Abstract
Keywords
Wastewater Treatment Sulfate Radical Diclofenac Advanced Oxidation
1. Background
Pharmaceutical residues are potential pollution of the environment (1, 2) and food chain (3) even at low concentrations. Therefore, they are a great threat to human health. The higher their accumulation in the environment, the higher the threat will be. These compounds are highly resistant to biological degradation processes (4), but even if they be degraded, they still can create adverse effects (5-7).
Diclofenac (DCF), which is a common pain tranquilizer, is one of the most important drug compounds that uses as a non-steroidal anti-inflammatory drug (NSAID) (8). In Table 1 and Figure 1, the physical/chemical properties of DCF are shown. DCF compounds excrete or metabolite into the environment. DCF is an emerging pollutant to the ecosystem (9, 10). Excreted DCF to the environment is a health threat and causes chronic toxic effects (9, 11, 12). Recently, it is reported by environmental quality standards (EQS) that the annual average of DCF in freshwater and marine waters is equal to 0.1 µg.L-1 and 0.01 µg.L-1, respectively (9). Removal of medicine compounds from water resources is of critical importance, and applying control measures is necessary (13, 14). It is widely reported that pharmaceutical residues biodegradation is too slow, and the efficiency of wastewater treatment plants is too low (15, 16). Therefore, in order to complete the purification process, complementary purification methods (e.g., advanced oxidation method, which is used in the current study), are needed.
Physical-Chemical Properties of the Sodium Diclofenac Researched
Diclofenac | |
---|---|
Chemical formula | C14H10Cl2NNaO2 |
Molecular weight, g/mol | 318.13 |
Log Kow | 4.51 |
PKa | 4.3 |
Solubility in water, mg/L (at 25°C) | 2.37 |
Structure of Sodium Diclofenac
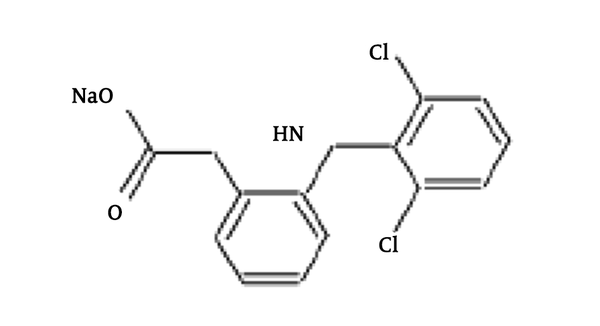
To date, many previous studies are conducted on issues such as advanced oxidation processes (AOPs), ozonation (9), photocatalyst (17, 18), adsorption (19, 20), coagulation-flocculation (21), chlorine dioxide (22), microwave (23), biodegradation (24, 25) and ultrasound radiation (26), which were focused on the removal of DCF from water. Recently, some studies reported that most of the non-biological processes with an emphasis on AOPs have been used for oxidation of pharmaceuticals in water (27). Oxidation is based on the generation of free radicals (•HO, •O2-, •HO2), particularly the hydroxyl radicals, which increase reaction rate constants from 106 to 109 M-1 s-1 (28) and are nonselective (27). Among various oxidation processes, ozone applies alone or in combination with other components (29). Other oxidants, such as ammonium persulfate (PS), are capable of producing the formation of a sulfate radical from sulfate anion (30). Sulfate radical-based oxidation processes are considered because of their high stability, aqueous solubility, relatively low cost, high efficiency, and powerful oxidant destruction of organic contaminants (31). Sulfate radicals’ half-life are longer than OH. Moreover, the redox potential of sulfate radicals are equal to 2.5 - 3.1 V, which is comparable to that of OH (2.8 V); so, sulfate radicals show a high reactivity with a wide range of organic compounds. Another advantage of purification with persulfate is the absence of residue in water (32). According to the reviewed literature, sole ozone was not sufficient for DCF mineralization (28). The results indicate that the simultaneous use of ozone with persulfate is an effective method for the removal of persistent compounds (33). The results of a study conducted by Abu Amr et al. (33) showed that removal rates of COD, NH3-N, and color are 72%, 55%, and 93%, respectively. Meanwhile, Wang et al. (34) reported that when ozone is used solely, the degradation efficiencies of COD, TOC, and color are 41.7%, 8.0%, and 35.0%, respectively. Results of a study conducted by Deming et al. (35) show that the efficiency of COD removal (using O3/Fe+2) is nearly 10% lower than that of O3/Fe+2/PS. As (NH4)2S2O8 is less expensive than sodium persulfate (60%), ultimate oxidation products of (NH4)2S2O8 can be used as agricultural fertilizers. Thus, (NH4)2S2O8 can be more economical and practical for diclofenac removal (30). Additionally, several methods, such as heat, UV irradiation, gamma radiation, and transition metals, can be used to activate persulfate to form sulfate radicals. Currently, •SO4- is generated via UVC irradiation, which has been widely used for oxidation (36). The process is as follows:
Shi et al. (37) demonstrated that the heat-activated persulfate could effectively remove DCF from aqueous. Also, the UV/PS process can be considered as a highly efficient procedure for several contaminants. Al Hakim et al. (38) showed that the theophylline (TP) could not be efficiently degraded by the use of photolysis phenomenon or UV254 alone, while the use of UV along with PS (UV/PS) (PS = 0.25 mM totally degraded TP = 10 mg.L-1 within 20 min), which follows a pseudo-first order reaction, increased the efficiency of the TP degradation than when UV was used alone.
