Abstract
Background:
Chromium is one of the most commonly used heavy metals in industry. It is known as a pollutant that its discharge into the environment needs special care.Objectives:
The current study aimed to evaluate the functionalized multi-walled carbon nanotubes (f-MWCNTs) as a novel adsorbent of chromium in aqueous solutions.Materials and Methods:
The virgin MWCNT was protonated using sulfuric acid as a strong oxidant. The operating conditions such as initial chromium concentration, contact time, adsorbent dosage and pH were evaluated in the adsorption process. A systematic model for chromium adsorption was presented based on the experimental design. Response surface method (RSM), as a mathematical technique, was used for modeling and optimizing considerable parameters. All experiments were performed according to the Standard Methods for the Examination of Water and WastewaterResults:
According to the statistical analysis, R2 (0.998), adjusted R2 (0.996), prediction R2 (0.906), coefficient of variation (1.38%), and the mean removal efficiency (54.76%) were calculated.Conclusions:
The f-MWCNTs can be applied as an adsorbent with excellent properties in the adsorption process. The Efficiency of 61.75% was predicted by the model in the optimum conditions. In the experimental condition an adsorption percentage of 59.44% was obtained.Keywords
1. Background
Chromium is a heavy metal that is discharged into the environment as industrial wastes, causing environmental problems. Electroplating, fertilizers, pigments, tanning, mining and metallurgyare the main industrial sources of chromium (1). Generally, chromium exists in the environment in two forms: trivalent Cr (III) and hexavalent Cr (VI) (2). According to toxicological studies, Cr (VI) is considered 1000 times more toxic than Cr (III) (3). The Cr (VI) can make some diseases like liver, kidney, lung, and gastrointestinal cancer (4). In aqueous solutions, Cr (VI) is present as dichromate (Cr2O2-7) and chromate (CrO-4), in acidic and alkaline conditions, respectively (5). Chemical precipitation, membrane filtration, liquid extractions, ion exchange, and adsorption are proposed as the available technologies for chromium removal (3, 6, 7). Among the techniques, absorption process is considered more seriously since it is cost-effective in operation and investment, and easy to use. The main problems with the other methods are high costs and complicated operation. Carbon nanotubes (CNTs) are new adsorbents in the carbon family and are intensively studied to remove various pollutants (3, 4, 8-10). Carbon nanotubes are presented in two forms: single-wall and multi-wall depending on the number of their layers (3). The use of multi-walled carbon nanotubes (MWCNTs) as an adsorbent to treat organic and inorganic pollutants such as dyes, phenanthrene, benzene, and heavy metals is studied intensively, and the results indicate that MWCNTs are suitable candidates for the preconcentration and solidification of pollutants from large volumes of wastewater (11). Functionalization of carbon nanotubes is a new approach to improve the applications. Carbon materials are usually functionalized by oxidative processes to change the sidewalls and tube tips (12). Adsorption is one of the applications of functionalized carbon nanotubes. For this purpose, oxidization and carboxylation by acidic and carboxylic groups is common (13).
2. Objectives
One of the techniques to improve the interaction between matrix and pollutants is submitting carbon nanotube to a process called functionalization. Functionalization is a chemical process that inserts functional materials on the sidewall of carbon nanotube. The technique can be utilized to obtain better interaction and dispersion of carbon nanotube into the appropriate matrices. Therefore, the current study aimed to apply f-functionalized-MWCNTs to remove chromium from aqueous solutions. Also, RSM was utilized to optimize the adsorption parameters. To the best of the authors’ knowledge and based on the literature of the studies, there was no previous report on the optimization of chromium adsorption by f-MWCNTs.
3. Materials and Methods
3.1. Materials
H2SO4, 1, 5 diphenylcarbazide, NaOH, HCl, and acetone were purchased from Merck Company (Merck, Germany). An aqueous stock solution of Cr (VI) was prepared using potassium dichromate (K2Cr2O7) in deionized distilled water. Different concentrations of chromium were obtained by diluting the stock solution.
3.2. Functionalization
The MWCNTs with the purity above 95% was prepared from the Iranian Research Institute of Petroleum Industry. The characteristics of MWCNTs are presented in Table 1. The virgin MWCNT was modified by sulfuric acid as source of proton and sulfur agents. In order to functionalize the adsorbent, MWCNT was immersed in sulfuric acid (1 M) for 12 hours. The treated MWCNT was dried at 70°C for six hours (Figure 1).
