Abstract
Background:
Cholestasis is described as a disease in which bile flow from the liver is reduced or stopped, and due to its oxidative effects, irreversible consequences may occur.Objectives:
Due to the remarkable antioxidant properties of Oliveria decumbens (OD) and the contribution of oxidants to the progression of bile duct ligation (BDL)-induced cholestasis, this research aimed to examine how the OD ethanolic extract affected liver damage and oxidant-antioxidant balance markers in BDL-induced cholestasis.Methods:
Forty male Wistar rats weighing 200 - 250 g were used. Cholestasis was induced using the BDL approach. The rats were categorized into four groups: Group 1, sham-control (SC); group 2, cholestatic; group 3, SC + OD; and group 4, cholestatic + OD. A dose of OD ethanolic extract was administered orally (500 mg/kg/day) to rats for seven days. Seven days following surgery, the rats’ blood samples were collected; after sacrifice, a part of the liver tissue was isolated. A histopathological examination was performed, while the rest was stored at -70°C in liquid nitrogen. Heparin-containing tubes were used to gather blood samples. In plasma and hepatic tissue, biochemical tests, histopathological evaluations, and oxidative stress markers staining levels were performed.Results:
Our findings showed that OD could effectively reduce liver injury by reducing the activity of liver function enzymes (AST and ALP). At the same time, it did not affect total bilirubin and protein. Bile duct ligation-induced hepatic markers of protein oxidation (PCO) and reactive nitrogen species (NO) were significantly decreased by OD, and it also promoted liver antioxidant capacity by enhancing superoxide dismutase (SOD) activities. Moreover, OD treatment prevented liver bile duct proliferative changes in histopathologic analysis.Conclusions:
Our study confirmed that OD exerts substantial hepatoprotective activities against BDL-induced cholestasis by improving liver damage markers and regulating oxidative stress. It may be a beneficial therapeutic agent for managing cholestasis. Bioassay-guided isolation and identification of bioactive OD secondary metabolites can further direct the discovery of potential natural-based drug candidates.Keywords
Cholestasis Oliveria decumbens Bile Duct Obstruction Stress Oxidative
1. Background
The liver is the principal organ in charge of the selective absorption, metabolism, and excretion of xenobiotics, drugs, and environmental toxins (1). Impaired liver function and defects in the generation, secretion, and flow of bile result in systemic and hepatic accumulation of chemicals in bile, which has damaging and toxic effects on liver cells and other organs in non-physiological concentrations. If this phenomenon persists, it can cause hepatic cholestasis, in which an increase in bile concentration triggers neutrophil accumulation, reactive oxygen species (ROS) generation, tissue inflammation, and the onset of oxidative stress, apoptosis, and necrosis of hepatocellular (2). Cholestasis is a severe liver disease associated with impaired bile formation and its acid accumulation. Intrahepatic cholestasis occurs in liver illnesses like hepatitis, drug-induced liver damage (erythromycin acetate and chlorpromazine), pregnancy, and primary biliary cirrhosis (3). Without efficient therapy, cholestasis can create itching, jaundice, or high blood cholesterol, gradually leading to liver fibrosis, cirrhosis, and even liver failure. Currently, ursodeoxycholic acid (UDCA) is the sole US Food and Drug Administration (FDA)-approved medicine for relieving symptoms and improving abnormal liver enzymes; nevertheless, some individuals show just a partial or no response to the UDCA therapy. Therefore, discovering potential drugs for treating cholestasis is indispensable (4).
Oxidative stress, triggered by free radicals, is one of the critical parameters involved in hepatic cholestasis development and progression. At the same time, the release of pro-inflammatory cytokines from Kupffer cells leads to chronic inflammation (5, 6). Antioxidants, as chemical agents, break down molecular oxygen radicals (pro-oxidants) or neutralize their effects. The enzymatic antioxidants comprising superoxide dismutase (SOD), catalase (CAT), and glutathione peroxidase (GPx) are important for intracellular protection by quickly suppressing ROS like hydrogen peroxide, superoxide, and hydroxyl radicals (7), however, non-enzymatic antioxidants like vitamins E, C (ascorbic acid), and A, flavonoids, albumin, glutathione, thioredoxins, uric acid, polyphenol metabolites, and metal ions chelators including ferritin, transferrin, and ceruloplasmin play also key roles in the defensive mechanism (7).
A non-malignant cell has a proper balance of pro-oxidants and antioxidants; however, raising pro-oxidants or reducing antioxidants generates oxidative stress, and its prolongation destroys biomolecules like proteins, lipids, nucleic acids, and structural carbohydrates (8). ROS can interact with cellular elements like proteins, lipids, and DNA through specific reactions. Among these reactions, lipid peroxidation is the most harmful since the formation of lipid peroxidation products directly promotes the production of free radicals. Lipid peroxidation disrupts membrane integrity, changes fluidity and permeability, alters ion transport and inhibits metabolic processes (9). The oxidation of thiol proteins and the formation of carbonyl proteins, which mainly occur in the amino acids cysteine and methionine, are the most common oxidative changes in proteins. These oxidative stresses can inhibit the substrate’s binding besides reducing the enzymes’ quality and activity (10).