A study conducted by Amasha et al. (39) revealed that the ketoprofen degradation can be performed using the most common PS activation techniques, including thermal, UV, and chemical activation. The authors concluded that UV/PS systems are the most economical efficient process for the removal of ketoprofen. Furthermore, Lu et al. (40) examined the influence of various parameters (such as different doses of PS and solution pH and natural organic matter (NOM)). They showed that compared to UV irradiation and PS oxidation alone, the UV/PS process can have the highest DCF degradation and is appropriate for removing the toxicity (40).
2. Objectives
Because few studies are conducted on removal of DCF from the aquatic environment using the O3/UV/S2O8 process and because extensive use of DCF in pharmaceutical compounds has resulted in pollution of the water and environment, the current study aims to investigate the potential effectiveness of combinatory use of two oxidants in the removal of DCF from aqueous. Furthermore, variation of oxidant concentration, and DCF removal mechanism are studied by ozone and ammonium persulfate [(NH4)2S2O8] activated UV in a semi-continuous reactor. The mineralization of diclofenac is also investigated.
3. Methods
The current experimental study is carried out at a laboratory scale in the laboratory of Shahid Beheshti University of Medical Sciences.
3.1. Chemicals
Diclofenac (97% purity), Na-salt, and humic acid (HA) were obtained from Sigma-Aldrich and used as received. Ammonium persulfate and acetic acid (100% purity) were from Merck Company. Methanol and water were of HPLC grade. Solutions were prepared using a Millipore system (Direct-Q model).
3.2. Reactor
Ozone was produced in a two-liter reactor, and oxidation occurred after contact with DCF (Figure 2). The gas phase was removed using gas washing bottles filled with a potassium iodide solution. Dosages of ozone were 0.5 and 2 g/h, and for the produced ozone was 1 g/h. In this system, the ozone output from the reactor was trapped in 2 containers containing 200 mL of KI solution and degraded.
The scheme of integrated systems in the O3/UV/S2O8 process
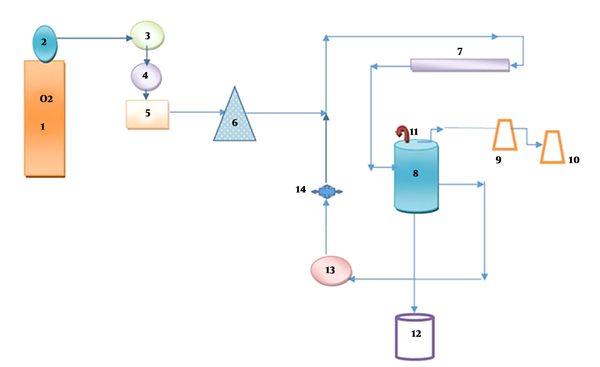
3.2.1. Experimental Set-Up (System Components)
(1) Pure oxygen capsule, (2) manometer, (3) flow meter, (4) silica gel, (5) ozone generator, (6) pitfall trap, (7) UVlamp, (8) Plexiglas’s reactor, (9 and 10) KI solution is two percent, (11) valve air, (12) sampling, (13) centrifugal pump, (14) pressure valve.
3.3. Procedure
All experiments were carried out in a 2-liter lab-scale semi-continuous reactor. (Figure 2) First, a stock solution of 250 cc DCF was prepared by dissolving 2.57 mg DCF in 250 mL deionized water and 1 cc methanol. Solution was prepared at various concentrations of the drug ranged from 5 to 15 mg/L, pH = 3 to 9 in the presence of persulfate at a concentration range of 20 to 220 mg/L at 10 to 40 minutes with ozone content of 1 g/h and for only ozone 0.5 to 2 g/h. The ozone generator device used in the current study can produce up to 20 g/h of ozone. This ozone generator (SS4 model) with a capacity of 20 g/h was obtained from Shamim Sharif Company in Iran. A high-purity oxygen capsule was supplied as an intake gas for ozone production. The amount of ozone produced by the ozone generator was measured and adjusted using the E2350 method of the standard (41).
