Abstract
Background:
Polycyclic aromatic hydrocarbons (PAHs) are a recalcitrant group of contaminants, known to be highly persistent in the environment. Most of these compounds are persistent pollutants in the soil.Objectives:
This study aimed to investigate the use of Pseudomonas aeruginosa and Sorghum bicolor in improving phytoremediation of soil contaminated with pyrene.Methods:
During 3 months of the experiment, soil samples were collected and analyzed once a month to determine the pyrene removal rate. After the experiment, the plant biomass was measured, and the number of bacteria in the rhizosphere was counted.Results:
The results showed that pyrene removal rate was 35 - 47% in the unplanted treatment. Use of Sorghum bicolor alone reduced soil pyrene by 53 - 70%, whereas the combination of this plant with Pseudomonas bacteria reduced pyrene by 66 - 82%. Moreover, the number of bacteria in the rhizosphere and pyrene removal rate significantly increased in the planted treatments, compared to the unplanted treatment.Conclusions:
Based on the findings, root exudates and Pseudomonas bacteria through stimulation of root development could increase the number of bacteria and enhance the efficiency of pyrene removal. Therefore, combination of phytoremediation with bioremediation can be a suitable alternative for remediation of pyrene-contaminated soils.Keywords
1. Background
Polycyclic aromatic hydrocarbons (PAHs) are a recalcitrant group of contaminants, known to be highly persistent in the environment (1). These compounds constitute a large group of organic molecules with diverse characteristics, such as the number of rings, molecular weight, solubility in water, volatility, and absorption coefficient (2, 3). Pyrene, with 4 benzene rings, is one of these compounds and is considered a persistent contaminant in the environment. It is metabolized to 1-hydroxypyrene by cytochrome P450 isoforms, CYP1A1, CYP1B1, and CYP1A2.
Urinary 1-hydroxypyrene (or its glucuronide conjugate) has been used as a biomarker of exposure to PAHs (4). Although pyrene is not a carcinogen, it is one of 16 polycyclic aromatic pollutants according to the United States environmental protection agency (USEPA) (5). In addition to toxicity, most PAHs in the soil are persistent and can enter the human body through food chains, causing significant risks to human health (6, 7). The USEPA maximum contaminant level (MCL) for pyrene is 0.2 µg.L-1 in water (8). Due to the widespread presence of these compounds in terrestrial ecosystems, several studies have been carried out on remediation of soil contaminated with PAHs (9).
Generally, methods for controlling and removing PAHs from soil include physical remediation, chemical remediation, bioremediation, and phytoremediation (10). Phytoremediation is a green technology, which uses plants for remediation of soil, sediments, and surface water. This technique is highly efficient and more cost-effective than physical and chemical methods of remediation (11). Overall, four major mechanisms are involved in phytoremediation of soil contaminated with PAHs: 1) direct absorption of contaminants by plants, 2) plant volatility and adsorption, 3) plant secretions and enzyme decomposition of PAHs, and 4) degradation of PAHs by microorganisms in the rhizosphere (10).
Although phytoremediation has many advantages over other methods of soil remediation, there are restrictions in the use of this technology in a large scale. Many plant species are sensitive to contaminants, such as PAHs, which can decelerate plant growth and deprive the soil of sufficient biomass for remediation. Therefore, combined use of plants and bacteria for cleaning PAH-contaminated soil can be effective (12, 13). The results of a study by Johnson et al. showed that increased microbial activity in the soil could enhance degradation of PAHs (14).
Use of plants with a fibrous root system can increase soil microbial activities. It has been reported that fibrous roots of grass produce maximum root surface area in the soil, eventually increasing the soil microbial activity (15). Additionally, some soil bacteria, such as plant growth-promoting rhizobacteria (PGPR), have significant positive effects on root development, as well as environmental stress tolerance. They also help plants remove organic and inorganic contaminants from the soil more effectively (16).
Pseudomonas is one of the abundant soil microorganisms, which can biodegrade complex organic compounds in the soil (17). Scarce data are available on combined phytoremediation with Pseudomonas in soil contaminated with pyrene. In this experiment, Sorghum bicolor, a plant of grass family with a fibrous root system, was used to reduce pyrene in the soil. In addition, Pseudomonas aeruginosa bacteria, compatible with petroleum hydrocarbons, were used to enhance pyrene removal from soil.