Since the common chemical treatments are long-lasting and have various side effects, today, the tendency to use herbal and traditional medicine has increased. Applying natural and available materials, compatibility of these substances with the body and less damage to the normal tissues and the body cells have encouraged many researchers to study medicinal plants and their therapeutic potencies. The mountain urchin, with the scientific name Oliveria decumbens vent (OD), is an annual plant of the Apiaceae family and grows in southeastern Anatolia, Syria, Iraq, and Iran (11). This plant is robust to adverse weather conditions and has many medicinal, nutritional, and cosmetic potentials (12). Oliveria decumbens is traditionally consumed to cure infections and diseases of the gastrointestinal tract. This plant (often known as "Shavasara" or "Moshk Choopan") grows in restricted parts of Iran’s south and west. It is utilized in traditional or ethnic medicine to cure several disorders, including diarrhea, indigestion, abdominal pain, infections, and fever. Ethnomedicinal reports showed that OD had been utilized as a heart and liver tonic and a central nervous system (CNS) stimulant (11). Its antibacterial, antioxidant, anti-Helicobacter pylori, anti-cholinesterase, anti-tumor, and insecticidal properties have also been demonstrated (12). Additionally, the presence of phenolic compounds has been linked to the plant essential oil’s notable antioxidant and antibacterial activities (11). Studies on the biological functions of OD essential oil have recently been carried out. According to gas chromatographic analysis, thymol, carvacrol, p-cymene, and γ-terpinene are the main compounds that are abundant in essential oils (11). The remedial properties of this plant can chiefly be ascribed to its volatile components, such as carvacrol and thymol. Thymol and carvacrol exhibit a wide spectrum of biological effects such as antibacterial, anti-Helicobacter pylori, and cytotoxic activities (12, 13).
Jamali et al. identified the mechanism of apoptosis related to the cytotoxicity of OD and introduced it as an option for cancer treatment (14). Recently, the incidence of opportunistic fungal infections such as candidiasis has significantly increased due to immunocompromised conditions. This nosocomial infection is caused by different Candida species, where C. albicans is the main disease agent and shows varied antifungal susceptibility (15).
A validated animal model based on the induction of extrahepatic cholestasis in mice using bile duct ligation (BDL) has been proposed to evaluate the hepatic cholestasis potency (16). This model induces bile duct-directed inflammatory response, which results in bile duct damage linked to biliary proliferation, eventually leading to liver fibrosis and cirrhosis. Cholestasis and obstructive jaundice occur approximately seven days after BDL, which can be used in therapeutic studies for the protective effect against cholestasis (17).
2. Objectives
The current study was planned to assess the potency of the OD ethanolic extract on liver damage and oxidant-antioxidant balance markers in BDL-induced cholestasis in a rat model.
3. Methods
3.1. Plant Extract Preparation
The OD flowers were gathered from the Sisakht region (next to Yasuj) in spring, then cleaned and dried in a dark room. The dried plant material was subsequently crushed, and 100 g was extracted through 1 L of ethanol (70%) using the maceration method at 37°C for 48 h. The filtrate was concentrated utilization of a rotary apparatus under vacuum conditions. The extract was then dried in an incubator at 50°C and kept at -20°C until further experiments (18).
3.2. Study Design and Target Groups
In the current study, 40 male Wistar rats (three months old) weighing 200 - 250 g were used. The study followed the ARRIVE guidelines, and all protocols were done under the Yasuj University of Medical Sciences Ethics Committee (Ethical code: IR.YUMS.REC.1400.046). In order to cause cholestasis in 20 rats, the BDL method was used. After producing general anesthesia with ketamine (50 mg/kg) and xylazine (10 mg/kg), the animal’s common bile duct was tied on both sides and cut in half, resulting in cholestasis seven days later. Simultaneously on the rest 20 rats, all surgeries were performed except ligation of the bile duct, and these animals were considered sham-operated (19). Generally, the rats were divided into four groups, and seven days after surgery, sampling was done under approved ethical protocols. Furthermore, they were sacrificed after anesthesia was induced by ether using a complete blood sample from the heart.
In the present case-control study, the animal population consisted of four groups of 10 rats, as follows: (1) Group 1, sham-control: Surgery except for BDL, daily intake: 500 μL normal saline; (2) group 2, cholestatic: Surgery with BDL, daily intake: 500 μL normal saline; (3) group 3, sham + OD: Surgery except for BDL, daily intake: OD ethanolic extract at a dose of 500 mg/kg for seven days (20); and (4) group 4, cholestatic + OD: Surgery with BDL, daily intake: OD ethanolic extract at a dose of 500 mg/kg for seven days.
Blood was taken from rats seven days after surgery, and plasma was isolated and stored for further analysis. After sacrificing, the livers were isolated and dissected into two parts: the first part was immediately submerged in a 10% neutral formalin solution aimed at histopathological examinations, while the second part was prepared as a homogenate (homogenized with a homogenizer) in phosphate-buffered saline (PBS) (pH = 7.4, 50 mM) for the measurement of biochemical parameters and pro-inflammatory proteins (21).
3.3. Biochemical Analyses
In the isolated plasma, the important parameters of liver enzymes like alanine aminotransferase (ALT), aspartate aminotransferase (AST), alkaline phosphatase (ALP), total protein, and total bilirubin were determined using a kit (Pars Azmoon kit, Iran) and calorimetric method (22).
3.4. Determination of Oxidative Stress
3.4.1. The Measurement of the Total Antioxidant Capacity of Plasma
The ferric reducing antioxidant power (FRAP) test was performed to determine the total antioxidant potency of the rats’ plasma. The synthesis of a blue-colored Fe2+-tripyridyl-triazine (TPTZ) complex from the colorless oxidized Fe3+ formed by the electron-donating characteristics of antioxidants is detected using this approach. The resulting color intensity can be analyzed spectrophotometrically at wavelength 593 nm (23).
3.4.2. The Measurement of Nitric Oxide Metabolite
The Griess technique was used to determine the plasma levels of NO metabolite (nitric oxide content) (24).