After titration, the amount of ozone produced was calculated by using the following equation:
A, mL titrant for trap A; B, mL titrant for trap B; N, normality of Na2S2O3; and T, ozonation time, min.
Samples were continuously mixed during the experiments and the remaining concentrations of target and reference compounds were analyzed by HPLC. Experiments were performed with 2 repetitions. A UV lamp was used with 254 nm wavelength and a light intensity of 6 watts. All experiments were performed in 2 replications.
The study began with optimization of pH, then ozone, time, DCF concentration, and persulfate were applied. The study includes optimization of pH, and the effects of other operating parameters including time, ozone concentrations, drug, persulfate, and natural organic matter (humic acid) on the degradation were checked.
3.4. Analytical Procedure
The concentration of DCF was measured by using the HPLC method. Before analysis, filtrations of the solution were done by a Whatman filter with a pore diameter of 0.45 µm. Diclofenac concentrations (5, 8, 10 or 15 mg/L) were detected by employing the HPLC Knauer system. The mobile phase consisted of 30% water HPLC grade with 70% methanol and 1% acetic acid in the case of DCF. A C18 (250 mm × 4.6 mm × 5 µm) column was exerted at a flow-rate of 1 mL/min in the isocratic mode of elution, and a detection wavelength of 276 nm was selected. The oven temperature was 28°C, and the DCF retention time was 15 min. The limits of detection (LODs) and the limits of quantification (LOQs) values were 0.1 mg/mL and 0.06 mg/mL, respectively. Total organic carbon (TOC) was measured using a (TOC-VCSH) TOC analyzer. All experiments were conducted at room temperature.
4. Results and Discussion
The effects of several factors on the DCF degradation are investigated in the current study.
4.1. The Effect of pH
Since the concentration and speciation of reactive radical depend on pH, reaction pH is a critical parameter for DCF degradation (36). As well, pH value of the solution has a substantial effect on ozonation and degradation.
The pH solutions were 3, 5, 6, and 9. The highest percentage of DCF removal was at pH = 6 and 26% to 34% for 10 to 40 minutes, respectively. The higher the pH (pH = 6), the higher would be the amount of •OH formation. As shown in Figure 3A, similarly, Chen et al. (14) reported that TOC removal rates of 23% to 31.6% in sole ozonation increase with pH, which causes the oxidation of generated •OH.
A, Effect of pH (PS = 200 mg/L, DCF = 8 mg/L); B, ozone (pH = 6, DCF = 8 mg/L); C, UV (pH = 6, DCF = 8 mg/L); D, DCF initial concentration (PS = 200 mg/L, pH = 6); E, PS initial concentration; and F, different PS concentration (DCF = 8 mg/L, pH = 6) on the DCF removal.
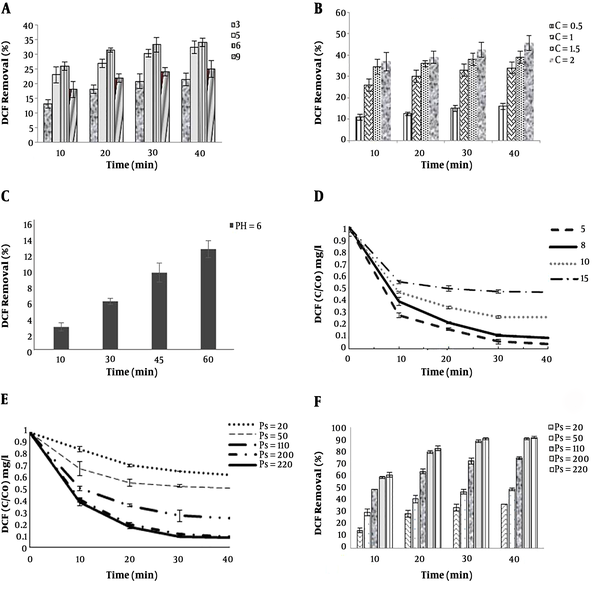
Nevertheless, when pH increase surpassed a certain level, the degradation efficiency decreased. Maybe it can be attributed to the quencher of •OH.
