Abstract
Background:
Echium amoenum (E. amoenum), as one of the most popular plants in Iran, is traditionally used to treat different types of disorders.Objectives:
This experimental study aimed to evaluate the modulatory effects of E. amoenum on permethrin (PMN)-induced oxidative stress in rats and to determine the cytoprotective effect of E. amoenum on PMN in SK-Hep-1 cells.Methods:
Twenty-four male Wistar rats were randomly divided into four equal groups, including the control (normal saline), orally treated PMN (125 mg/kg of PMN), E. amoenum (100 mg/kg), and E. amoenum + PMN groups for 28 days. The levels of alanine aminotransferase (ALT), alkaline phosphatase (ALP), aspartate aminotransferase (AST), lipid peroxidation (LPO), catalase (CAT), and glutathione peroxidase (GPx), as well as the expression of catalase (CAT) and glutathione peroxidase (GPx), were measured in the liver of all rats. Also, the cytoprotective effect of E. amoenum against PMN was evaluated in the treated SK-Hep-1 cells.Results:
The results indicated that LPO increased significantly in the PMN-treated group, as evidenced by the high concentration of malondialdehyde (MDA) in the liver. Alterations of the antioxidant system were also confirmed by the significant decline in CAT and GPx activities (2.9 ± 0.14 and 0.5 ± 0.03, respectively; P < 0.05) and the significant downregulation of CAT (0.4 ± 0.02 folds) and GPx (0.3 ± 0.01 folds) mRNA expression in the liver (P < 0.05). PMN also stimulated significant changes in hepatic biomarkers and induced pathological changes in the liver. On the other hand, administration of E. amoenum significantly reduced abnormalities in biochemical markers, LPO, antioxidant enzymes, gene expression, and pathological complications induced by PMN (P < 0.05). E. amoenum also exhibited cytoprotective effects against cytotoxicity induced by PMN in SK-Hep-1 cells.Conclusions:
The present results demonstrated that E. amoenum has significant antioxidant, gene-regulating, and cytoprotective effects.Keywords
Permethrin Echium amoenum Oxidative Stress mRNA Expression Liver Rat Cytotoxicity
1. Background
Oxidative stress can be caused by the excess production of reactive oxygen species (ROS) and reactive nitrogen species (RNS). The concentrations of ROS and RNS can be controlled through production and elimination. On the other hand, imbalance between ROS production and antioxidant level can result in oxidative stress. Also, accumulation of ROS and RNS leads to oxidative damage in various molecules, such as proteins, lipids, and nucleic acids. It is known that oxidative stress plays a major role in many important pathophysiological processes (1-3). Pyrethroids are the most popular insecticides, used globally as pest controllers (4). Permethrin (PMN) is the most popular pyrethroid with a wide spectrum of insecticidal activities in agriculture and vector control interventions in public health. It can also control a wide range of veterinary parasites, including flies, ticks, mites, lice, fleas, and mosquitoes (2-4). Recently, the fatal attributes of pesticides have encouraged researchers to study the effects of such agents on oxidative stress and antioxidant defense mechanisms in mammals (3). It is confirmed that pyrethroids can induce toxicity due to the induction of oxidative stress in humans and animals. Previous studies suggest that PMN can induce apoptosis, as well as protein and DNA damage. It has been also shown that oxidative stress may be by far the most essential mechanism of PMN toxicity (5). Therefore, there is a possibility of exposure to PMN in humans and animals.
Today, it is clear that some herbal products can reduce the toxic effects of various toxicants, such as pesticides in mammals (6, 7). These valuable plants exert their protective effects by scavenging free radicals and regulating the antioxidant defense system (7). Some medicinal plants are recognized as sources of natural antioxidants in the biological system. Echium amoenum (E. amoenum) is one of these important medicinal plants in traditional Iranian medicine. All parts of this plant, including the stems, leaves, and flowers, have pharmaceutical properties, except the roots (8, 9). E. amoenum is widely used as a sedative and mood-enhancing agent. It is also applied against cough, sore throat, and pneumonia (10).
The neuroprotective effects of E. amoenum, including anti-ischemic (11), analgesic (8), and anxiolytic effects (12, 13), have been shown in animal models. The bluish-purple flowers of E. amoenum are sources of phenolic compounds, such as rosmarinic acid, cyanidin, and delphinidin (11). Cyanidin-3-glucoside, as the most important anthocyanin in this plant, exhibits protective effects against brain damage and apoptosis, caused by cerebral ischemia (14). To the best of our knowledge, there is no study regarding the effects of E. amoenum on oxidative stress, gene expression, and antioxidant enzymes contributing to oxidative stress in subacute PMN poisoning in animal models.
2. Objectives
In the present study, we aimed to evaluate the effects of E. amoenum on preventing or ameliorating oxidative stress induced by PMN in rats by measuring hepatic catalase (CAT) and glutathione peroxidase (GPx) activities and determining the expression of oxidative stress genes, including CAT and GPx in the rat liver. We also analyzed liver damage by measuring hepatic markers, such as alanine aminotransferase (ALT), alkaline phosphatase (ALP), and aspartate aminotransferase (AST). To confirm liver damage, pathological and cytotoxicity assessments were carried out in SK-Hep-1 cells.