2. Objectives
This study aimed to evaluate the efficacy of P. aeruginosa and Sorghum bicolor combination in improving phytoremediation of pyrene-contaminated soil.
3. Methods
Pyrene-free soil samples were collected from Shiraz, Iran at a depth of 0 - 20 cm from the ground. Physical and chemical properties of the soil are summarized in Table 1. The particle size distribution (45.5% sand, 37% silt, and 17.5% clay) identified the soil as loam. The air-dried soil samples passed through a 4-mm sieve before use (10). The initial pyrene concentrations in the soil were 150 and 300 mg/kg, respectively. For soil contamination, pyrene was dissolved in acetone and mixed with 10% of the soil (100 g).
Physical and Chemical Properties of the Soil
Characteristics | Quantity |
---|---|
pH | 7.5 |
Electrical conductivity,µs.cm-1 | 225 |
Cation exchange capacity,cmol.kg-1 | 4.83 |
Organic matter content,% | 2 |
Total nitrogen,% | 1.2 |
Potassium ,% | 0.78 |
Phosphorus,mg.kg-1 | 8.2 |
Particle size distribution,% | |
Sand | 45.5 |
Silt | 37 |
Clay | 17.5 |
Acetone was evaporated in a fume hood for 2 days, and soil was mixed with the remaining unpolluted soil (900 g); then, it was sieved twice to ensure the uniform distribution of pyrene. Following that, it was placed under the hood until acetone evaporated completely. Finally, the uncontaminated soil was mixed with contaminated soil before being sieved 3 times for soil homogeneity (18).
Afterwards, 1000 g of soil was poured in each plastic pot, which was then stored in the darkness for 1 week. It should be noted that 3 Sorghum bicolor seeds were planted in each pot. Soil moisture was maintained at 60% water holding capacity. The impact of different parameters, including presence or absence of the plant, various contaminant concentrations, and time, was examined during 90 days of the experiment. It should be mentioned that the experiments were performed in duplicate. Pyrene removal rates were also measured every 30 days. At the end of each 30-day interval, the soil in each pot was homogenized by sifting after harvest.
The treatments in this study included: T blank, pyrene-free soil; P, sorghum plants in pyrene-free soil; T0a, contaminated soil at pyrene concentration of 150 mg.kg-1 under unplanted conditions; T0b, contaminated soil at pyrene concentration of 300 mg.kg-1 under unplanted conditions; T1a, planting sorghum at pyrene concentration of 150 mg.kg-1; T1b, planting sorghum at pyrene concentration of 300 mg.kg-1; T2a, planting sorghum at pyrene concentration of 150 mg.kg-1, along with P. aeruginosa; and T2b, planting sorghum at pyrene concentration of 300 mg.kg-1, along with P. aeruginosa.
After a 90-day period, the plants were harvested. The roots and shoots were separated, washed with tap water, and rinsed with distilled water. To measure dry weight, drying was done in an oven at 105°C for 24 hours (19); the mineral medium is shown in Table 2. The pH of the medium was adjusted to 7, using 2 normal NaOH solutions. A solid mineral medium was prepared by adding 15 g.L-1 of agar and used for separation of pure bacteria. The target bacteria, detected by polymerase chain reaction (PCR), were identified as P. aeruginosa strains.
Characteristics of the Mineral Medium Used for Isolation of Bacteria
Materials,g.L-1 | Quantity | Trace Elements,mg.L-1 | Quantity |
---|---|---|---|
KH2PO4 | 0.2 | MnCL2.4H2O | 40 |
K2HPO4 | 0.8 | MOO3 | 80 |
CaSO4.2H2O | 0.05 | CaSO4 | 6 |
MgSO4 | 0.5 | ZnSO4 | 60 |
FeSO4.7H2O | 0.09 | H3BO3 | 0.03 |
(NH4)2SO4 | 1 |
In order to calculate the number of bacteria in the soil in different treatments, a sample soil suspension was prepared by dissolution of 2 g of soil in 100 mL of distilled water. The solution was then serially diluted and spread on a nutrient agar medium. The plates were then incubated at 28°C for 3 - 5 days, and the number of colony units was calculated as colony-forming units (CFU) and presented as CFU.g-1 of dry soil (20).