Normally, decomposition of ozone generates HO2, HO3 O3 radicals. As shown in Equations 3 to 6, the reaction of these radicals can generate •OH radicals and increase their concentration. Consequently, •OH reaction with these radicals can result in the cancellation of radical destruction (14).
According to the results, reactiveness of DCF is higher at pH = 6. But it should be noted that when increasing the pH up to 6, excessiveness of scavenger may act as prohibitor (42). A similar finding is reported by Lin et al. (43). Shi et al. (37) revealed that DCF removal at pH < 7 is the main cause of •SO4-. In the current study, the optimal initial pH was 6.0.
4.2. The Effect of Ozone
At the beginning of experiments ozone concentrations were 0.5 to 2 g/h. The amount of ozone from the reactor was measured using potassium iodide and titration. As shown in Figure 3B, when only sole-ozone technique was applied, by increasing the ozone concentration, the DCF degradation rate was also increasing. The removal rates of DCF at concentrations of 0.5 to 2 g/h, at 30 min, were 15% to 42.5%, respectively. However, the efficiency of sole-ozonation in DCF removal was disappointing, and degradation was too low. This result is in line with findings of Naddeo et al. (44). Therefore, ozone resistant byproducts remain in the solution (45). This issue can probably be due to the confined oxidation capacity of ozone as an intermediator, for instance, oxalic acid, acetic acid, and formic acid (16). In the current study, the amount of ozone intake was lower than that of other studies. In optimal conditions (30 min, 8 mg concentration, and inlet ozone dose of 1 gr/h), ozone consumption and efficiency were 72.3 O3mg and 33%, respectively. Because of economic considerations, the time of the reaction and the amount of ozone have been reduced.
4.3. The Effect of UV
The results show that DCF degradation decreases with UV irradiation alone, and the removal of DCF (13% within 60 min) is achieved (Figure 3C). Using UV alone is more likely to eliminate the chemical bond of organic pollutants and lower efficiency in drug degradation; it is better to combine it with other oxidants such as PS (46). Also, intensity of the UV lamps further enhances its efficiency (47). Lu et al. (40) demonstrated 75% DCF removal after 60 min of irradiation by (UV-254 nm and 75 W) activates persulfate (UV/PS); the intensity of the UV lamps in their study was better than that of the present study (6 w).
4.4. The Effect of DCF Dosage
Various initial concentrations and the degradation pattern of DCF are shown in Figure 3D. As shown in the figure, increasing initial DCF concentration from 5 to 15 mg/L has a small effect on DCF removal, so that after 20 minutes, changes in DCF removal are negligible. It has been discussed that the reaction between DCF molecules and reactive species in steady status decreases. In addition, higher concentrations of DCF may suggest a higher scavenging effect, as a result, the concentration of sulfate radical decreases (48). Additionally, maybe it can be attributed to the formation of organic by-products (44). These results are in agreement with that of other studies (44, 48, 49).
4.5. The Effect of PS Dosage and O3/UV/S2O8 Process
As shown in Figure 3, sole O3 or UV can remove DCF (33% removal at 30 min and 13% at 60 min). The O3/UV/S2O8 process was utilized to achieve a higher removal rate. The primary PS concentration in the O3/UV/S2O8 process is significant, which affects the degradation efficiency of intended organic pollutants (36). As shown in the (Figure 3E), increasing time and PS concentration, increases the efficiency of DCF removal (30 min, at concentrations of 20 to 220 mg/L, DCF removal of 34% to 91% and PS = 200 mg/L, reaction periods of 10 to 40, DCF removal of 59% to 91%). The degradation process was carried out for 10 - 40 min. As shown in Figure 3F, the DCF removal efficiency was increased from 10 to 30 and a slight increase can be observed in removal efficiency at 40 min. The best degradation efficiencies were obtained (PS = 200 mg/L) for the reaction period of 30 min.
Based on the O3/UV/S2O8 process, when the PS dosage increased from 200 to 220 mg/L, the removal efficiency rate was insignificant (Figure 3F). Moreover, the effects of excess PS concentration on reducing removal efficiency are examined by several similar studies. Reactions of sulfate radicals, alternatively reaction of the radicals with the contaminant, and high PS concentration would be according to Equation 7. It was due to the generation of less reactive •S2O8- when the •SO4 functions as a scavenger. The process is as follows (7, 50, 51):
Similar results are reported by the Shi et al. (37); their results showed that the elimination of DCF enhances with the increase of initial persulfate dosage. Furthermore, Lu et al. (40) reported 85% removal of DCF in 60 min using the UV/PS process.