SEM Micrograph of Functionalized Multi-Walled Carbon Nanotube
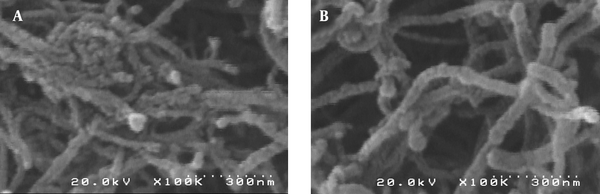
The Characteristics of Virgin Multi-Walled Carbon Nanotube
Characteristic | Value |
---|---|
Specific surface area (BET), m2/g | 270 |
Length, µm | 10-20 |
Diameter, nm | 10-30 |
Thermal conductivity, w/mV | 1500 |
3.3. Analysis
The chromium concentration was measured spectrophotometrically at 540 nm in combination with 1,5- diphenycarbazide [CO(NH.NHC6H5)2] regent using a UV/Vis spectrophotometer (Rayleigh UV 9200, China), according to the standard methods for the analysis of water and wastewater (14). The pH was adjusted to the desired value with 1 M HCl and 1 M NaOH. All experiments were performed in duplicate to minimize errors. To increase the contact between adsorbent and chromium, a rotary shaker at 100 rpm was utilized. The removal percentage of the chromium was calculated using the following Equation 1:

Where C0 (mg/L) is the initial chromium concentration. The experiments were conducted in duplicate and the mean values were considered.
3.4. Response Surface Methodology (RSM)
RSM is includes the collections of the mathematical and statistical methods to model and determine the model equations. First, suitable approximate function between responses and the independent variables were evaluated. This approximate function must be a polynomial of the independent variables. Also the behavior of the system is explained by the following quadratic Equation 2 (15, 16);

Where y: is the response, β0 β1 βii βij: are the regression coefficients, ε: is the error value and Xj: coded variables of the system. In order to estimate the polynomial approximation, the least squares method is used. Central composite design (CCD) was used to fit this model as the most famous design. Table 2 illustrates the CCD for the natural and coded parameters (as; x1: Chromium concentration, x2: initial pH and x3: f-MWCNTs Dosage). In the CCD, low axial and factorial, a central point, high factorial and axial are coded as -2, -1, 0, +1 and +2, respectively. Analysis range start in low axial to high axial and this range for x1, x2 and x3 are considered as; 3-9, 5-20 and 25-75, respectively.
The Natural and Coded Parameters
Factors | Code | Low Axial, (-α = -2) | Low Factorial (-1) | Central (0) | High Factorial (+1) | High Axial (+α = +2) |
---|---|---|---|---|---|---|
pH | x1 | 3 | 4.2 | 6 | 7.8 | 9 |
Chromium, mgL- | x2 | 5 | 8 | 12.5 | 16.8 | 20 |
Dose, mg | x3 | 25 | 35 | 50 | 64 | 75 |
4. Results
Figure 1 shows shows the removal percentage with 5% error during the adsorption of chromium at M- MWCNT. The experiments were conducted at initial concentration of 5-10 mg/L and 0.05 g/100mL of f-MWCNTs dosage during the contact time of four hours. According to the trend of adsorption efficiency in both concentrations of chromium, it can be concluded that maximum removal occurred in 150 minutes and this time for the above experiments were considered as the equivalent time. The removal percentage for 5 and 10 mg/L concentrations were estimated 74% and 55.3%, respectively. The experimental design method was applied 20 runs to optimize the considered parameters. Table 3 displays the run of experiments with the coded factors using the CCD; also adsorption efficiency of various conditions is shown in two categories. The experimental data column was obtained in the designed conditions and the model percentages were calculate based on the provided equation and coded factors (Equation 3). Final equation based on the coded factors was as follows;

The Central Composite Design and Its Responses (Adsorption Efficiency) in the Experimental and Coded Equation Condition (in %)
Run | x1 | x2 | x3 | Experimental Data | Model Data |
---|---|---|---|---|---|
1 | 0 | 2 | 0 | 41.3 | 39.9 |
2 | 0 | 0 | -2 | 35.3 | 31.38 |
3 | -1 | 1 | -1 | 51.1 | 51.09 |
4 | -1 | -1 | -1 | 60.5 | 60.13 |
5 | 0 | 0 | 0 | 55.8 | 54.84 |
6 | -1 | 1 | 1 | 63 | 62.87 |
7 | 0 | -2 | 0 | 75.1 | 80.34 |
8 | 1 | 1 | 1 | 52.2 | 51.33 |
9 | 0 | 0 | 0 | 54.6 | 54.84 |
10 | 2 | 0 | 0 | 34.7 | 30.32 |
11 | 0 | 0 | 0 | 54.5 | 54.84 |
12 | 0 | 0 | 0 | 55 | 54.84 |
13 | -1 | -1 | 1 | 71.1 | 70.87 |
14 | 0 | 0 | 2 | 69.9 | 71.82 |
15 | 1 | -1 | -1 | 48.5 | 47.07 |
16 | 1 | 1 | -1 | 39.9 | 38.07 |
17 | 0 | 0 | 0 | 55.5 | 54.84 |
18 | 0 | 0 | 0 | 54.6 | 54.84 |
19 | 1 | -1 | 1 | 57 | 56.33 |
20 | -2 | 0 | 0 | 71 | 74.32 |
Table 4 indicates ANOVA for the RSM model of chromium adsorption by p-MWCNT. Figure 2, indicates the predicted values versus the actual (experimental) values. Figure 3 displays the contour response surface plot for interaction between initial Cr (VI) concentrations and adsorbent dosage. According to the obvious trend of this diagram, the higher adsorption efficiency occurred in the lower initial concentration and higher f-MWCNTs dosage and vice versa. Interactions between different pH and chromium concentrations are presented in Figure 4, and maximum efficiency is obtained in the lower pH and initial concentrations.