3.4.3. The Measurement of Sulfhydryl Protein Content
In order to analyze the sulfhydryl protein content, 790 μL of absolute methanol solution and 150 μL of Tris base-EDTA (ethylenediaminetetraacetic acid) buffer were initially mixed in the test tube, then 50 μL of homogenized tissue was added. After thoroughly mixing, 50 μL of the dithiobis nitrobenzoic acid (DNTB) reagent was added and vortexed. In that other tube, the same amount of sample was added, along with 50 μL of the homogenized tissue, but no DNTB reagent was added (blank sample). The reagents were poured into the third tube without spilling any protein (reagent blank). The blank sample and the blank reagent remove excess protein-related adsorption and DNTB reagent-related light absorption; consequently, the light absorption resulting from the reaction of the thiol groups will only be retained. The test tubes were incubated for 15 min at room temperature, and then it was read at 412 nm (25).
3.4.4. The Measurement of Carbonyl Protein Amount
The carbonyl group concentration, which quantifies the oxidative alteration of proteins, was measured spectrophotometrically using 2,4-dinitrophenylhydrazine, a standard carbonyl reagent (24).
3.4.5. The Measurement of the Malondialdehyde Amount
Measurement of the malondialdehyde (MDA) concentration in homogenized liver tissue was performed following the reaction with thiobarbituric acid (TBA), and analysis of the amount of light absorption of the red product was read at 412 nm (24).
3.5. Determination of Enzymatic Antioxidant
3.5.1. The Measurement of Catalase Activity
Hugo Aebi’s method investigated CAT enzyme activity in red blood cells. The breakdown rate of the H2O2 substrate was determined using a spectrophotometer at 240 nm in this approach (26).
3.5.2. The Measurement of Superoxide Dismutase Activity
A colorimetrically enzymatic test kit (Karmania Pars Gene, Kerman, Iran) was used to detect SOD activity. The anion superoxide was utilized in this technique to convert H2O2; finally, the produced product changes the existing chromogen into a color that an ELISA reader can read at 420 nm (27).
3.5.3. The Measurement of Glutathione Peroxidase
This activity was analyzed using an approach that relied on the reduction of glutathione disulfide (GSSG) by nicotinamide adenine dinucleotide phosphate oxidase (NADPH), followed by the absorbance declining at 340 nm spectrophotometrically (28).
3.6. Preparation of Tissue for Microscopic Examinations (Pathological Investigation)
The liver tissue specimens were initially fixed in 8% formalin buffer for histopathological investigation. The specimens were then dehydrated with alcohol and molded in paraffin; 5 μm tissue blocks were made and stained with hematoxylin and eosin staining.
In accordance with the previous studies, the histopathological grade and the score of BDL-induced liver injury are shown in Table 1 (29).
Histopathological Grade/Score of Bile Duct Ligation-Induced Liver Injury
Histopathologic Type | Histopathological Grade/Score | ||||
---|---|---|---|---|---|
Bile duct proliferation | None: 0 mild:1: ↓ < 20% | Moderate:2 : 20 - 50% | Marked/severe: 3 (↑ < 50%): | ||
Portal inflammation | None: 0 | Mild:1 | Moderate: 2 | Marked/severe: 3 | |
Focal inflammation | None: 0 | ↓ < 1 focal: 1 | 2 - 4 focal: 2 | 5 - 10 focal: 3 | ↑ < 10 focal: 4 |
Piecemeal necrosis | None: 0 | Mild:1 | Mild/moderate: 2 | Moderate: 3 | Severe: 4 |
Portal tract fibrosis | None: 0 | Fibrous expansion of some portal areas:1 | Fibrous expansion of most portal areas: 2 | Marked bridging: 3Cirrhosis: 4 |
According to previous studies, the grade and histopathological score of liver damage caused by BDL are shown in Table 1.
Bile duct proliferation: none, 0; mild, 1 (↓ < 20%); moderate, 2 (20 - 50%); marked/severe, 3 (↑ < 50%).
Portal inflammation: none, 0; mild, 1; moderate, 2; marked/moderate,3; marked/severe,4.
Focal inflammation: none, 0; ↓ < 1 focal, 1; 2 - 4 focal, 2; 5 - 10 focal, 3; ↑ < 10 focal, 4.
Piecemeal necrosis: none, 0; mild, 1; mild/moderate, 2; moderate, 3; severe, 4.
Portal tract fibrosis: none, 0; fibrous expansion of some portal areas, 1; fibrous expansion of most portal areas, 2; marked bridging, 3; cirrhosis, 4.
3.7. Antifungal and Antibacterial Activities
In order to evaluate the antifungal properties, the broth microdilution method was utilized based on the CLSI-M27 A2 guideline. The fresh colony of each isolate was used for standard suspension. Serial dilution of the OD extract was prepared in RPMI 1640 from 16000 μg/mL to 62.5 μg/mL. Subsequently, 100 μL of the diluted standard suspension in RPMI (1: 1000) was added to 96-well microplates, and serial dilution of the extract was added to each well. Finally, after 24 h of incubation, the minimum inhibitory concentration (MIC), MIC50, and MIC90 were calculated for each isolate.
The antibacterial activity of the extract was also determined based on the CLSI-M100 guideline (reference). In brief, 10 Staphylococcus aureus strains were selected, including nine clinical (five wound infections, four bacteremia isolates) and one standard isolate (ATCC-25923). To prepare the serial drug dilution, 4 mg of the ethanolic extract was dissolved in distilled water. Then, 100 μL of each serial dilution was added to 10 μL of the standard suspension with 990 μL of Mueller Hinton broth medium (Sigma, Germany) and incubated for 24 h at 37°C. The minimum bactericidal concentration (MBC) and MIC values were finally determined for each isolate.
3.8. Statistical Analysis
All data are presented as mean ± SE. SPSS software was used to analyze the data, including a one-way variance analysis (ANOVA) and Tukey’s multiple comparison test. Statistical significance was defined as a P-value < 0.05.