Extraction of pyrene from soil was performed using an ultrasound device, based on the USEPA Extraction Method, 3550B. For this purpose, the soil samples were poured into special tubes, and acetone and hexane were added subsequently at 1:1 ratio. Next, the mixture was centrifuged at 6000 rpm for 10 minutes to separate the supernatant from soil. Finally, the supernatant was filtered by polytetrafluorethylene, and some of the filtrated solution was used for the final analysis (21).
A gas chromatography system (Hewlett Packard 5890A), equipped with a flame ionization detector and an HP-5 capillary column (length, 30 m; internal diameter, 0.32 mm; film thickness, 0.25 µm), was used to measure the pyrene concentration. For separation, the initial oven temperature was set at 60°C (holding for 1 minute), which was increased to 250°C at 15°C min-1 for 4 minutes. Helium was also used as the carrier gas at a flow rate of 1.5 ml.min-1. Moreover, the injector and detector temperatures were set at 250 and 300°C, respectively.
4. Results
4.1. Pyrene Removal from Soil
The percentages of pyrene removal during 3 months are presented in Figure 1. At the end of the third month, the residual pyrene in the planted treatments was significantly lower in comparison to the unplanted treatment (P < 0.05). Therefore, use of sorghum and Pseudomonas bacteria significantly reduced PAHs in the soil. Accordingly, in unplanted T0 treatment, the removal percentages were 47% and 35% at pyrene concentrations of 150 and 300 mg.kg-1, respectively. In T1 treatment, where sorghum was planted, pyrene removal percentages were 70.01% and 52.96%, respectively. Moreover, in T2 where Pseudomonas bacteria and sorghum plants were used in combination, the corresponding percentages increased to 82.7% and 65.97%, respectively.
The removal percentage of pyrene during three 30-days periods at concentrations of 150 mg kg-1 (a) and 300 mg kg-1 (b). Error bars represent the standard deviation. The treatments were unplanted soil (T0), sorghum planted soil (T1), and sorghum planted soil along with application of Pseudomonas bacteria (T2).
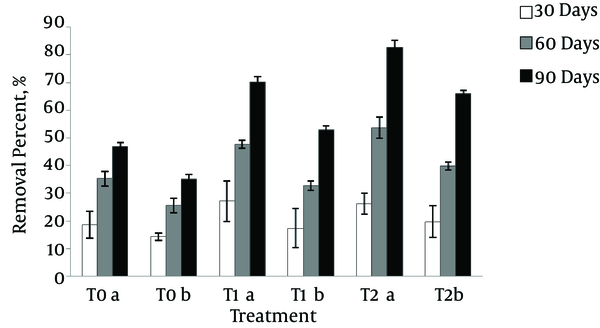
4.2. Plant Biomass
The root and shoot biomass is presented in Figure 2, and the overall height of the plant in different treatments is depicted in Figure 3. Based on the results, at the end of the experiment, sorghum root and shoot biomass increased by 14.5% and 21.27%, respectively at pyrene concentration of 150 mg.kg-1 in planted treatment with sorghum and Pseudomonas, compared to the control treatment. However, root and shoot biomass reduced in sorghum-planted treatment, compared to the control treatment. On the other hand, increasing the concentration to 300 mg.kg-1 reduced the root and shoot biomass in both treatments (P < 0.05).
Sorghum root and shoot biomass after 90 days at two concentrations of (a) 150 mg kg-1 and (b) 300 mg kg-1 .Error bars represent the standard deviation.
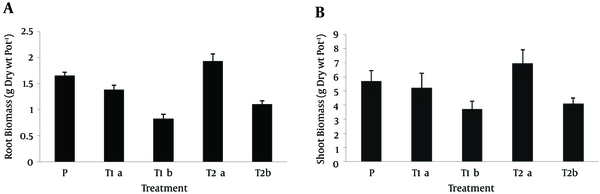
Sorghum height after 90 days at two concentrations of (a) 150 mg kg-1 and (b) 300 mg kg-1 . Error bars represent the standard deviation.