3. Methods
3.1. Animals and Materials
PMN, a pale yellow liquid (CAS No. 52645-53-1; purity ≥ 95%), was purchased from Shanghai Bosman Industrial Co., Ltd. (China). Twenty-four adult male Wistar rats were obtained from Razi Vaccine and Serum Research Institute of Iran. All rats were housed in separate cages and allowed to adapt to the laboratory environment before the experiment. Following seven days of adaptation, the rats were randomly divided into four groups. They were kept under hygienic and standard conditions (temperature, 22 ± 2°C; humidity, 55 ± 5%) in a 12:12 h light/dark cycle, with access to standard laboratory food and water. The animals were maintained according to the guidelines of the Animal Care Committee of Tehran University and the National Institutes of Health (NIH) Guide for Care and Use of Laboratory Animals (NIH publication 86 - 23, revised in 1985).
3.2. E. amoenum Extract Preparation
The petals of E. amoenum were collected from a farm near Qazvin, a city in west of Iran, and the plant samples were identified by a botanist. Extraction was carried out, based on the maceration protocol (15). The plant powder was mixed in double distilled water and ethanol 70% (1:5) for 72 hours. Next, the hydroethanolic extract was filtered, and the filtrate was concentrated via evaporation under vacuum at 40°C. Finally, the extract from the dried flowers of E. amoenum was kept at -20°C until further experiments.
3.3. Groups and Treatments
PMN and E. amoenum were separately suspended in a sterile saline solution container. Twenty-four rats, weighing 200 - 220 g, were randomly divided into four groups of six rats each. The first group (control group) received normal saline (1 mL/kg) for 28 days. The second group was orally administered a single dose of PMN (125 mg/kg, 1/10 LD50) by gavage for 28 days (16). The third group received a single dose of E. amoenum extract (100 mg/kg) orally by gavage for 28 days. Finally, the fourth group received a single dose of E. amoenum extract (100 mg/kg) and a single dose of PMN (125 mg/kg), which were mixed and administered orally by gavage for 28 days. The concentration of E. amoenum extract was selected based on previous studies (17, 18). All animals were observed twice for mortality on a daily basis (for 28 days), and clinical signs, including changes in the skin, fur, eyes, mucous membranes, secretions and excretions, and autonomic activity (e.g., lacrimation, piloerection, pupil size, and unusual respiratory patterns), were investigated. Also, changes in the gait, posture, response to handling, stereotypies (e.g., excessive grooming and repetitive circling), and bizarre behaviors (e.g., self-mutilation and walking backwards) were recorded for 28 days (19). At the end of the experimental period, the rats were anesthetized by intraperitoneal injection of ketamine (30 - 50 mg/kg) and xylazine (3 - 5 mg/kg) for blood withdrawal from the heart. Finally, the rats were sacrificed by cervical dislocation. The liver specimens were quickly excised and washed with cold saline.
3.4. Tissue Preparation
About 500 mg of the liver sample was homogenized in 2 mL of a phosphate-buffered saline (PBS) solution. Then, homogenates were prepared with a homogenizer under ice-cold conditions and centrifuged at 3000 rpm for 15 minutes at 4°C. Next, the produced supernatant was used for measuring the malondialdehyde (MDA) concentration, CAT activity, and GPx activity.
3.5. Analysis of Oxidative Stress and Antioxidant Parameters
The MDA concentration in the liver was assessed, according to a standard method for lipid peroxidation (LPO) analysis (20). Also, GPx and CAT activities were measured, using the methods proposed by Valentine and Aebi, respectively (21, 22).
3.6. Serum Clinical Chemistry Parameters
Blood samples were collected in test tubes, containing EDTA. They were kept for 30 minutes and centrifuged at 3000 rpm for 20 minutes. Next, the serum samples were separated, and the serum concentrations of ALT, AST, and ALP were measured, using an autoanalyzer (BT3000, Italy) and commercial Biosystems kits (Spain), according to the manufacturer’s instructions.
3.7. Histopathological Studies
The liver was isolated and immersed in 10% buffered formalin at room temperature and sectioned transversely into 3 - 4 µm slices. The samples were dehydrated in a graded series of alcohol and xylene and embedded in paraffin. Finally, the sectioned tissues were stained with hematoxylin and eosin (23), and evaluated for morphological and histological changes by light microscopy (Labomed LX-400, USA).
3.8. Total RNA Isolation and cDNA Synthesis
The liver tissue samples were homogenized in phosphate buffer (pH 7.0) at 4°C with a homogenizer, and then, 50 mg of the homogenate was used from each rat to extract total RNA, using the TRI reagentTM solution (Sigma-Aldrich, USA), according to the standard kit protocol. The concentration and purity of RNA were characterized using a NanoDrop ND-1000 spectrophotometer (Thermo Fisher Scientific, Newington, NH, USA). Also, cDNA was synthesized from 1 μg of total RNA, using a cDNA reverse transcription kit (Roche, Basel, Switzerland), according to the manufacturer’s instructions.
3.9. Quantitative Polymerase Chain Reaction (qPCR) for Evaluating Catalase and GPx mRNA Transcription
Real-time PCR amplification was performed, using RevertAid Reverse Transcriptase (Stratagene Mx3000P system, Agilent Technologies, CA, USA) to measure mRNA transcription of target genes (GPx and CAT) in the liver. The sequences of primers were designed, using the GenBank database (Table 1). The PCR reactions were performed under the following conditions: at 95°C for 10 minutes (polymerase activation), followed by 40 cycles of 95°C for 15 seconds (denaturation) and 60°C for one minute (annealing/extension). The results were normalized relative to ß-actin gene expression and reported as fold changes. The relative mRNA expression was calculated, using the Livak method (24).