4. Results
4.1. The Effect of Oliveria decumbens Extract on Biochemical Parameters in Bile Duct Ligation-Induced Cholestasis
According to our data, the BDL group had significantly higher amounts of ALT, ALP, AST, total protein, and bilirubin than the SC group (P < 0.05). Oliveria decumbens extract at a dosage of 500 mg/kg in the cholestatic group lowered ALT, protein, and total bilirubin levels are slightly more than in the BDL group, but it significantly reduced AST and ALP levels (Figure 1) (P < 0.05).
Effect of the Oliveria decumbens extract on biochemical markers in bile duct ligation-induced cholestatic rats. A, Aspartate aminotransferase; B, alanine aminotransferase; C, alkaline phosphatase; D, total bilirubin; E, total protein. Each value represents the mean ± SEM. ∗ Significantly different from the SC group (P < 0.05). # Significantly different from BDL (P < 0.05). Abbreviations: ALT, alanine aminotransferase; ALP, alkaline phosphatase; AST, aspartate aminotransferase; BDL, bile duct ligation; OD, Oliveria decumbens; SC, sham-control.
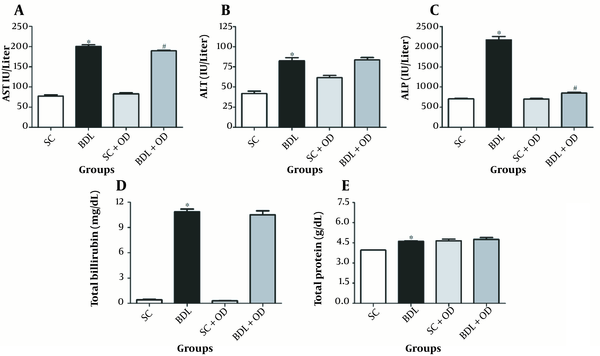
4.2. The Effect of Oliveria decumbens Extract on Plasma Oxidative Stress Markers in BDL-Induced Cholestasis
As seen in Figure 2, the plasma concentration of FRAP, MDA, protein oxidation (PCO), and nitrogen species (NO) in the BDL group were remarkably greater than in the SC group (P < 0.05). Although our findings indicated no meaningful effect of the OD on MDA compared to only the BDL group, the antioxidant activity (evaluated via FRAP assay) was significantly elevated in the BDL-OD group; in addition, the PCO and NO were notably decreased (P < 0.05).
Effect of the Oliveria decumbens extract on A, antioxidant activity (FRAP assay); B, malondialdehyde plasma levels; C, protein oxidation (PCO); and D, nitric oxide metabolites. Each value represents the mean ± SEM. ∗ Significantly different from SC (P < 0.05); # Significantly different from BDL (P < 0.05). Abbreviations: BDL, bile duct ligation; FRAP, ferric reducing antioxidant power; MDA, malondialdehyde; NO, nitric oxide; OD, Oliveria decumbens; PCO, carbonyl protein; SC, sham-control.
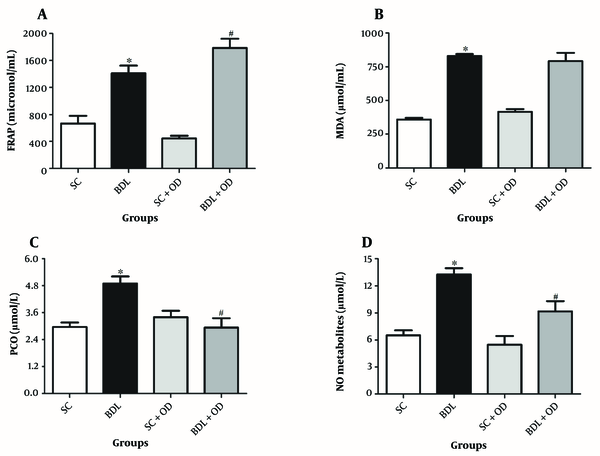
4.3. The Effect of Oliveria decumbens Extract on Liver Oxidative Stress Markers in Bile Duct Ligation-Induced Cholestasis
The total thiol (TT) amount in the BDL group did not differ from that in the SC group. In comparison to the SC group, the antioxidant power, MDA, and NO amounts in the BDL group were significantly greater (P < 0.05). Furthermore, our findings demonstrated that the OD extract had no significant influence on TT and MDA levels, but FRAP and NO were considerably reduced compared to the BDL group (Figure 3) (P < 0.05).
Effect of the Oliveria decumbens extract on the tissue oxidative stress markers. A, Ferric reducing activity of plasma; B, total thiol; C, malondialdehyde; and D, nitric oxide. Each value represents the mean ± SEM. ∗ Significantly different from SC (P < 0.05). # Significantly different from BDL (P < 0.05). Abbreviations: BDL, bile duct ligation; FRAP, ferric reducing activity of plasma; MDA, malondialdehyde; NO, nitric oxide; OD, Oliveria decumbens. SC, sham-control; TT, total thiol.
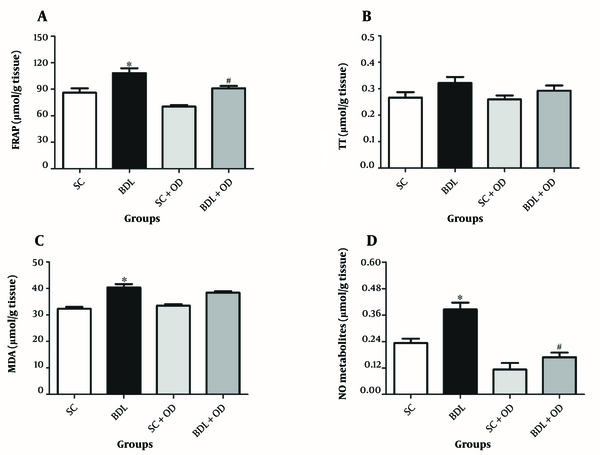
4.4. The Effect of Oliveria decumbens Extract on Enzymatic Antioxidants in Bile Duct Ligation-Induced Cholestasis
As shown in Figure 4, the GPx and SOD activities were not altered in the BDL group in comparison with the SC group; however, the CAT content was significantly decreased. Administration of the OD meaningfully increased the SOD activity in the BDL-OD group compared to the BDL group. However, it had no remarkable effect on the CAT and GPx activities.