4.6. Reaction Kinetic of DCF Removal
As shown in Figures 4A and B, the DCF degradation rates of the first and second orders were k1 = 6.1 × 10-2, R2 = 0.95 and k2 = 3.3 × 10-2, R2 = 0.97, respectively. The correlation coefficient R kinetic equations of the first and second order kinetic equations were close, but k1 was higher than k2. Therefore, the kinetics of the first-order reaction was selected. Similarly, degradation of the ozonation rate was in line with the results of Naddeo et al. study (44). Also, Lu et al. (40) showed that the degradation of DCF by the UV/PS followed the pseudo first order kinetics and R2 = 0.95.
A, First order; and B, second order reaction kinetic of DCF removal, PS = 200 mg/L, pH = 6, DCF = 8 mg/L, O3 = 1 g/h.
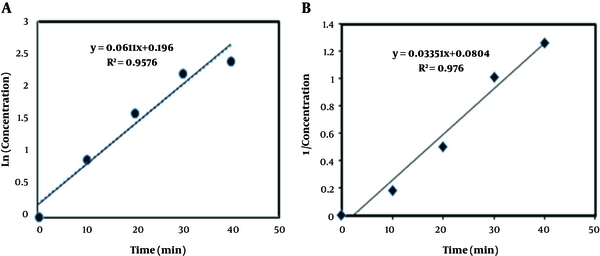
As shown in Figure 4A, the velocity of the DCF reduces the response time function.
4.7. Mineralization of DCF and the Effect of NOM
Another part of the study was the mineralization of DCF by O3/UV/S2O8. Nearly 19% of TOC was removed in 30 min. Therefore, the perfect removal of TOC was not achieved. Considering the reaction rate and amount of mineralization, to achieve higher levels of mineralization the time period should be increased. Also, to get the best results, followed by this reactor, the use of biological reactors for biodegradable organic and inorganic hybrid efficiency is recommended (52). Findings of a study conducted by Lu et al. (40) revealed that, even with 180 min UV/PS treatment, DCF mineralization (32% TOC removal) is limit; the reaction time in their study was much higher than that of the current study.
Humic acid, also, was selected as a scavenging compound in DCF degradation in O3/UV/S2O8 process. When (5 - 20 mg/L) HA was added, the removal rates of DCF diminished from 89% to 87% and 76%, respectively. The negative impacts of HA on the DCF degradation is shown in Figure 5. The removal of DCF was notably inhibited in the presence of HA. Therefore, it can function as a main scavenger of •HO in the reaction system. This issue is addressed by several studies (36, 48, 49, 53). It can be said that the •HA has a strong absorption for UV photons and can reduce the production of •SO4-. Therefore, the reaction between PS and UV photon also decreases (40).
Effect of humic acid on degradation of DCF in the O3/UV/S2O8 process: pH = 6.0, DCF = 8 mg/L, O3 = 1 g/h, PS = 200 mg/L, Time = 10 - 40 min.
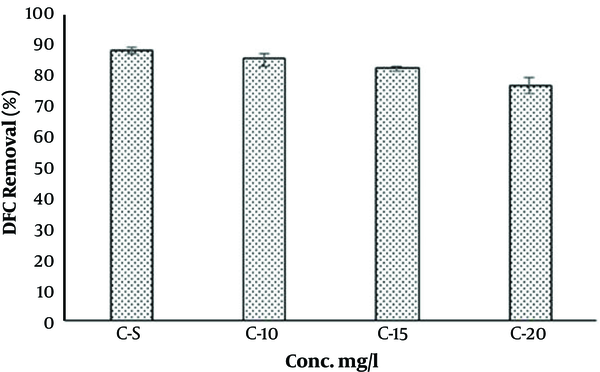
5. Conclusions
Combining O3/UV/S2O8 processes increases the efficiency of DCF removal compared to sole use of these processes. The O3/UV/S2O8 processes can efficiently reduce pharmaceutical compounds; this process enhances the DCF degradation rate through generation of OH and S2O8 radicals.
Initial pH solution, initial DCF and PS and ozone concentration influence the DCF degradation. Sulfate radical-based technologies show promising results for the removal of these particular pharmaceuticals from wastewater treatment plant effluents, mainly because of the higher selectivity of sulfate radical anion over hydroxyl radical, limiting radical scavenging by natural organic matter and allowing for higher abatement and mineralization rates.
References
-
1.
Puckowski A, Mioduszewska K, Łukaszewicz P, Borecka M, Caban M, Maszkowska J, et al. Bioaccumulation and analytics of pharmaceutical residues in the environment: A review. Journal of pharmaceutical and biomedical analysis. 2016;127:232-55. [PubMed ID: 26968887].
