidant Status and DNA Turn-over Enzyme Activities in Erythrocytes and Heart, Kidney, Liver, and Ovary Tissues From Rats: Possible Protective Role of Vitamin C. Toxicol Mech Methods. 2008;18(9):679-83. [PubMed ID: 20020924]. https://doi.org/10.1080/15376510701380182.
-
16.
Ozguner F, Altinbas A, Ozaydin M, Dogan A, Vural H, Kisioglu AN, et al. Mobile phone-induced myocardial oxidative stress: protection by a novel antioxidant agent caffeic acid phenethyl ester. Toxicol Ind Health. 2005;21(9):223-30. [PubMed ID: 16342473]. https://doi.org/10.1191/0748233705th228oa.
-
17.
Gaitanaki C, Mastri M, Aggeli IK, Beis I. Differential roles of p38-MAPK and JNKs in mediating early protection or apoptosis in the hyperthermic perfused amphibian heart. J Exp Biol. 2008;211(Pt 15):2524-32. [PubMed ID: 18626088]. https://doi.org/10.1242/jeb.018960.
-
18.
Goraca A, Ciejka E, Piechota A. Effects of extremely low frequency magnetic field on the parameters of oxidative stress in heart. J Physiol Pharmacol. 2010;61(3):333-8. [PubMed ID: 20610864].
-
19.
Cui J, Sinoway LI. Cardiovascular responses to heat stress in chronic heart failure. Curr Heart Fail Rep. 2014;11(2):139-45. [PubMed ID: 24599558]. [PubMed Central ID: PMC4042428]. https://doi.org/10.1007/s11897-014-0191-y.
-
20.
Mohamed FA, Ahmed AA, El-Kafoury BM, Lasheen NN. Study of the cardiovascular effects of exposure to electromagnetic field. Life Sci J. 2011;8(1):260-74.
-
21.
Monsefi MAZ, Khosravanifard S. [Stereological Study of Heart Volume in Male Rats After Exposure to Electromagnetic Fields]. Iran South Med J. 2008;10(2):112-8. Persian.
-
22.
Simko M, Remondini D, Zeni O, Scarfi MR. Quality Matters: Systematic Analysis of Endpoints Related to "Cellular Life" in Vitro Data of Radiofrequency Electromagnetic Field Exposure. Int J Environ Res Public Health. 2016;13(7). [PubMed ID: 27420084]. [PubMed Central ID: PMC4962242]. https://doi.org/10.3390/ijerph13070701.
-
23.
Ertilav K, Uslusoy F, Ataizi S, Naziroglu M. Long term exposure to cell phone frequencies (900 and 1800 MHz) induces apoptosis, mitochondrial oxidative stress and TRPV1 channel activation in the hippocampus and dorsal root ganglion of rats. Metab Brain Dis. 2018;33(3):753-63. [PubMed ID: 29332300]. https://doi.org/10.1007/s11011-017-0180-4.
-
24.
Kwiecien S, Jasnos K, Magierowski M, Sliwowski Z, Pajdo R, Brzozowski B, et al. Lipid peroxidation, reactive oxygen species and antioxidative factors in the pathogenesis of gastric mucosal lesions and mechanism of protection against oxidative stress - induced gastric injury. J Physiol Pharmacol. 2014;65(5):613-22. [PubMed ID: 25371520].
-
25.
Lushnikova EL, Nepomnyashchikh LM, Molodykh OP, Klinnikova MG. Death, elimination, and regeneration of cardiomyocytes in mice after hyperthermia. Bull Exp Biol Med. 2000;130(8):810-2. [PubMed ID: 11177251]. https://doi.org/10.1007/BF02766102.