Effect of the Oliveria decumbes extract on the A, catalase; B, superoxide dismutase; and C, glutathione peroxidase activities in the liver tissue. Each value represents the mean ± SEM. # Significantly different from the BDL group. Abbreviations: BDL, bile duct ligation; CAT, catalase; GPx, glutathione peroxidase; OD, Oliveria decumbes; SC, sham-control; SOD, superoxide dismutase.
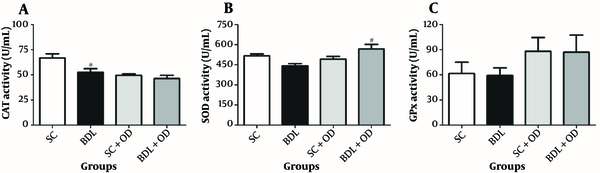
4.5. The Effect of Oliveria decumbens Extract on Histological Parameters in BDL-Induced Cholestasis
Figure 5 displays microscopic pictures of the liver specimen stained with hematoxylin–eosin. The sham-operated rats had normal liver structure without fibrosis (score: 0), whereas the bile duct ligation rats showed bile duct proliferation within two weeks (score:3); meanwhile, spreading out of fibrosis to the portal areas was observed (score: 3). The microscopic images of liver specimens from the BDL group indicated localized aggregation of inflammatory cells in hepatic parenchyma as well as apoptotic cells, focal inflammation, and severe portal inflammation (score: 2). Following these results, we observed that rats treated with the OD extract exhibited a significant decline in bile duct proliferation (score: 1) (Table 2).
Liver photomicrographs represent histopathological alterations in cholestatic rats (stained with hematoxylin and eosin). Abbreviations: BDL, bile duct ligation; OD, Oliveria decumbens; SC, sham-control.
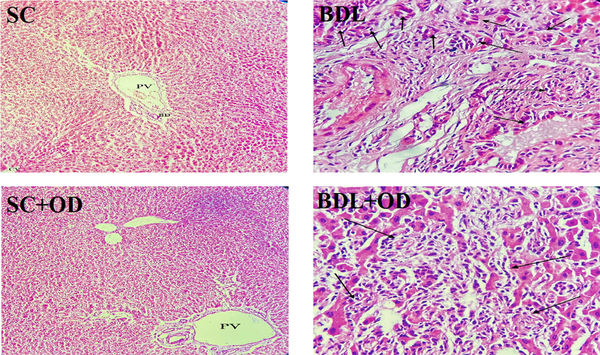
Effect of the Oliveria decumbens Extract on the Histopathological Indices in Bile Duct Ligation-Induced Cholestasis Rats
Group/Score | Bile Duct Proliferation | Portal Inflammation | Piecemeal Necrosis | Focal Inflammation | Portal Tract Fibrosis |
---|---|---|---|---|---|
SC | 0 | 0 | 0 | 0 | 0 |
BDL | 3 | 2 | 2 | 2 | 2 |
SC + OD | 0 | 0 | 0 | 0 | 0 |
BDL + OD | 1 | 2 | 2 | 2 | 1 |
4.6. Antifungal and Antibacterial Susceptibility Effects
The MIC values for C. albicans isolates ranged from 125 - 8000 μg/mL. This parameter was lower in blood culture isolate (250 - 500 μg/mL) than in vaginitis isolate (500 - 8000 μg/mL). For the S. aureus isolates, the MIC value was calculated at 18,750 μg/mL, while the clinical isolates were approximately equal to the standard isolate (ATCC-25923).
5. Discussion
Hepatic cholestasis is a common reason for liver cirrhosis and fibrosis, and BDL is an established experimental model that can mimic the mechanism of hepatic cholestasis. The double duct closure in mice has been used as an experimental model for a long time. This method was introduced over three decades ago, in which blockage of the ducts causes cirrhosis and changes in mice's liver that are identical to those seen in human cirrhosis (30). In this in vivo model, the necrosis and apoptosis of hepatocytes, eventually fibrosis, and cirrhosis, are generated by cytotoxic components of bile such as bile lipophilic acid, plasma membrane injury, and oxidative stress (31). In the current study, the antioxidant and protective properties of the OD extract, as well as the role of this plant in the amount of pro-inflammatory proteins in the mice’s BDL, were investigated.
The present study showed that plasma levels of the most sensitive biomarkers of liver injury, including; AST, ALT, ALP, total bilirubin, and total protein were significantly higher in the BDL group than in the SC group, which is in agreement with the results of other studies (32-34).
The AST and ALT are mostly found in hepatocytes and released into the blood when the liver cell membranes are injured due to duct occlusion. These alterations in liver enzymes result in the loss of liver tissue integrity and the death of hepatocytes (35, 36). In our study, OD treatment notably reduced AST and slightly reduced ALT levels in BDL-induced cholestatic. The perusalses show that the OD extract prevents the release of AST and ALT in the blood and lowers liver injury by preventing free radicals generation due to its antioxidant properties.
The main factor contributing to the pathophysiology of BDL-induced hepatic cholestasis is the development of oxidative stress (3). Bile duct ligation increases ROS formation and disturbs oxidants’ and antioxidants’ equilibrium (37). Hydrophobic bile acids have also been shown to directly injure hepatocytes by generating intracellular ROS by activating kupffer cells, resulting in oxidative lipid modification, apoptosis, and protein oxidation (38). The accumulated MDA in particular organs indicates lipid peroxidation and oxidative stress. In the present research, rising MDA levels in rats’ plasma and liver tissue were observed, according to the results presented by Mohamed et al. (39), although the OD treatment did not affect the MDA content.