-
2.
Sui Q, Cao X, Lu S, Zhao W, Qiu Z, Yu G. Occurrence, sources and fate of pharmaceuticals and personal care products in the groundwater: a review. Emerging Contaminants. 2015;1(1):14-24.
-
3.
Zhang Y, Yin Z, Dai C, Zhou X, Chen W. Interfacial thermodynamics and kinetics of sorption of diclofenac on prepared high performance flower-like MoS 2. Journal of Colloid and Interface Science. 2016;481:210-9. [PubMed ID: 27475708].
-
4.
Xu J, Li Y, Yuan B, Shen C, Fu M, Cui H, et al. Large scale preparation of Cu-doped α-FeOOH nanoflowers and their photo-Fenton-like catalytic degradation of diclofenac sodium. Chemical Engineering Journal. 2016;291:174-83.
-
5.
Chong S, Zhang G, Wei Z, Zhang N, Huang T, Liu Y. Sonocatalytic degradation of diclofenac with FeCeO x particles in water. Ultrasonics Sonochemistry. 2017;34:418-25. [PubMed ID: 27773264].
-
6.
Lu X, Shao Y, Gao N, Chen J, Zhang Y, Wang Q, et al. Adsorption and removal of clofibric acid and diclofenac from water with MIEX resin. Chemosphere. 2016;161:400-11. [PubMed ID: 27448753].
-
7.
de Luna MDG, Budianta W, Rivera KKP, Arazo RO. Removal of sodium diclofenac from aqueous solution by adsorbents derived from cocoa pod husks. Journal of Environmental Chemical Engineering. 2017;5(2):1465-74.
-
8.
Song S, Su Y, Adeleye AS, Zhang Y, Zhou X. Optimal design and characterization of sulfide-modified nanoscale zerovalent iron for diclofenac removal. Applied Catalysis B: Environmental. 2017;201:211-20.
-
9.
Lonappan L, Brar SK, Das RK, Verma M, Surampalli RY. Diclofenac and its transformation products: Environmental occurrence and toxicity-A review. Environment International. 2016;96:127-38. [PubMed ID: 27649472].
-
10.
Espino-Estévez MR, Fernández-Rodríguez C, González-Díaz OM, Araña J, Espinós JP, Ortega-Méndez JA, et al. Effect of TiO 2–Pd and TiO 2–Ag on the photocatalytic oxidation of diclofenac, isoproturon and phenol. Chemical Engineering Journal. 2016;298:82-95.
-
11.
Hua Z, Dai Z, Bai X, Ye Z, Wang P, Gu H, et al. Copper nanoparticles sensitized TiO 2 nanotube arrays electrode with enhanced photoelectrocatalytic activity for diclofenac degradation. Chemical Engineering Journal. 2016;283:514-23.
-
12.
Kummerová M, Zezulka Š, Babula P, Tříska J. Possible ecological risk of two pharmaceuticals diclofenac and paracetamol demonstrated on a model plant Lemna minor. Journal of hazardous materials. 2016;302:351-61. [PubMed ID: 26476323].
-
13.
Lin KA, Yang H, Lee W. Enhanced removal of diclofenac from water using a zeolitic imidazole framework functionalized with cetyltrimethylammonium bromide (CTAB). RSC Advances. 2015;5(99):81330-40.
-
14.
Chen W, Li X, Pan Z, Ma S, Li L. Effective mineralization of Diclofenac by catalytic ozonation using Fe-MCM-41 catalyst. Chemical Engineering Journal. 2016;304:594-601.
-
15.
Ensano BMB, Borea L, Naddeo V, Belgiorno V, de Luna MDG, Ballesteros FC. Removal of Pharmaceuticals from Wastewater by Intermittent Electrocoagulation. Water. 2017;9(2):85.
-
16.
Gao G, Shen J, Chu W, Chen Z, Yuan L. Mechanism of enhanced diclofenac mineralization by catalytic ozonation over iron silicate-loaded pumice. Separation and Purification Technology. 2017;173:55-62.
-
17.
Plakas KV, Sarasidis VC, Patsios SI, Lambropoulou DA, Karabelas AJ. Novel pilot scale continuous photocatalytic membrane reactor for removal of organic micropollutants from water. Chemical Engineering Journal. 2016;304:335-43.
-
18.
Cui Y, Ma Q, Deng X, Meng Q, Cheng X, Xie M, et al. Fabrication of Ag-Ag 2 O/reduced TiO 2 nanophotocatalyst and its enhanced visible light driven photocatalytic performance for degradation of diclofenac solution. Applied Catalysis B: Environmental. 2017.