The Primers Sequences for Real Time PCR (CAT and GPx Genes)
Gene Name | Primer Sequence |
---|---|
GPx | |
Forward | 5’-GTCCACCGTGTATGCCTTCTCC-3’ |
Reverse | 5’-TCTCCTGATGTCCGAACTGATTGC-3’ |
CAT | |
Forward | 5’-GAACGAGGAGGAGAGGAAAC-3’ |
Reverse | 5’-TGAAATTCTTGACCGCTTTC-3’ |
β-actin | |
Forward | 5’-GGTCGGTGTGAACGGATTTGG-3’ |
Reverse | 5’-ATGTAGGCCATGAGGTCCACC-3’ |
3.10. Cell Culture
The SK-Hep-1 cells were purchased from the Cell Bank of Pasteur Institute (Iran). The cell line was cultured in a minimal essential medium (α-MEM medium), supplemented with fetal bovine serum (10%, v/v) and antibiotics (penicillin and streptomycin 1%) at 37°C in a 5% CO2 atmosphere with 95% humidity. The cells were seeded in a flask and examined microscopically. The cell count was monitored with a hemocytometer (Germany).
3.11. Cytotoxic Evaluation
Cell viability was evaluated using the MTT assay. In this colorimetric assay, mitochondrial dehydrogenases convert MTT into formazan crystals in living cells. For cytotoxicity analysis, exponentially growing SK-Hep-1 cells were collected and cultured (1 × 104 cells/well) in a 96-well microtiter plate and then incubated for 24 hours in a 5% CO2 atmosphere before treatment. The cells were treated with agents when their confluency reached up to 70%. A range of PMN and E. amoenum concentrations (10, 100, and 1000 µM) were used to evaluate toxicity, with 0.1% DMSO as the solvent control. Also, the rats were exposed to a range of PMN and E. amoenum concentrations (10, 100, and 1000 µM) simultaneously to evaluate toxicity. After treatment for 24 hours, 100 µL of MTT solution (5 mg/mL) was added to each well and incubated at 37°C for three hours. Next, the supernatants were removed, and 100 mL of DMSO was added to each well to solubilize the formed formazan crystals. Absorbance was read at 570 nm, using a plate spectrophotometer (BioTek, USA).
3.12. Statistical Analysis
Data are presented as mean ± SEM. The mean values of all parameters were compared between the groups, using one-way ANOVA, followed by Tukey’s post-hoc test. Data were analyzed in SPSS version 19, and P < 0.05 was considered statistically significant.
4. Results
4.1. Clinical Signs and Body Weight
There was no mortality in any of the experimental groups. PMN induced clinical signs, including anorexia, depression, mild muscular tremors, head shaking, and mild ataxia in the PMN group. On the other hand, no clinical sign was observed in the E. amoenum group. Also, administration of E. amoenum plus PMN reduced the severity of clinical symptoms mediated by PMN. The mean body weight significantly reduced after the administration of PMN in the second group, compared to the control group. However, there were no significant changes in the mean body weight of the E. amoenum group, compared to the control group. However, administration of E. amoenum plus PMN decreased the body weight, compared to the PMN-treated rats (Table 2).
Groups | Body Weight (g) in the First Day | Body Weight (g) in the Last Day |
---|---|---|
Control | 202 ± 20 | 357 ± 33 |
PMN | 219 ± 22 | 284 ± 27* |
E. amoenum | 210 ± 21 | 361 ± 24 |
PMN + E. amoenum | 209 ± 20 | 310 ± 22** |
4.2. Analysis of Biochemical Markers
There was a significant increase in the mean AST, ALT, and ALP levels (197.6 ± 21.5, 96 ± 8.7, and 679 ± 61.8, respectively) in the PMN group, compared to the control group (P < 0.05). However, regarding the biochemical parameters, no significant changes were induced in the E. amoenum group, compared to the control group. Also, co-administration of E. amoenum plus PMN reduced the AST, ALT, and ALP concentrations (151.3 ± 12.7, 68 ± 6.5, and 531 ± 42.8, respectively) (P < 0.05) (Figure 1).
Effect of PMN, E. amoenum and PMN plus E. amoenum on the ALT, AST, and ALP blood concentrations in rats; Values were given as means ± SEM for six animals in each group. Significantly different from control group (*P < 0.05); significantly different from PMN group (**P < 0.05)
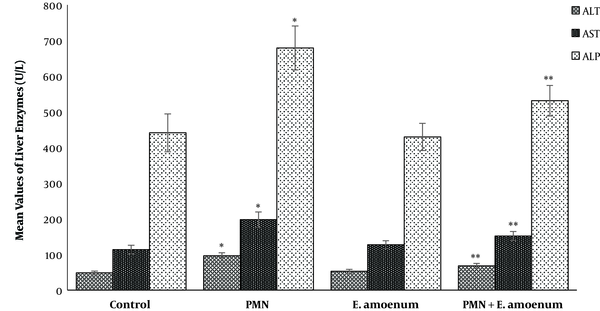
4.3. Analysis of MDA as an LPO Index
A significant increase was observed in the MDA level (98 ± 7.5) following PMN exposure in the PMN group (P < 0.05). On the other hand, simultaneous oral administration of E. amoenum plus PMN significantly reduced the MDA level (72.8 ± 7.5) in the liver tissue, compared to the PMN group (P < 0.05). On the other hand, there were no significant changes in the MDA level of the E. amoenum group, compared to the control group (Figure 2).