Ferric reducing antioxidant power is an index reflecting non-enzymatic antioxidants. In the present study, a rise in FRAP capacity was seen after seven days. These results verified Sadeghi et al.’s finding, reporting a significant increase in the plasma FRAP levels of the BDL-induced cholestatic animals compared to the SC group (37). Furthermore, we observed a remarkable rise in plasma FRAP levels after consuming the OD hydroalcoholic extract in cholestatic rats. Since phenolics and flavonoids are the main antioxidant active compounds (40), the OD extract, rich in these phytoconstituents, can preserve liver cells from oxidative damage triggered by BDL by suppressing and trapping free radicals.
Nitric oxide free radical is a highly reactive molecule having less than a one-second half-life, released by endothelial, Kupffer, and hepatic cells in response to various triggers in the liver (37). Nitric oxide, through its peroxynitrite derivative, as a powerful oxidizing agent, is mainly responsible for oxidative damage that causes damage to proteins, lipids, DNA, and biological membranes (41). According to the literature, the NO content is increased in both tissues and plasma of BDL rats (24, 42); however, our findings demonstrated that the NO level was significantly lower in OD-treated rats than in the BDL group.
Nowadays, due to the side effects of chemical drugs, using herbal medicines has attracted the attention of contemporary researchers (43). In terms of biochemical improvement, previous experiments have revealed that natural antioxidants frequently offer potential free-radical scavenging capabilities and anti-inflammatory properties, which are important variables in cholestatic therapy (44). In this sense, OD, due to its antioxidant capacity, can considerably decrease the formation of NO and/or neutralize it, thus protecting the liver against oxidative damage.
In the current study, protein carbonyl compounds, markers of oxidative protein stress, were enhanced in the plasma of cholestatic rats. Since proteins are often catalysts rather than stoichiometric mediators, they are more prone to oxidative damage in cells (45); As a result, oxidized protein accumulation may damage liver functioning through redox status disturbance. The OD extract significantly reversed these alterations, which might be attributed to its antioxidant action, which was shown in our study.
Enzymatic antioxidants (SOD, CAT, and GPx) have been recognized as mutually beneficial defensive mechanisms against ROS. Reactive oxygen species formation can cause considerable damage to cell organelles, causing intracellular antioxidant enzymes to fail under oxidative stress (38). Our findings demonstrated that bile duct ligation reduced CAT and SOD activity. This reduction can result in increased superoxide radical absorption, which aids causes lipid peroxidation.
The decrease in antioxidative enzymes following BDL might be attributed to ROS-induced protein suppression because the oxidative injury can destroy a particular protein component (SOD), which facilitates the formation of H2O2 and O2 from superoxide anion. In addition to CAT, which plays a vital role in decomposing H2O2 into O2 and H2O, GPx is a critical enzyme that catalyzes the reduction of H2O2 (46, 47). Increased GPx and SOD levels in liver tissue can decrease oxidative stress-mediated injury in cholestatic animal models (48, 49). Our findings showed that OD therapy in BDL-induced cholestatic rats significantly promotes the functions of SOD and GPx in liver tissue. This rebalancing is predominantly due to the high antioxidant potential of the plant, associated with secondary metabolite contents (i.e., flavonoids, phenolic acids, and polyphenols). Because flavonoids inhibit the free radical formation and chelate metal ions by their highly active hydroxyl group (C3-OH), OD, through regulating antioxidant defense mechanisms, inhibit the occurrence of oxidative stress and maintains hepatic function in BDL-induced cholestatic rats.
Based on preclinical and clinical findings, it has been determined that the main apoptotic trigger in different liver diseases is chronic inflammation, which is influenced by the accumulation of hydrophobic bile salts in the liver, which are toxic to hepatocytes and bile duct epithelial cells (50). Many microscopic alterations occur when a large bile duct is blocked along its path. Bile-duct occlusion causes portal fibrosis in rats, starting with bile duct and portal periductular fibroblast proliferation and spreading between adjacent portal ducts. In this research, the expansion of proliferated bile ducts into portal regions, localized and portal inflammation, and fragment necrosis in the BDL group were all signs of bile duct proliferation and fibrosis in portal ducts. The present histopathological findings confirm the study of Moslemi et al. (51). Our research showed that OD reduced bile duct proliferative associated with BDL-induced extrahepatic cholestasis through antioxidant and anti-inflammatory actions.
In the current study, the OD extract demonstrated no effective activity against S. aureus isolates. Mahboubi et al. showed that spore-forming bacteria, especially Bacillus species, were resistant to OD essential oil (52). Moreover, they reported a lower MIC value (0.25 μg/mL) against C. albicans than our finding, confirming the higher anti-Candida albicans of the OD essential oil versus its hydroethanolic extract. Amin et al. similarly exhibited that OD essential oil had antifungal efficacy against C. albicans and Aspergillus niger isolates (53). Overall, OD indicated potent antifungal activity; however, antibacterial activity varied based on the bacteria species and the OD product.