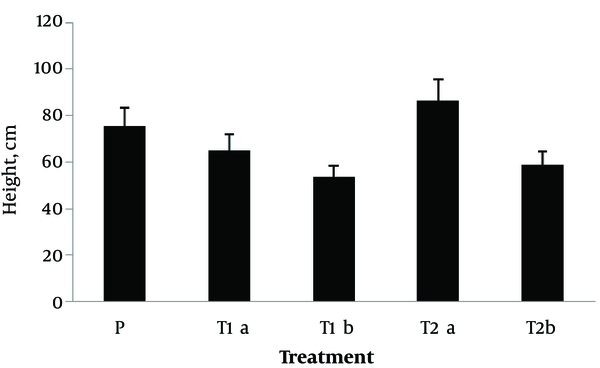
4.3. Soil Microbial Count
Soil microbial count after 3 months (Figure 4) indicated that the number of bacteria increased significantly at both pyrene concentrations in T0-T2 treatments (P < 0.05), compared to the uncontaminated soil samples (T blank). Additionally, the number of bacteria increased significantly at both concentrations in the planted treatments, compared to T0. The number of bacteria ranged from 5.28 to 6.24 × 107 CFU.g-1 of dry soil at concentrations of 150-300 mg.kg-1 in the unplanted soils. The corresponding count ranged from 7.05 to 9.12 × 107 CFU.g-1 of dry soil at 150 mg.kg-1 concentration in T1a and T2a treatments. Besides, the number of soil bacteria ranged from 8.33 to 10.53 × 107 CFU.g-1 of dry soil at pyrene concentration of 300 mg.kg-1 in T1b and T2b treatments.
The number of soil rhizosphere bacteria after 90 days at concentrations of 150 mg kg-1 (a) and 300 mg kg-1 (b) in different treatments: T blank (pyrene-free soil), T0 (unplanted soil), T1 (sorghum planted soil), and T2 (sorghum planted soil along with application of Pseudomonas bacteria). Error bars represent the standard deviation.
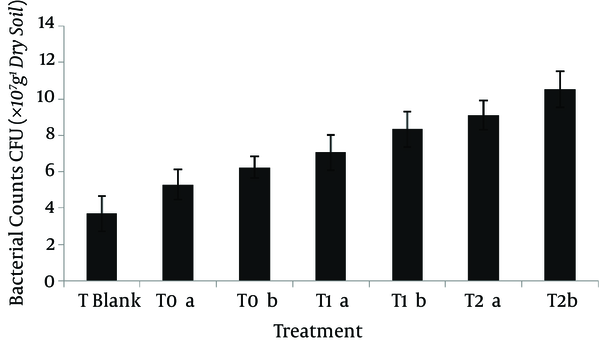
5. Discussion
In the present study, sorghum plant and P. aeruginosa, compatible with petroleum hydrocarbons, were used in combination to improve phytoremediation of soil contaminated with pyrene. In natural PAH-contaminated soils, microbial biomass is not adequate to remove these compounds to an acceptable level (22, 23). The present results confirmed that plants played an important role in pyrene removal from contaminated soil. Accordingly, in T1 treatment (planted treatment), the removal percentages were 70% and 52% for pyrene concentrations of 150 and 300 mg.kg-1, respectively.
Studies performed in this area have also shown that plants increased the removal of PAHs from soil (24). Similarly, Cheema et al. showed that after 65 days of experiment, the initial pyrene (199.3 mg.kg−1) and phenanthrene (200 mg.kg-1) concentrations significantly decreased in the planted soils, as well as unplanted controls; however, a more marked rate of elimination was evident in the presence of plants (25). A mechanism involved in this process is the presence of root exudates, which stimulate microorganisms in PAH dissipation (14, 26). It has been also reported that plant roots cause changes in the soil chemical structure and increase its microbial activities. In other words, increased root biomass increases microbial activity in the soil, which has a positive effect on the reduction of PAH (27-29). According to Figure 4, in the present study, the number of soil bacteria ranged from 6.24 to 9.12 × 107 CFU g-1 of dry soil at 300 mg.kg-1 concentration in T0b and T1b treatments, respectively.
Generally, there are several strategies to increase plant growth and enhance the removal of contaminants from soil. PGPRs, such as Pseudomonas, increase plant growth both indirectly and directly. PGPRs act against phytopathogens and enhance plant growth indirectly. Mechanisms, which may indirectly contribute to plant growth, are antibiotic production, depletion of iron from the rhizosphere, induced systemic resistance, production of fungal cell-wall lytic enzymes, and competition for binding sites on the root (16).