Effect of PMN, E. amoenum, and PMN plus E. amoenum on MDA levels of the liver in rats after 4 weeks; values were given as means ± SEM for six animals in each group. Significantly different from control group (*P < 0.05); significantly different from PMN group (**P < 0.05)
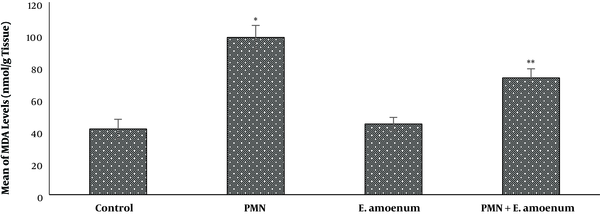
4.4. Antioxidant Enzyme Activities in the Liver
Exposure to PMN induced significant adverse effects on the liver redox status, as evidenced by a significant reduction in CAT (2.9 ± 0.14) and GPx (0.5 ± 0.03) activities, compared to the controls (P < 0.05). Moreover, E. amoenum supplementation with PMN significantly increased the activities of CAT (6.1 ± 0.4) and GPx (0.98 ± 0.06) to a near normal status in the liver (P < 0.05). Also, E. amoenum treatment did not exert any adverse effects on these antioxidant enzymes (Figure 3).
Effect of PMN, E. amoenum, and PMN plus E. amoenum on CAT (A) and GPx (B) activity of the liver in rats after 4 weeks; Values were given as means ± SEM for six animals in each group. Significantly different from control group (*P < 0.05); significantly different from PMN group (**P < 0.05)
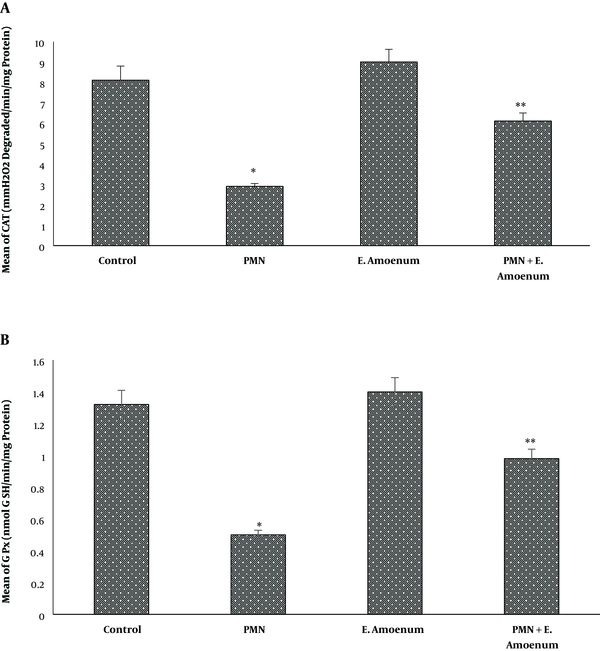
4.5. Histopathology
PMN administration for four weeks induced some pathological complications, such as mononuclear cell infiltration (lymphocytes) and congestion in the sinusoids, central vein, and portal vein in the livers of treated rats. On the other hand, administration of E. amoenum plus PMN could reduce pathological abnormalities, and the liver tissue appeared almost normal. Also, E. amoenum did not induce any pathological abnormalities in the treated animals (Figure 4).
Photomicrographs of the hepatic sections stained with H&E. (A) normal liver (control group), (B) mononuclear cell infiltrates, congestion in sinusoids, central vein and portal vein; (second group), (C) normal liver (third group), (D) mild congestion in central vein with normal hepatocytes (forth group). CV: central vein, arrow: congestion in central vein, circle: mononuclear cell infiltrates, rectangle: congestion in portal vein, squares: mild congestion in central vein. H&E, x 20.
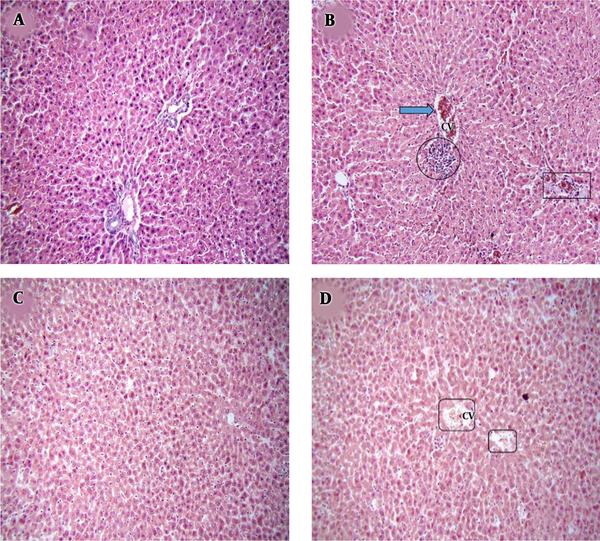
4.6. Expression of GPx and CAT Genes in the Liver
The results of the present study demonstrated that PMN significantly reduced the expression of GPx and CAT (0.3 ± 0.01 and 0.4 ± 0.02 folds, respectively), compared to the control group (0.9 ± 0.05 and 1 ± 0.1 folds, respectively) (P < 0.05). The expression levels of CAT (0.7 ± 0.08 folds) and GPx (0.5 ± 0.06 folds) were significantly higher in the animals treated with a combination of E. amoenum and PMN, compared to the PMN group (P < 0.05). Also, expression of these genes in E. amoenum-treated rats did not change, compared to the control group (Figure 5).