In this study, the protective effect of the hydroalcoholic extract of OD on BDL-induced cholestasis was observed by reducing aminotransferases, suppressing oxidative stress, increasing intracellular antioxidants, and inhibiting inflammation in the liver. In this context, studies have been conducted on the effects of OD extract and its compounds on hepatotoxicity caused by different substances, confirming our study’s results. One study investigated the effect of hepatoprotective OD extract against hepatotoxicity induced by cadmium. Oliveria decumbens extract reduced the levels of AST, ALT, and ALP, which is likely due to the presence of potent antioxidant components in OD extract, which shields the liver cells from oxidative stress and stops the release of liver enzymes into the blood by generating membrane stability in the cells (20). According to gas chromatographic analysis, thymol, carvacrol, p-cymene, and γ-terpinene are the main compounds that are abundant in OD essential oils (11). The remedial properties of OD can chiefly be ascribed to its volatile components, such as carvacrol and thymol (12, 13). Thymol has a strong ameliorative effect against hydrocortisone-induced oxidative stress injury in hepatic tissues by decreased AST, ALT, and total oxidative capacity (TOC), as well as a significant increase in serum total protein, albumin, and total antioxidant capacity (TAC) (54). Carvacrol improved oxidative stress, inflammation, and liver dysfunction induced by lipopolysaccharide (LPS) through increased AST, ALT, ALP, interleukin-1β (IL-1β), MDA, NO, and decreased total protein, albumin, thiol, SOD, and CAT (55). Treatment of carvacrol lowered serum transaminases, ALP, and MDA levels and elevated SOD and CAT levels in Azathioprine-intoxicated rats (56). In another study, carvacrol suppresses the progression of liver fibrosis via its antioxidant, anti-inflammatory, and anti-apoptotic effects. Carvacrol decreased thioacetamide-increased serum liver enzymes, AST, ALT, ALP, and gamma-glutamyl transferase (GGT) and direct bilirubin and total bilirubin levels as well as increased total protein (TP) and albumin levels. Carvacrol reduced glutathione depletion (GSH), NO, and MDA accumulation in liver tissue. The anti-inflammatory effect of carvacrol was confirmed by decreasing nuclear factor kappa B (NF-κB), IL-1β, and inducible nitric oxide synthase (iNOS) contents (57). Oral administration of carvacrol had a significant protective and antioxidant effect against D-GalN-induced damages by reducing AST, ALT, ALP, and GGT and increasing SOD, CAT, and glutathione peroxidase in the plasma and liver (58). This evidence shows that the protective effects of OD extract are probably due to the presence of compounds such as thymol, carvacrol, and other compounds.
5.1. Conclusion
In summary, our results demonstrated the potential protective effect of OD hydro-ethanolic extract on bile duct ligation-induced cholestasis rats. Their hepatic protective activity was probably related to the decreased hepatic aminotransferases, suppression of oxidative stress, increased intracellular antioxidants, and inhibition of hepatic inflammation. Consequently, we propose that the present findings are predominantly owing to the significant content of phenolic compounds, which are well-known antioxidants playing pivotal roles in curing and preventing several diseases. Moreover, the OD application may represent a promising and valuable strategy for treating cholestasis. Further phytochemical investigations can promisingly perform to discover the liver protective phyto-based drug candidate to be rendered for complementary drug development procedure.
Acknowledgements
References
-
1.
Messelmani T, Morisseau L, Sakai Y, Legallais C, Le Goff A, Leclerc E, et al. Liver organ-on-chip models for toxicity studies and risk assessment. Lab Chip. 2022;22(13):2423-50. [PubMed ID: 35694831]. https://doi.org/10.1039/d2lc00307d.
-
2.
Izadpanah E, Hassanzadeh K, Yousefinejad V, Shahveisi K, Fatahi N, Moloudi MR. [Effect of selegiline on liver cholestasis induced by bile duct ligation in rat]. Sci J Kurdistan Univ Medical Sci. 2016;21(5):20-30. Persian.
-
3.
Ommati MM, Amjadinia A, Mousavi K, Azarpira N, Jamshidzadeh A, Heidari R. N-acetyl cysteine treatment mitigates biomarkers of oxidative stress in different tissues of bile duct ligated rats. Stress. 2021;24(2):213-28. [PubMed ID: 32510264]. https://doi.org/10.1080/10253890.2020.1777970.
-
4.
Cao WR, Ge JQ, Xie X, Fan ML, Fan XD, Wang H, et al. Protective effects of petroleum ether extracts of Herpetospermum caudigerum against alpha-naphthylisothiocyanate-induced acute cholestasis of rats. J Ethnopharmacol. 2017;198:139-47. [PubMed ID: 28065777]. https://doi.org/10.1016/j.jep.2017.01.003.
-
5.
Zhang L, Bansal MB. Role of Kupffer Cells in Driving Hepatic Inflammation and Fibrosis in HIV Infection. Front Immunol. 2020;11:1086. [PubMed ID: 32612603]. [PubMed Central ID: PMC7308419]. https://doi.org/10.3389/fimmu.2020.01086.
-
6.
Copple BL, Jaeschke H, Klaassen CD. Oxidative stress and the pathogenesis of cholestasis. Semin Liver Dis. 2010;30(2):195-204. [PubMed ID: 20422501]. https://doi.org/10.1055/s-0030-1253228.
-
7.
Tiwari S, Tiwari S, Singh M, Singh A, Prasad SM. Generation Mechanisms of Reactive Oxygen Species in the Plant Cell. In: Singh VP, Singh S, Tripathi DK, Prasad SM, Chauhan DK, editors. Reactive Oxygen Species in Plants: Boon Or Bane - Revisiting the Role of ROS. New Jersey, USA: John Wiley & Sons Ltd; 2017. p. 1-22. https://doi.org/10.1002/9781119324928.ch1.
-
8.
Ano Bom AP, Rangel LP, Costa DC, de Oliveira GA, Sanches D, Braga CA, et al. Mutant p53 aggregates into prion-like amyloid oligomers and fibrils: implications for cancer. J Biol Chem. 2012;287(33):28152-62. [PubMed ID: 22715097]. [PubMed Central ID: PMC3431633]. https://doi.org/10.1074/jbc.M112.340638.
-
9.