ANOVA of the Response Surface Model
Source | Sum of Squares | Mean Square | F Value | P Value |
---|---|---|---|---|
Quadratic Model | 2563.64 | 213.6367 | 372.7434 | < 0.0001 |
x1 | 684.5 | 684.5 | 1194.284 | < 0.0001 |
x2 | 578 | 578 | 1008.468 | < 0.0001 |
x3 | 578 | 578 | 1008.468 | < 0.0001 |
x1 x2 | 1.125 | 1.125 | 1.962848 | 0.2039 |
x2 x3 | 3.125 | 3.125 | 5.452355 | 0.0500 |
x12 | 5.700947 | 5.700947 | 9.946749 | 0.0161 |
x22 | 24.94254 | 24.94254 | 43.51859 | 0.0003 |
x32 | 9.355946 | 9.355946 | 16.32382 | 0.0049 |
x1 x2 x3 | 1.125 | 1.125 | 1.962848 | 0.2039 |
x12x2 | 128.7493 | 128.7493 | 224.6358 | < 0.0001 |
x12x3 | 66.6043 | 66.6043 | 116.2081 | < 0.0001 |
x1 x22 | 78.75771 | 78.75771 | 137.4128 | < 0.0001 |
Lack of Fit | 868.57 | 173.71 | 2.32 | 0.189 not significant |
Removal Percentage of Chromium
![Removal Percentage of Chromium [conditions: contact time (0-240 min); p-MWCNT dose (50 mg); initial chromium concentrations (5 - 10 mg/L) at pH = 6.6]](http://services.brieflands.com/cdn/serve/313ea/36b8f920dc1fd33dfee816e89d9b7aa2dbc0b316/healthscope-4-1-19892-i001-preview.png)
Contour Diagram of Natural Variables for Cr (VI) Removal Efficiency
![Contour Diagram of Natural Variables for Cr (VI) Removal Efficiency [Conditions: chromium concentration range = 5-20 mg/L, M-MWCNT dosage = 25-75 mg/L, pH 3-9 equivalent time =150 min].](http://services.brieflands.com/cdn/serve/313ea/b8631998329859d623edffd2b832bef849149084/healthscope-4-1-19892-i002-preview.png)
Contour Diagram of Natural Variables for Cr (VI) Removal Efficiency
![Contour Diagram of Natural Variables for Cr (VI) Removal Efficiency [Conditions: chromium concentration range 5-20 mg/L, M-MWCNT dosage = 25-75 mg/L, pH 3-9, equivalent time = 150 min].](http://services.brieflands.com/cdn/serve/313ea/b8bf9cbf7f85e380b2143f6653fe4b9bcb2e9ad8/healthscope-4-1-19892-i003-preview.png)
5. Discussion
5.1. Contact Time
Removal rate of the hexavalent chromium using f-MWCNTs in various conditions is illustrated in Figure 2. According to the obtained results, maximum efficiency occurred in 150 minutes and it was considered as the equivalent time. The equivalent time was assumed equal to the adsorption and desorption rate of hexavalent chromium ions. Adsorption rate occurred faster in the primary times. The maximum absorption was acquired by 90 minutes. With regard to the behavior of f-MWCNTs, it can be evaluated in two stages: (i) the rapid uptake of Cr (VI) ions due to the availability of free sites adsorbent, (ii) decline of the free sites for linkage of Cr (VI) ions with f-MWCNT surface.