The mRNA expression of CAT (A) and GPx (B) in the liver of Wistar rats after 28 days oral exposure with PMN, E. amoenum and E. amoenum plus PMN. Values were given as means ± SEM for six animals in each group. Significantly different from control group (*P < 0.05); significantly different from PMN group (**P < 0.05)
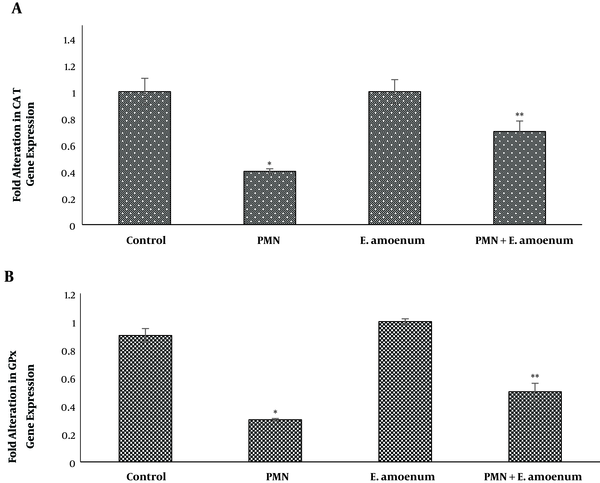
4.7. Cytotoxic Activity
The SK-Hep-1 cells were treated with a range of PMN concentrations (10, 100, and 1000 µM) and E. amoenum for 24 hours. PMN significantly reduced the viability of SK-Hep-1 cells (68 ± 4%) in a dose-dependent manner, starting at 100 µM (P < 0.05). The survival rate of these cells was the lowest (34 ± 2%) at the highest dose (1000 µM). PMN did not induce any significant cytotoxic effects at a concentration of 10 µM for 24 hours (Figure 6). Similarly, E. amoenum did not induce any significant cytotoxic effects at any of the concentrations (Figure 6). In the E. amoenum + PMN group, E. amoenum significantly reduced the cytotoxic effects mediated by PMN (P < 0.05) (Figure 6).
The effect of different doses of PMN (A), E. amoenum (B), and E. amoenum plus PMN (C) on viability of SK-Hep-1 cell lines. Values were given as means ± SEM. Significantly different from control group (*P < 0.05); significantly different from PMN group (**P < 0.05)
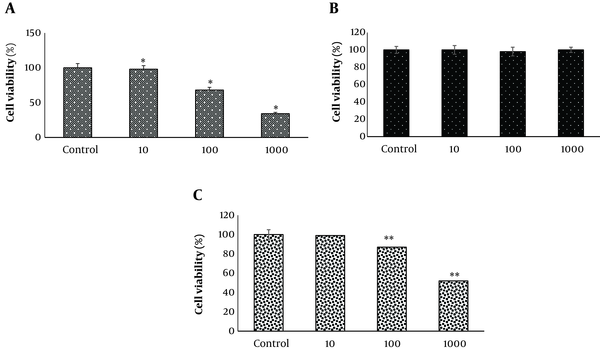
5. Discussion
In the present study, the protective effect of E. amoenum extract on PMN-induced oxidative stress was evaluated in rats. Antioxidant enzymes protect the cells against the toxic effects of ROS through oxidation/reduction processes (25). Our findings revealed that PMN reduced the activities of CAT and GPx in the liver of treated animals. Antioxidant enzyme changes induced by PMN have been shown to cause dose-, time-, tissue-, gender-, and enantioselective-dependent effects (5). In agreement with our results, Gabbianelli et al. reported that GPx and CAT activities decreased following the treatment of rats with PMN (300 mg/kg) for 22 days (26). The reduction in the intracellular concentration of CAT, glutathione S-transferase (GST), glutathione (GSH), and GPx is an indicator of PMN-induced oxidative stress. Consequently, inadequate antioxidant defense or overproduction of free radicals may cause oxidative stress. Our results are compatible with the results of some studies, indicating that PMN reduced the concentrations of GSH, CAT, and GPx in rats (27, 28). In agreement with our findings, previous studies have shown that cypermethrin treatment reduced the GSH content in the liver (29). In the present study, important genes associated with oxidative stress (GPx and CAT) were evaluated. Our results revealed the downregulation of GPx and CAT mRNA expression in rats following PMN exposure. Also, we found that simultaneous treatment with E. amoenum and PMN could ameliorate the downregulation of mRNA transcription. However, we could not find any reports regarding the mRNA transcription of antioxidant enzymes following PMN treatment. In this regard, Badgujar et al. reported that mRNA transcription of GPx and CAT reduced following fipronil treatment in the rat liver, which is similar to our results (30).