-
19.
Larous S, Meniai A. Adsorption of Diclofenac from aqueous solution using activated carbon prepared from olive stones. International Journal of Hydrogen Energy. 2016;41(24):10380-90.
-
20.
Bhadra BN, Seo PW, Jhung SH. Adsorption of diclofenac sodium from water using oxidized activated carbon. Chemical Engineering Journal. 2016;301:27-34.
-
21.
Carballa M, Omil F, Lema JM. Removal of cosmetic ingredients and pharmaceuticals in sewage primary treatment. Water Research. 2005;39(19):4790-6. [PubMed ID: 16246396].
-
22.
Wang Y, Liu H, Liu G, Xie Y. Oxidation of diclofenac by aqueous chlorine dioxide: identification of major disinfection byproducts and toxicity evaluation. Science of The Total Environment. 2014;473:437-45. [PubMed ID: 24388821].
-
23.
Das S, Subuddhi U. Studies on the complexation of diclofenac sodium with β–cyclodextrin: Influence of method of preparation. Journal of Molecular Structure. 2015;1099:482-9.
-
24.
Bessa VS, Moreira IS, Tiritan ME, Castro PML. Enrichment of bacterial strains for the biodegradation of diclofenac and carbamazepine from activated sludge. International Biodeterioration & Biodegradation. 2017;120:135-42.
-
25.
Kruglova A, Ahlgren P, Korhonen N, Rantanen P, Mikola A, Vahala R. Biodegradation of ibuprofen, diclofenac and carbamazepine in nitrifying activated sludge under 12 C temperature conditions. Science of the Total Environment. 2014;499:394-401. [PubMed ID: 25215408].
-
26.
Chong S, Zhang G, Zhang N, Liu Y, Zhu J, Huang T, et al. Preparation of FeCeOx by ultrasonic impregnation method for heterogeneous Fenton degradation of diclofenac. Ultrasonics sonochemistry. 2016;32:231-40. [PubMed ID: 27150766].
-
27.
Feng L, van Hullebusch ED, Rodrigo MA, Esposito G, Oturan MA. Removal of residual anti-inflammatory and analgesic pharmaceuticals from aqueous systems by electrochemical advanced oxidation processes. A review. Chemical Engineering Journal. 2013;228:944-64.
-
28.
Rivera-Utrilla J, Sánchez-Polo M, Ferro-García MÁ, Prados-Joya G, Ocampo-Pérez R. Pharmaceuticals as emerging contaminants and their removal from water. A review. Chemosphere. 2013;93(7):1268-87. [PubMed ID: 24025536].
-
29.
Panda KK, Mathews AP. Ozone oxidation kinetics of Reactive Blue 19 anthraquinone dye in a tubular in situ ozone generator and reactor: Modeling and sensitivity analyses. Chemical Engineering Journal. 2014;255:553-67.
-
30.
Liu Y, Wang Q. Removal of elemental mercury from flue gas by thermally activated ammonium persulfate in a bubble column reactor. Environmental science & technology. 2014;48(20):12181-9. [PubMed ID: 25251199].
-
31.
Eslami A, Bahrami H, Asadi A, Alinejad A. Enhanced sonochemical degradation of tetracycline by sulfate radicals. Water Science and Technology. 2016;73(6):1293-300. [PubMed ID: 27003069].
-
32.
Mokhtari SA, Farzadkia M, Esrafili A, Kalantari RR, Jafari AJ, Kermani M, et al. Bisphenol A removal from aqueous solutions using novel UV/persulfate/H 2 O 2/Cu system: optimization and modelling with central composite design and response surface methodology. Journal of Environmental Health Science and Engineering. 2016;14(1):19. [PubMed ID: 27980792].
-
33.
Amr SSA, Aziz HA, Adlan MN, Bashir MJ. Pretreatment of stabilized leachate using ozone/persulfate oxidation process. Chemical engineering journal. 2013;221:492-9.
-
34.
Wang H, Li X, Hao Z, Sun Y, Wang Y, Li W, et al. Transformation of dissolved organic matter in concentrated leachate from nanofiltration during ozone-based oxidation processes (O 3, O 3/H 2 O 2 and O 3/UV). Journal of Environmental Management. 2017;191:244-51. [PubMed ID: 28113066].
-
35.
Deming G, Zequan Z, Moses A, Haikui Z, Jianfeng C, Lei S. Degradation of methyl orange by ozone in the presence of ferrous and persulfate ions in a rotating packed bed. Chemosphere. 2016;146:413-8. [PubMed ID: 26741546].