5.2. Statistical Analysis
To optimize adsorption, some factors including maximum chromium, pH, and f-MWCNTs dosage were taken into consideration. The optimization defined run is shown in Table 3. Table 4 indicates Analysis of variance (ANOVA) of the RSM model of chromium adsorption by f-MWCNTs. In all the tests, the confidence interval (95% CI) was set at 0.05, resulting in the verification of the accuracy and reliability of the adsorption model. However, a test was performed under optimal factors. Chromium adsorption percentage of about 59.44% was obtained. The significance level was P < 0.0001. The x1x2 and x1x2x3 interaction with 0.2032 had higher P value (> 0.05), which expressed less conformity. The lack of fit of model was not significant (0.05 < 0.189), and the sum of squares, mean squares and F Value for quadratic model were found 2563.64, 213.63 and 372.74, respectively. Statistical values of the quadratic model are shown in Table 4. Response surface based on ANOVA (Table 5) is provided as R2 (0.998), Adjusted R2 (0.996), prediction R2 (0.906), Coefficient of variation (1.38%) and the mean removal efficiency (54.76%). At the end of analysis the optimum conditions are given out by RSM (Table 6). Under optimum conditions, the efficiency of 61.75% was predicted by the model. Figure 3 indicates the predicted values versus the actual (experimental) values. The predicted values were calculated based on the model equation (Equation 3). The respective R² 0.9938 and its fitted equation indicates the reasonability of the experiments.
Statistical Values of the Obtained Removal Data
Standard deviation (Std. ev.) | 0.757065 | R-Squared | 0.998437 |
---|---|---|---|
Mean | 54.763 | Adj R-Squared | 0.995759 |
Coefficient of variance (C.V.) % | 1.382438 | Pred R-Squared | 0.906757 |
PRESS | 239.4146 | Adeq Precision | 67.17292 |
Optimum Values of the Chromium Adsorption Parameter
Cr (IV), mg.L- | Dosage, mg | pH | Cr removal, % | |
---|---|---|---|---|
Model | Experimental | |||
11.5 | 60 | 4.5 | 61.75 | 59.44 |
5.3. Interaction of Cr (VI) Concentration, f-MWCNTs Dosage and pH
Figure 4 indicates the contour response surface plot of the interaction between initial Cr (VI) concentrations and adsorbent dosage. In higher dosage the M-MWCNT dose overall efficiency increased by the same causes as; (i) increasing the available sites on the surfaces, and (ii) increasing the active surface with more oxygen-containing functional groups. Based on the CNTs can be adsorption behavior different sites on a homogeneous bundle such as: interior of the individual tubes, interstitial channels between nanotubes, external groove sites, and the outer surface sites of the individual tubes on the peripheral surface of the bundles (16, 17). Increasing the efficiency at lower concentrations can occurre due to the proper distribution of ions on the CNTs surface and proportion between the amount of chromium ions in the solution and the number of available sites. Interactions between different pH and chromium ions are presented in Figure 5. The maximum efficiency is obtained in lower pH and initial concentrations. Argun et al. reported the chromium adsorption rate as a function of pH, with H2CrO4, HCrO4−, Cr2O72−, CrO42− and Cr3+ ions present as dominant species (18). The role of pH variations and its effects of oxo groups (CxO and CxO2) on carbonaceous material surfaces are expressed by Chaudhary (18). These facts can be used for MWCNT as a carbonaceous material and are shown in Equations 4-6. Also, adsorption decrease at higher pH (pH > 5) are expressed due to the formation of soluble hydroxilated complexes (19).