Oxidative stress leads to significant LPO in the cell membrane lipids, where LPO can be evaluated by examination of changes in MDA and thiobarbituric acid reactive substances (TBARS). The results of the present study indicated that PMN administration increased the level of MDA in the liver of treated rats. Overall, increased MDA concentration in the liver is an indicator of LPO, induced by this pesticide (26). Gabbianelli et al. reported that when rats were treated with 34.05 mg/kg of PMN, LPO significantly increased (31). Also, treatment with various concentrations of PMN in rats caused an increase in MDA after 45 and 60 days (32). In the present study, we found the potential ameliorating effects of E. amoenum on CAT and GPx activities, which were reduced in the liver of treated animals by PMN. To the best of our knowledge, no study has examined the protective effects of this plant on changes of these antioxidant enzymes. In a previous study, Ranjbar et al. reported that administration of 7 mg/kg of E. amoenum extract for two weeks in humans increased the total antioxidant capacity (TAC) and thiol group molecules, while reducing LPO (33). Also, several studies reported that E. amoenum contains various antioxidants, including flavonoids, tannins, coumarins, xanthenes, and more recently, procyanidins and procyanidins (34, 35). Moreover, Velioglu et al. found a significant correlation between the phenolic content and antioxidant activity (36), whereas another study found no significant relationship (37).
In another study, Rabiei and Setorki showed that pretreatment of scopolamine-exposed rats with E. amoenum extract (50, 75, and 100 mg/kg, IP) daily for 21 days increased TAC and reduced MDA in the brain. They found that E. amoenum has protective effects against scopolamine-induced learning and memory impairments (17). In the present study, the levels of ALT, AST, and ALP significantly increased following the oral administration of PMN in rats. Overall, AST and ALT, in combination with other markers, are essential in the diagnosis of liver disorders. The significant increase of serum ALP level is usually attributed to liver damage (38). Our results are compatible with a previous report, indicating that deltamethrin could induce liver and kidney failure. The findings of these studies reported the increase of biomarkers, including ALT, AST, and lactate dehydrogenase (LDH) following deltamethrin treatment (25 mg/kg body weight) in rats (35). In the present study, E. amoenum ameliorated PMN-induced changes in hepatic parameters. Some studies reported that several species of E. amoenum contained pyrrolizidine alkaloids. In this regard, Mehrabani et al. reported that the total alkaloid content of one E. amoenum species was 0.01% in Iran (39). Based on our findings, this dose of E. amoenum did not induce any hepatic damage in rats. It was also quite efficient in reducing hepatic damage. Moreover, PMN administration caused some pathological complications, including mononuclear cell infiltration and congestion in the sinusoids, central vein, and portal vein in the liver. There is a significant correlation between pathological complications and biochemical changes in the liver, which can indicate PMN hepatotoxicity.
In the present study, PMN reduced the viability of SK-Hep-1 cells in a dose-dependent manner. The survival rate of these cells was the lowest (34%) at the highest dose of PMN (1000 µM). E. amoenum did not induce any significant cytotoxic effects at any of the concentrations. Also, E. amoenum could significantly ameliorate the cytotoxic effects, related to PMN. We can suggest that the antioxidant components of E. amoenum have protective effects against the oxidant activity of PMN in SK-Hep-1 cells. In this regard, Safaeian et al. demonstrated that pretreatment of human umbilical vein endothelial cells (HUVECs) with E. amoenum extract at concentrations of 100 - 1000 μg/mL decreased cell death induced by H2O2 in a dose-dependent manner (11). In another study, the free radical scavenging activity of E. amoenum was evaluated. The results showed that E. amoenum had the highest activity in the hot water extract, while the lowest activity was observed in the acetonic extract (40).
In conclusion, the present results demonstrated that E. amoenum has an ameliorating effect on PMN-induced LPO, oxidative stress, biochemical changes, gene expression, and pathological abnormalities in rats and cytotoxicity in SK-Hep-1 cells. The mechanism of this ameliorating effect may be associated with the free radical scavenging and antioxidant activities. Therefore, E. amoenum can be considered a potential new pharmacological option for ameliorating oxidative stress-induced damages in individuals exposed to pesticides, such as PMN. Meanwhile, further studies are needed to characterize the mechanisms of the protective effects of E. amoenum.
References
-
1.
Lushchak VI. Free radicals, reactive oxygen species, oxidative stress and its classification. Chem Biol Interact. 2014;224:164-75. [PubMed ID: 25452175]. https://doi.org/10.1016/j.cbi.2014.10.016.
-
2.
McCord JM. The evolution of free radicals and oxidative stress. The American Journal of Medicine. 2000;108(8):652-9. https://doi.org/10.1016/s0002-9343(00)00412-5.
-
3.
Li S, Tan HY, Wang N, Zhang ZJ, Lao L, Wong CW, et al. The Role of Oxidative Stress and Antioxidants in Liver Diseases. Int J Mol Sci. 2015;16(11):26087-124. [PubMed ID: 26540040]. [PubMed Central ID: PMC4661801]. https://doi.org/10.3390/ijms161125942.
-
4.
Chrustek A, Holynska-Iwan I, Dziembowska I, Bogusiewicz J, Wroblewski M, Cwynar A, et al. Current Research on the Safety of Pyrethroids Used as Insecticides. Medicina (Kaunas). 2018;54(4). [PubMed ID: 30344292]. [PubMed Central ID: PMC6174339]. https://doi.org/10.3390/medicina54040061.
-
5.
Wang X, Martinez MA, Dai M, Chen D, Ares I, Romero A, et al. Permethrin-induced oxidative stress and toxicity and metabolism. A review. Environ Res. 2016;149:86-104. [PubMed ID: 27183507]. https://doi.org/10.1016/j.envres.2016.05.003.
-
6.
Lushchak VI, Matviishyn TM, Husak VV, Storey JM, Storey KB. Pesticide toxicity: a mechanistic approach. EXCLI J. 2018;17:1101–1136. [PubMed ID: 30564086]. [PubMed Central ID: PMC6295629]. https://doi.org/10.17179/excli2018-1710.