-
36.
Luo C, Jiang J, Ma J, Pang S, Liu Y, Song Y, et al. Oxidation of the odorous compound 2, 4, 6-trichloroanisole by UV activated persulfate: Kinetics, products, and pathways. Water research. 2016;96:12-21. [PubMed ID: 27016634].
-
37.
Shi H, Zhou G, Liu Y, Fu Y, Wang H, Wu P. Kinetics and pathways of diclofenac degradation by heat-activated persulfate. RSC Advances. 2019;9(54):31370-7.
-
38.
Al Hakim S, Jaber S, Eddine NZ, Baalbaki A, Ghauch A. Degradation of theophylline in a UV254/PS system: Matrix effect and application to a factory effluent. Chemical Engineering Journal. 2020;380:122478.
-
39.
Amasha M, Baalbaki A, Ghauch A. A comparative study of the common persulfate activation techniques for the complete degradation of an NSAID: the case of ketoprofen. Chemical Engineering Journal. 2018;350:395-410.
-
40.
Lu X, Shao Y, Gao N, Chen J, Zhang Y, Xiang H, et al. Degradation of diclofenac by UV-activated persulfate process: Kinetic studies, degradation pathways and toxicity assessments. Ecotoxicology and Environmental Safety. 2017;141:139-47. [PubMed ID: 28340369].
-
41.
Franson MAH. American public health association American water works association water environment federation. Methods. 1995;6(84).
-
42.
Coelho AD, Sans C, Agüera A, Gómez MJ, Esplugas S, Dezotti M. Effects of ozone pre-treatment on diclofenac: intermediates, biodegradability and toxicity assessment. Science of the Total Environment. 2009;407(11):3572-8. [PubMed ID: 19249077].
-
43.
Liu S, Zhao X, Zeng H, Wang Y, Qiao M, Guan W. Enhancement of photoelectrocatalytic degradation of diclofenac with persulfate activated by Cu cathode. Chemical Engineering Journal. 2017;320:168-77.
-
44.
Naddeo V, Belgiorno V, Ricco D, Kassinos D. Degradation of diclofenac during sonolysis, ozonation and their simultaneous application. Ultrasonics sonochemistry. 2009;16(6):790-4. [PubMed ID: 19386533].
-
45.
Aziz KHH, Miessner H, Mueller S, Kalass D, Moeller D, Khorshid I, et al. Degradation of pharmaceutical diclofenac and ibuprofen in aqueous solution, a direct comparison of ozonation, photocatalysis, and non-thermal plasma. Chemical Engineering Journal. 2017;313:1033-41.
-
46.
Tayo LL, Caparanga AR, Doma BT, Liao C. A review on the removal of pharmaceutical and personal care products (PPCPs) using advanced oxidation processes. Journal of Advanced Oxidation Technologies. 2018;21(1):196-214.
-
47.
Hashim N. Visible Light Driven Photocatalysis for Degradation of Diclofenac. 2016.
-
48.
Nasseri S, Mahvi AH, Seyedsalehi M, Yaghmaeian K, Nabizadeh R, Alimohammadi M, et al. Degradation kinetics of tetracycline in aqueous solutions using peroxydisulfate activated by ultrasound irradiation: Effect of radical scavenger and water matrix. Journal of Molecular Liquids. 2017.
-
49.
Li B, Li L, Lin K, Zhang W, Lu S, Luo Q. Removal of 1, 1, 1-trichloroethane from aqueous solution by a sono-activated persulfate process. Ultrasonics sonochemistry. 2013;20(3):855-63. [PubMed ID: 23266439].
-
50.
Eslami A, Asadi A, Meserghani M, Bahrami H. Optimization of sonochemical degradation of amoxicillin by sulfate radicals in aqueous solution using response surface methodology (RSM). Journal of Molecular Liquids. 2016;222:739-44.
-
51.
Hou L, Zhang H, Xue X. Ultrasound enhanced heterogeneous activation of peroxydisulfate by magnetite catalyst for the degradation of tetracycline in water. Separation and Purification Technology. 2012;84:147-52.
-
52.
Aghapour AA, Moussavi G, Yaghmaeian K. Degradation and COD removal of catechol in wastewater using the catalytic ozonation process combined with the cyclic rotating-bed biological reactor. Journal of environmental management. 2015;157:262-6.
-
53.
Liu Y, He X, Fu Y, Dionysiou DD. Degradation kinetics and mechanism of oxytetracycline by hydroxyl radical-based advanced oxidation processes. Chemical Engineering Journal. 2016;284:1317-27. [PubMed ID: 25913467].