Gao et al. reported no significant effects on the reduction of plant biomass at low PAH concentrations. However, the increase in concentration resulted in an inhibitory effect on plant growth and a decline in biomass (9). Besides, Reilley et al. found that PAH-contaminated soils were incapable of providing the required water and nutrients for plants, causing a reduction in the plant biomass (30).
The increase in microbial activities of planted treatments could be related to factors, such as plant roots and Pseudomonas bacteria. Based on the results, sorghum root biomass increased by 14.5% at pyrene concentration of 150 mg.kg-1 in the planted treatment with sorghum and Pseudomonas bacteria in comparison with the control treatment. Plant roots exudate organic compounds, such as amino acids, organic acids, sugars, and enzymes, providing carbon and energy sources for the growth of rhizosphere microorganisms in the soil (31, 32).
Rhizobacteria with 1-aminocyclopropane-1-carboxylate (ACC) deaminase activity stimulate ACC exudation from plant roots, as a unique source of nitrogen and carbon for the microorganisms. This compound causes the rapid growth of microorganisms with ACC deaminase activity, compared to other microorganisms around the roots. ACC is an immediate precursor of ethylene in plants. On the other hand, ethylene has an inhibitory effect on the lengthening of the root. When plant roots secrete ACC, concentration of the substance inside the plant is reduced. Therefore, less ethylene is produced in the plant roots, thereby stimulating root development (33, 34). It can be stated that interactions between Pseudomonas and plant roots increase the microbial number and stimulate root development.
A previous research indicated that after 12 months of plant growth, the number of bacteria in the planted treatments was 100 folds greater than that of unplanted ones (19). The results of the current study showed that pyrene concentration in soil is another factor, which may increase the number of bacteria in the soil. In the present study, increasing pyrene concentration led to an increase in the number of bacteria in the soil. Similarly, Liste and Prutz suggested that the presence of PAHs in soil could increase the number of soil bacteria, as it provides carbon for the bacteria (35). In addition, the results presented in Table 3 revealed a significant correlation between pyrene removal rate and number of bacteria in the soil at both concentrations. Pyrene removal percentage was also significantly correlated with the root and shoot biomass.
The Correlation between Pyrene Removal Percentage and Various Parameters after 90 Days of Sorghum Growth
Parameters | Pyrene,150 mg.kg-1 | Pyrene,300 mg.kg-1 |
---|---|---|
Bacteria | 0.88 | 0.93 |
Root | 0.85 | 0.92 |
Shoot | 0.87 | 0.95 |
5.1. Conclusion
The findings of this study demonstrated that phytoremediation with Pseudomonas significantly reduced PAHs in the soil. According to the results, sorghum plant alone reduced soil pyrene by 53 - 70%, while use of a combination of sorghum and Pseudomonas reduced pyrene by 66 - 82%. In general, plant roots secrete compounds, which increase decomposition of organic compounds, such as pyrene. Presence of PGPRs, such as Pseudomonas, around the root can also stimulate root development and increase the number of bacteria in the rhizosphere. Furthermore, increased pyrene removal efficiency can be associated with increased root development and rhizosphere bacterial count. Therefore, combination of phytoremediation with bioremediation can be a suitable alternative for remediation of pyrene-contaminated soils.
Acknowledgements
References
-
1.
Huang XD, El-Alawi Y, Penrose DM, Glick BR, Greenberg BM. A multi-process phytoremediation system for removal of polycyclic aromatic hydrocarbons from contaminated soils. Environ Pollut. 2004;130(3):465-76. [PubMed ID: 15182977]. https://doi.org/10.1016/j.envpol.2003.09.031.
-
2.
Mohan SV, Kisa T/, Ohkuma T, Kanaly RA, Shimizu Y. Bioremediation technologies for treatment of PAH-contaminated soil and strategies to enhance process efficiency. Rev. Environ. Sci. Biotechnol. 2006;5(4):347–74.
-
3.
Hoseini M, Yunesian M, Nabizadeh R, Yaghmaeian K, Ahmadkhaniha R, Rastkari N, et al. Characterization and risk assessment of polycyclic aromatic hydrocarbons (PAHs) in urban atmospheric Particulate of Tehran, Iran. Environ Sci Pollut Res Int. 2016;23(2):1820-32. [PubMed ID: 26400241]. https://doi.org/10.1007/s11356-015-5355-0.
-
4.