-
7.
Qin S, Huang L, Gong J, Shen S, Huang J, Tang Y, et al. Meta-analysis of randomized controlled trials of 4 weeks or longer suggest that curcumin may afford some protection against oxidative stress. Nutr Res. 2018;60:1-12. [PubMed ID: 30527253]. https://doi.org/10.1016/j.nutres.2018.08.003.
-
8.
Heidari MR, Azad EM, Mehrabani M. Evaluation of the analgesic effect of Echium amoenum Fisch & C.A. Mey. extract in mice: possible mechanism involved. J Ethnopharmacol. 2006;103(3):345-9. [PubMed ID: 16185831]. https://doi.org/10.1016/j.jep.2005.08.027.
-
9.
Assadi SM, Amini HOMAYOUN, Saiiah M, Akhondzadeh S, Kamalinejad M. Efficacy of aqueous extract of Echium amoenum L. in the treatment of mild to moderate major depressive disorder: A randomized double blind clinical trial. Journal of Medicinal Plants. 2004;2(10):61-8.
-
10.
Behnammanesh G, Khalilpour S, Majid ASA, Majid AMSA. Pharmacological actions and potential neuroprotective effects of Rhus coriaria L. And Echium amoenum L.: a brief review. Webmedcentral. 2015;2015:1-8.
-
11.
Safaeian L, Haghjoo Javanmard S, Ghanadian M, Seifabadi S. Cytoprotective and antioxidant effects of Echium amoenum anthocyanin-rich extract in human endothelial cells (HUVECs). Avicenna J Phytomed. 2015;5(2):157-66. [PubMed ID: 25949957]. [PubMed Central ID: PMC4418065].
-
12.
Rabbani M, Sajjadi SE, Vaseghi G, Jafarian A. Anxiolytic effects of Echium amoenum on the elevated plus-maze model of anxiety in mice. Fitoterapia. 2004;75(5):457-64. [PubMed ID: 15261383]. https://doi.org/10.1016/j.fitote.2004.04.004.
-
13.
Sayyah M, Siahpoosh A, Khalili H, Malayeri A, Samaee H. A Double-Blind, Placebo-Controlled Study of the Aqueous Extract of Echium amoenum for Patients with General Anxiety Disorder. Iran J Pharm Res. 2012;11(2):697-701. [PubMed ID: 24250495]. [PubMed Central ID: PMC3832167].
-
14.
Min J, Yu SW, Baek SH, Nair KM, Bae ON, Bhatt A, et al. Neuroprotective effect of cyanidin-3-O-glucoside anthocyanin in mice with focal cerebral ischemia. Neurosci Lett. 2011;500(3):157-61. [PubMed ID: 21651957]. https://doi.org/10.1016/j.neulet.2011.05.048.
-
15.
Handa SS, Khanuja SPS, Longo G, Rakesh DD. Extraction Technologies for Medicinal and Aromatic Plants. 1st ed. Italy: United Nations Industrial Development Organization and the International Centre for Science and High Technology; 2008.
-
16.
Cantalamessa F. Acute toxicity of two pyrethroids, permethrin, and cypermethrin in neonatal and adult rats. Arch Toxicol. 1993;67(7):510-3. [PubMed ID: 8240001]. https://doi.org/10.1007/BF01969923.
-
17.
Rabiei Z, Setorki M. Effect of hydroalcoholic Echium amoenum extract on scopolamine-induced learning and memory impairment in rats. Pharm Biol. 2018;56(1):672-7. [PubMed ID: 31070534]. [PubMed Central ID: PMC6292346]. https://doi.org/10.1080/13880209.2018.1543330.
-
18.
Safaeian L, Tameh AA, Ghannadi A, Naghani EA, Tavazoei H, Alavi SS. Protective effects of Echium amoenum Fisch. and C.A. Mey. against cerebral ischemia in the rats. Adv Biomed Res. 2015;4:107. [PubMed ID: 26261809]. [PubMed Central ID: PMC4513330]. https://doi.org/10.4103/2277-9175.157809.
-
19.
OECD. OECD guidelines for the testing of chemicals. Section. 407: repeated Dose 28-day oral toxicity study in rodents. 2008.
-
20.
Shafiq ur R, Rehman S, Chandra O, Abdulla M. Evaluation of malondialdehyde as an index of lead damage in rat brain homogenates. Biometals. 1995;8(4):275-9. [PubMed ID: 7580048]. https://doi.org/10.1007/BF00141599.
-
21.
Paglia DE, Valentine WN. Studies on the quantitative and qualitative characterization of erythrocyte glutathione peroxidase. J Lab Clin Med. 1967;70(1):158-69. [PubMed ID: 6066618]. https://doi.org/10.5555/uri:pii:0022214367900765.
-
22.
Aebi H. Catalase in vitro. Methods Enzymol. 1984;105:121-6. [PubMed ID: 6727660]. https://doi.org/10.1016/s0076-6879(84)05016-3.
-
23.
Anissian A. Histotechnique. Islamic Azad University Abhar Branch Press; 2005. p. 75-82.
-
24.
Livak KJ, Schmittgen TD. Analysis of relative gene expression data using real-time quantitative PCR and the 2(-Delta Delta C(T)) Method. Methods. 2001;25(4):402-8. [PubMed ID: 11846609]. https://doi.org/10.1006/meth.2001.1262.