Predicated Value Versus Actual Value of the Chromium Adsorption Percentage
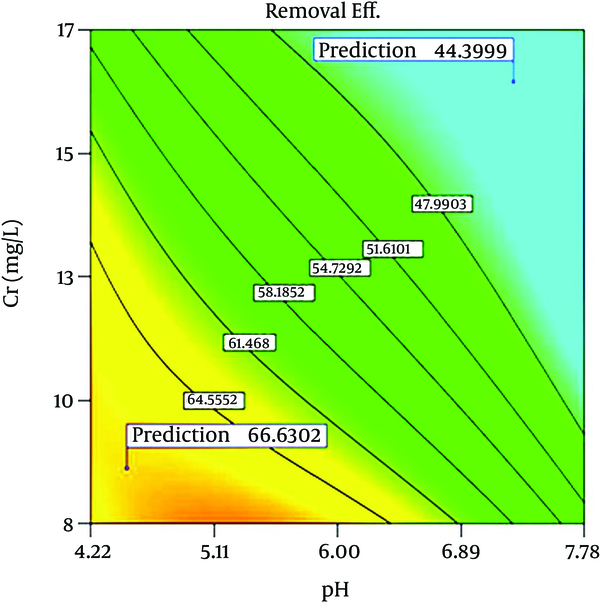
5.4. Sorption Mechanism of Chromium into MWCNTs
In general, the sorption mechanism of surface complexation is significantly affected by pH, whereas the sorption mechanism of ion exchange is influenced by ionic strength. The strong pH dependent and ionic strength independent adsorption of hexavalent chromium to oxidized MWCNTs suggests that the adsorption of hexavalent chromium is mainly dominated by surface complexation rather than ion exchange (3). Figure 6 illustrates the schematic diagram of the oxidization of virgin MWCNTs and the adsorption of hexavalent chromium to oxidized MWCNTs. The functional groups (such as - COOH, - OH2+, - COO−, -OH, -O−, etc.) on the surfaces of oxidized MWCNTs participate in hexavalent chromium adsorption to MWCNTs. Part of the adsorbed hexavalent chromium may be reduced to trivalent chromium on solid surfaces, and are also marked in the diagram. Although the oxygen-containing functional groups are dependent on pH values, there are enough groups to provide adsorption sites for hexavalent chromium uptake from solution to oxidized MWCNTs. It is necessary to note that part of the hexavalent chromium may enter the inner-channel of the oxidized MWCNTs with increasing contact time (20, 21).
Schematic Diagram of the Major Mechanism for the Adsorption of Chromium on Oxidized MWCNT’ Surfaces
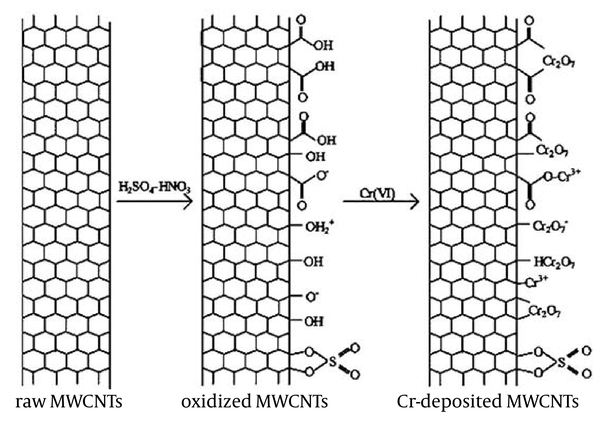
Based on the results of the current study it can be expressed that the f-MWCNTs can be applied as an adsorbent with excellent characteristics in the adsorption process. Based on ANOVA, R2 (0.998), Adjusted R2 (0.996), prediction R2 (0.906), coefficient of variation (1.38%) and the mean removal efficiency (54.76%) were achieved. In the optimum conditions, the efficiency of 61.75% and 59.44% was predicted by the model and the experimental condition.
Acknowledgements
References
-
1.
Kumar R, Bishnoi NR, Bishnoi K. Biosorption of chromium(VI) from aqueous solution and electroplating wastewater using fungal biomass. Chem Eng J. 2008;135(3):202-8. https://doi.org/10.1016/j.cej.2007.03.004.
-
2.
Raji C, Anirudhan TS. Batch Cr(VI) removal by polyacrylamide-grafted sawdust: Kinetics and thermodynamics. Water Res. 1998;32(12):3772-80. https://doi.org/10.1016/s0043-1354(98)00150-x.
-
3.
Hu J, Chen C, Zhu X, Wang X. Removal of chromium from aqueous solution by using oxidized multiwalled carbon nanotubes. J Hazard Mater. 2009;162(2-3):1542-50. [PubMed ID: 18650001]. https://doi.org/10.1016/j.jhazmat.2008.06.058.
-
4.
Tuzen M, Soylak M. Multiwalled carbon nanotubes for speciation of chromium in environmental samples. J Hazard Mater. 2007;147(1-2):219-25. [PubMed ID: 17258392]. https://doi.org/10.1016/j.jhazmat.2006.12.069.
-
5.
Srinath T, Verma T, Ramteke PW, Garg SK. Chromium (VI) biosorption and bioaccumulation by chromate resistant bacteria. Chemosphere. 2002;48(4):427-35. [PubMed ID: 12152745].