Yong-Dae K, Todoroki H, Oyama T, Isse T, Matsumoto A, Yamaguchi T, et al. Identification of cytochrome P450 isoforms involved in 1-hydroxylation of pyrene. Environ. Res. 2004;94(3):262-6.
-
5.
Thompson OA, Wolf DC, Mattice JD, Thoma GJ. Influence of nitrogen addition and plant root parameters on phytoremediation of pyrene-contaminated soil. Water Air Soil Pollut. 2008;189(1-4):37–47.
-
6.
Song Q, Li J, Zeng X. Minimizing the increasing solid waste through zero waste strategy. J Clean Prod. 2015;104:199-210.
-
7.
Shabani A, Ghoamalizadeh Ahangar A. Predicting soil sorption coefficients of phenanthrene using a neural network model. Health. Scope. 2016;5(4).
-
8.
Alani R, Olayinka K, Alo B. Studies on persistent organic pollutants (POPs) in the Lagos Lagoon 1: occurrence and levels of polycyclic aromatic hydrocarbons (PAHs) in surface waters of the lagoon. J. Emerg. Trends Eng. Appl. Sci. 2013;4(6):811-8.
-
9.
Gao Y, Zhu L. Plant uptake, accumulation and translocation of phenanthrene and pyrene in soils. Chemosphere. 2004;55(9):1169-78. [PubMed ID: 15081757]. https://doi.org/10.1016/j.chemosphere.2004.01.037.
-
10.
Liu R, Xiao N, Wei S, Zhao L, An J. Rhizosphere effects of PAH-contaminated soil phytoremediation using a special plant named Fire Phoenix. Sci Total Environ. 2014;473-474:350-8. [PubMed ID: 24374595]. https://doi.org/10.1016/j.scitotenv.2013.12.027.
-
11.
Alkorta I, Garbisu C. Phytoremediation of organic contaminants in soils. Bioresour Technol. 2001;79(3):273-6. [PubMed ID: 11499581].
-
12.
Sheng XF, Gong JX. Increased degradation of phenanthrene in soil by Pseudomonas sp. GF3 in the presence of wheat. Soil Biol. Biochem. 2006;38(9):2587-92.
-
13.
Siciliano SD, Fortin N, Mihoc A, Wisse G, Labelle S, Beaumier D, et al. Selection of specific endophytic bacterial genotypes by plants in response to soil contamination. Appl Environ Microbiol. 2001;67(6):2469-75. [PubMed ID: 11375152]. https://doi.org/10.1128/AEM.67.6.2469-2475.2001.
-
14.
Johnson DL, Anderson DR, McGrath SP. Soil microbial response during the phytoremediation of a PAH contaminated soil. Soil Biol. Biochem. 2005;37(12):2334-6.
-
15.
Aprill W, Sims RC. Evaluation of the use of prairie grasses for stimulating polycyclic aromatic hydrocarbon treatment in soil. Chemosphere. 1990;20(1-2):253-65.
-
16.
Glick BR. Using soil bacteria to facilitate phytoremediation. Biotechnol Adv. 2010;28(3):367-74. [PubMed ID: 20149857]. https://doi.org/10.1016/j.biotechadv.2010.02.001.
-
17.
Dehghani M, Taatizadeh SB, Samaei MR. Biodegradation of n-hexadecane in acinetobacter radioresistens liquid culture. Health Scope. 2013. Health. Scope. 2013;2(3):162-7.
-
18.
D'Orazio V, Ghanem A, Senesi N. Phytoremediation of pyrene contaminated soils by different plant species. Soil Air Water. 2013;41(4):377–82.
-
19.
Rostami S, Azhdarpoor A, Rostami M, Samaei MR. The effects of simultaneous application of plant growth regulators and bioaugmentation on improvement of phytoremediation of pyrene contaminated soils. Chemosphere. 2016;161:219-23. [PubMed ID: 27434251]. https://doi.org/10.1016/j.chemosphere.2016.07.026.
-
20.
Cheema SA, Khan MI, Tang X, Zhang C, Shen C, Malik Z, et al. Enhancement of phenanthrene and pyrene degradation in rhizosphere of tall fescue (Festuca arundinacea). J Hazard Mater. 2009;166(2-3):1226-31. [PubMed ID: 19150175]. https://doi.org/10.1016/j.jhazmat.2008.12.027.
-
21.