-
25.
Yang HY, Lee TH. Antioxidant enzymes as redox-based biomarkers: a brief review. BMB Rep. 2015;48(4):200-8. [PubMed ID: 25560698]. [PubMed Central ID: PMC4436855]. https://doi.org/10.5483/bmbrep.2015.48.4.274.
-
26.
Gabbianelli R, Nasuti C, Falcioni G, Cantalamessa F. Lymphocyte DNA damage in rats exposed to pyrethroids: effect of supplementation with Vitamins E and C. Toxicology. 2004;203(1-3):17-26. [PubMed ID: 15363578]. https://doi.org/10.1016/j.tox.2004.05.012.
-
27.
El-Demerdash FM. Oxidative stress and hepatotoxicity induced by synthetic pyrethroids-organophosphate insecticides mixture in rat. J Environ Sci Health C Environ Carcinog Ecotoxicol Rev. 2011;29(2):145-58. [PubMed ID: 21660820]. https://doi.org/10.1080/10590501.2011.577679.
-
28.
Nasuti C, Falcioni ML, Nwankwo IE, Cantalamessa F, Gabbianelli R. Effect of permethrin plus antioxidants on locomotor activity and striatum in adolescent rats. Toxicology. 2008;251(1-3):45-50. [PubMed ID: 18692543]. https://doi.org/10.1016/j.tox.2008.07.049.
-
29.
Sankar P, Telang AG, Manimaran A. Protective effect of curcumin on cypermethrin-induced oxidative stress in Wistar rats. Exp Toxicol Pathol. 2012;64(5):487-93. [PubMed ID: 21130633]. https://doi.org/10.1016/j.etp.2010.11.003.
-
30.
Badgujar PC, Chandratre GA, Pawar NN, Telang AG, Kurade NP. Fipronil induced oxidative stress involves alterations in SOD1 and catalase gene expression in male mice liver: Protection by vitamins E and C. Environ Toxicol. 2016;31(9):1147-58. [PubMed ID: 25721553]. https://doi.org/10.1002/tox.22125.
-
31.
Gabbianelli R, Palan M, Flis DJ, Fedeli D, Nasuti C, Skarydova L, et al. Imbalance in redox system of rat liver following permethrin treatment in adolescence and neonatal age. Xenobiotica. 2013;43(12):1103-10. [PubMed ID: 23713974]. https://doi.org/10.3109/00498254.2013.796427.
-
32.
Issam C, Zohra H, Monia Z, Hassen BC. Effects of dermal sub-chronic exposure of pubescent male rats to permethrin (PRMT) on the histological structures of genital tract, testosterone and lipoperoxidation. Exp Toxicol Pathol. 2011;63(4):393-400. [PubMed ID: 20381324]. https://doi.org/10.1016/j.etp.2010.02.016.
-
33.
Ranjbar A, Khorami S, Safarabadi M, Shahmoradi A, Malekirad AA, Vakilian K, et al. Antioxidant Activity of Iranian Echium amoenum Fisch & C.A. Mey Flower Decoction in Humans: A cross-sectional Before/After Clinical Trial. Evid Based Complement Alternat Med. 2006;3(4):469-73. [PubMed ID: 17173110]. [PubMed Central ID: PMC1697746]. https://doi.org/10.1093/ecam/nel031.
-
34.
Czinner E, Hagymási K, Blázovics A, Kéry Á, Szőke É, Lemberkovics É. In vitro antioxidant properties of Helichrysum arenarium (L.) Moench. Journal of Ethnopharmacology. 2000;73(3):437-43. https://doi.org/10.1016/s0378-8741(00)00304-4.
-
35.
Mehrabani M, Ghassemi N, Ghannadi ESA, Shams-Ardakani M. Main phenolic compound of petals of Echium amoenum Fisch. and CA Mey., a famous medicinal plant of Iran. DARU Journal of Pharmaceutical Sciences. 2005;13(2):65-9.
-
36.
Velioglu YS, Mazza G, Gao L, Oomah BD. Antioxidant Activity and Total Phenolics in Selected Fruits, Vegetables, and Grain Products. Journal of Agricultural and Food Chemistry. 1998;46(10):4113-7. https://doi.org/10.1021/jf9801973.
-
37.
Kahkonen MP, Hopia AI, Vuorela HJ, Rauha JP, Pihlaja K, Kujala TS, et al. Antioxidant activity of plant extracts containing phenolic compounds. J Agric Food Chem. 1999;47(10):3954-62. [PubMed ID: 10552749]. https://doi.org/10.1021/jf990146l.
-
38.
Mahna D, Puri S, Sharma S. Cypermethrin Induced Liver Toxicity: Altered Gene Expression and DNA Methylation. The FASEB Journal. 2019;33(1_supplement):621.9.
-
39.
Mehrabani M, Ghanadi AR, Sajjadi E, Ghassemi N, SHAMS AM. Toxic pyrrolizidine alkaloids of Echium amoenum Fisch. & Mey. Daru J Pharm Sci. 2006;14(3):122-7.
-
40.
Adel Pilerood S, Prakash J. Evaluation of nutritional composition and antioxidant activity of Borage (Echium amoenum) and Valerian (Valerian officinalis). J Food Sci Technol. 2014;51(5):845-54. [PubMed ID: 24803690]. [PubMed Central ID: PMC4008743]. https://doi.org/10.1007/s13197-011-0573-z.