-
6.
Di ZC, Ding J, Peng XJ, Li YH, Luan ZK, Liang J. Chromium adsorption by aligned carbon nanotubes supported ceria nanoparticles. Chemosphere. 2006;62(5):861-5. [PubMed ID: 16403560]. https://doi.org/10.1016/j.chemosphere.2004.06.044.
-
7.
Guru M, Venedik D, Murathan A. Removal of trivalent chromium from water using low-cost natural diatomite. J Hazard Mater. 2008;160(2-3):318-23. [PubMed ID: 18417281]. https://doi.org/10.1016/j.jhazmat.2008.03.002.
-
8.
Yang K, Xing B. Adsorption of fulvic acid by carbon nanotubes from water. Environ Pollut. 2009;157(4):1095-100. [PubMed ID: 19084305]. https://doi.org/10.1016/j.envpol.2008.11.007.
-
9.
Wu CH. Adsorption of reactive dye onto carbon nanotubes: equilibrium, kinetics and thermodynamics. J Hazard Mater. 2007;144(1-2):93-100. [PubMed ID: 17081687]. https://doi.org/10.1016/j.jhazmat.2006.09.083.
-
10.
Stafiej A, Pyrzynska K. Adsorption of heavy metal ions with carbon nanotubes. Sep Purif Technol. 2007;58(1):49-52. https://doi.org/10.1016/j.seppur.2007.07.008.
-
11.
Bazrafshan E, Kord Mostafapour F, Faridi H, Mahvi AH, Sargazi S. Removal of 2, 4-dichlorophenoxyacetic acid (2, 4-D) from aqueous environments using single-walled carbon nanotubes. Health Scope. 2013;2(1):39-46.
-
12.
Datsyuk V, Kalyva M, Papagelis K, Parthenios J, Tasis D, Siokou A, et al. Chemical oxidation of multiwalled carbon nanotubes. Carbon. 2008;46(6):833-40. https://doi.org/10.1016/j.carbon.2008.02.012.
-
13.
Arasteh R, Masoumi M, Rashidi AM, Moradi L, Samimi V, Mostafavi ST. Adsorption of 2-nitrophenol by multi-wall carbon nanotubes from aqueous solutions. Appl Sur Sci. 2010;256(14):4447-55. https://doi.org/10.1016/j.apsusc.2010.01.057.
-
14.
APHA AWPCF. Standard Methods for the Examination of Water and Wastewater. 21th ed ed. Washington, DC,USA: American Public Health Association; 2005.
-
15.
Zaroual Z, Chaair H, Essadki AH, El Ass K, Azzi M. Optimizing the removal of trivalent chromium by electrocoagulation using experimental design. Chem Eng J. 2009;148(2-3):488-95. https://doi.org/10.1016/j.cej.2008.09.040.
-
16.
Gholami M, Mirzaei R, Mohammadi R, Zarghampour Z, Afshari A. Destruction of Escherichia coli and Enterococcus faecalis using Low Frequency Ultrasound Technology: A Response Surface Methodology. Health Scope. 2014;3(1). ee14213.
-
17.
Agnihotri S, Mota JP, Rostam-Abadi M, Rood MJ. Structural characterization of single-walled carbon nanotube bundles by experiment and molecular simulation. Langmuir. 2005;21(3):896-904. [PubMed ID: 15667165]. https://doi.org/10.1021/la047662c.
-
18.
Argun ME, Dursun S, Ozdemir C, Karatas M. Heavy metal adsorption by modified oak sawdust: thermodynamics and kinetics. J Hazard Mater. 2007;141(1):77-85. [PubMed ID: 16879919]. https://doi.org/10.1016/j.jhazmat.2006.06.095.
-
19.
Chaudhary AJ, Goswami NC, Grimes SM. Electrolytic removal of hexavalent chromium from aqueous solutions. J Chem Technol Biotechnol. 2003;78(8):877-83. https://doi.org/10.1002/jctb.871.
-
20.
Wang X, Chen C, Hu W, Ding A, Xu D, Zhou X. Sorption of 243Am(III) to multiwall carbon nanotubes. Environ Sci Technol. 2005;39(8):2856-60. [PubMed ID: 15884386].
-
21.
Hummer G, Rasaiah JC, Noworyta JP. Water conduction through the hydrophobic channel of a carbon nanotube. Nature. 2001;414(6860):188-90. [PubMed ID: 11700553]. https://doi.org/10.1038/35102535.