Jorfi S, Rezaee A, Moheb-Ali GA, Jaafarzadeh NA. Pyrene removal from contaminated soils by modified Fenton oxidation using iron nano particles. J Environ Health Sci Eng. 2013;11(1):17. [PubMed ID: 24499620]. https://doi.org/10.1186/2052-336X-11-17.
-
22.
Tam NF, Wong YS. Effectiveness of bacterial inoculum and mangrove plants on remediation of sediment contaminated with polycyclic aromatic hydrocarbons. Mar Pollut Bull. 2008;57(6-12):716-26. [PubMed ID: 18374368]. https://doi.org/10.1016/j.marpolbul.2008.02.029.
-
23.
Glick BR. Phytoremediation: synergistic use of plants and bacteria to clean up the environment. Biotechnol Adv. 2003;21(5):383-93. [PubMed ID: 14499121].
-
24.
Huesemann MH, Hausmann TS, Fortman TJ, Thom RM, Cullinan V. In situ phytoremediation of PAH- and PCB-contaminated marine sediments with eelgrass (Zostera marina). Ecol. Eng. 2009;35(10):1395-404.
-
25.
Cheema SA, Imran Khan M, Shen C, Tang X, Farooq M, Chen L, et al. Degradation of phenanthrene and pyrene in spiked soils by single and combined plants cultivation. J Hazard Mater. 2010;177(1-3):384-9. [PubMed ID: 20079966]. https://doi.org/10.1016/j.jhazmat.2009.12.044.
-
26.
Muratova A, Golubev S, Wittenmayer L, Dmitrieva T, Bondarenkova A, Hirche F. Effect of the polycyclic aromatic hydrocarbon phenanthrene on root exudation of Sorghum bicolor (L.) Moench. Environ Exp Bot. 2009;66(3):514-21.
-
27.
Kaimi E, Mukaidani T, Miyoshi S, Tamaki M. Ryegrass enhancement of biodegradation in diesel-contaminated soil. Environ Exp Bot. Environ Exp Bot. 2006;55(1):110-9.
-
28.
Xu SY, Chen YX, Wu WX, Wang KX, Lin Q, Liang XQ. Enhanced dissipation of phenanthrene and pyrene in spiked soils by combined plants cultivation. Sci Total Environ. 2006;363(1-3):206-15. [PubMed ID: 15985280]. https://doi.org/10.1016/j.scitotenv.2005.05.030.
-
29.
Binet P, Portal J, Leyval C. Dissipation of 3–6-ring polycyclic aromatic hydrocarbons in the rhizosphere of ryegrass. Soil Biol. Biochem. 2000;32(14):2011-7.
-
30.
Reilley KA, Banks MK, Schwab AP. Dissipation of polycyclic aromatic hydrocarbons in the rhizosphere. J Environ Qual. 1996;25(2):212-9.
-
31.
Rentz JA, Alvarez PJ, Schnoor JL. Benzo[a]pyrene co-metabolism in the presence of plant root extracts and exudates: Implications for phytoremediation. Environ Pollut. 2005;136(3):477-84. [PubMed ID: 15862401]. https://doi.org/10.1016/j.envpol.2004.12.034.
-
32.
Shim H, Chauhan S, Ryoo D, Bowers K, Thomas SM, Canada KA, et al. Rhizosphere competitiveness of trichloroethylene-degrading, poplar-colonizing recombinant bacteria. Appl Environ Microbiol. 2000;66(11):4673-8. [PubMed ID: 11055909].
-
33.
Arshad M, Saleem M, Hussain S. Perspectives of bacterial ACC deaminase in phytoremediation. Trends Biotechnol. 2007;25(8):356-62. [PubMed ID: 17573137]. https://doi.org/10.1016/j.tibtech.2007.05.005.
-
34.
Huang XD, El-Alawi Y, Penrose DM, Glick BR, Greenberg BM. Responses of three grass species to creosote during phytoremediation. Environ Pollut. 2004;130(3):453-63. [PubMed ID: 15182976]. https://doi.org/10.1016/j.envpol.2003.12.018.
-
35.
Liste HH, Prutz I. Plant performance, dioxygenase-expressing rhizosphere bacteria, and biodegradation of weathered hydrocarbons in contaminated soil. Chemosphere. 2006;62(9):1411-20. [PubMed ID: 15996713]. https://doi.org/10.1016/j.chemosphere.2005.05.018.