Catala A. Lipid peroxidation modifies the picture of membranes from the "Fluid Mosaic Model" to the "Lipid Whisker Model". Biochimie. 2012;94(1):101-9. [PubMed ID: 21983178]. https://doi.org/10.1016/j.biochi.2011.09.025.
-
10.
Bayir H. Reactive oxygen species. Crit Care Med. 2005;33(12 Suppl):S498-501. [PubMed ID: 16340433]. https://doi.org/10.1097/01.ccm.0000186787.64500.12.
-
11.
Eftekhari M, Shams Ardekani MR, Amin M, Attar F, Akbarzadeh T, Safavi M, et al. Oliveria decumbens, a Bioactive Essential Oil: Chemical Composition and Biological Activities. Iran J Pharm Res. 2019;18(1):412-21. [PubMed ID: 31089376]. [PubMed Central ID: PMC6487399].
-
12.
Karami A, Khoushbakht T, Esmaeili H, Maggi F. Essential Oil Chemical Variability in Oliveria decumbens (Apiaceae) from Different Regions of Iran and Its Relationship with Environmental Factors. Plants (Basel). 2020;9(6). [PubMed ID: 32471254]. [PubMed Central ID: PMC7356547]. https://doi.org/10.3390/plants9060680.
-
13.
Didry N, Dubreuil L, Pinkas M. [Antibacterial activity of thymol, carvacrol and cinnamaldehyde alone or in combination]. Pharmazie. 1993;48(4):301-4. [PubMed ID: 8321882].
-
14.
Jamali T, Kavoosi G, Ardestani SK. In-vitro and in-vivo anti-breast cancer activity of OEO (Oliveria decumbens vent essential oil) through promoting the apoptosis and immunomodulatory effects. J Ethnopharmacol. 2020;248:112313. [PubMed ID: 31655147]. https://doi.org/10.1016/j.jep.2019.112313.
-
15.
Cassone A, Cauda R. Candida and candidiasis in HIV-infected patients: where commensalism, opportunistic behavior and frank pathogenicity lose their borders. AIDS. 2012;26(12):1457-72. [PubMed ID: 22472853]. https://doi.org/10.1097/QAD.0b013e3283536ba8.
-
16.
Mariotti V, Strazzabosco M, Fabris L, Calvisi DF. Animal models of biliary injury and altered bile acid metabolism. Biochim Biophys Acta Mol Basis Dis. 2018;1864(4 Pt B):1254-61. [PubMed ID: 28709963]. [PubMed Central ID: PMC5764833]. https://doi.org/10.1016/j.bbadis.2017.06.027.
-
17.
Ackerman Z, Weinreb M, Amir G, Pollak RD. Bone mineral metabolism and histomorphometry in rats with cholestatic liver disease. Liver. 2002;22(2):166-72. [PubMed ID: 12028412]. https://doi.org/10.1046/j.0106-9543.2002.01566.x.
-
18.
Nadimi M, Zia M, Madani M. The effect of aqueous and ethanolic extracts of Teucrium polium on Candida albicans and two species of Malassezia. Zahedan J Res Med Sci. 2013;15(8):34-8.
-
19.
Doustimotlagh AH, Dehpour AR, Etemad-Moghadam S, Alaeddini M, Kheirandish Y, Golestani A. Nitrergic and opioidergic systems affect radiographic density and histomorphometric indices in bile-duct-ligated cirrhotic rats. Histol Histopathol. 2017;32(7):743-9. [PubMed ID: 27782295]. https://doi.org/10.14670/HH-11-836.
-
20.
Rahimi Kazerouni S, Mokhtari M, Shariati M, Rahimi Kazerooni M. [Hepatoprotective effect of hydro-alcoholic extract of Oliveria decumbens against hepatotoxicity induced by cadmium chloride in adult male rat]. Medical Science Journal of Islamic Azad Univesity-Tehran Medical Branch. 2015;25(2):105-11.
-
21.
Noeman SA, Hamooda HE, Baalash AA. Biochemical study of oxidative stress markers in the liver, kidney and heart of high fat diet induced obesity in rats. Diabetol Metab Syndr. 2011;3(1):17. [PubMed ID: 21812977]. [PubMed Central ID: PMC3174870]. https://doi.org/10.1186/1758-5996-3-17.
-
22.
Lin SY, Wang YY, Chen WY, Liao SL, Chou ST, Yang CP, et al. Hepatoprotective activities of rosmarinic acid against extrahepatic cholestasis in rats. Food Chem Toxicol. 2017;108(Pt A):214-23. [PubMed ID: 28789951]. https://doi.org/10.1016/j.fct.2017.08.005.
-
23.
Hsieh C, Rajashekaraiah V. Ferric reducing ability of plasma: a potential oxidative stress marker in stored plasma. Acta Haematologica Polonica. 2021;52(1):61-7. https://doi.org/10.5603/ahp.2021.0009.
-
24.
Sadeghi H, Azarmehr N, Razmkhah F, Sadeghi H, Danaei N, Omidifar N, et al. The hydroalcoholic extract of watercress attenuates protein oxidation, oxidative stress, and liver damage after bile duct ligation in rats. J Cell Biochem. 2019;120(9):14875-84. [PubMed ID: 31016763]. https://doi.org/10.1002/jcb.28749.
-
25.
Doustimotlagh AH, Kokhdan EP, Vakilpour H, Khalvati B, Barmak MJ, Sadeghi H, et al. Protective effect of Nasturtium officinale R. Br and quercetin against cyclophosphamide-induced hepatotoxicity in rats. Mol Biol Rep. 2020;47(7):5001-12. [PubMed ID: 32533401]. https://doi.org/10.1007/s11033-020-05556-7.
-
26.
Aebi H. Catalase in vitro. Methods Enzymol. 1984;105:121-6. [PubMed ID: 6727660]. https://doi.org/10.1016/s0076-6879(84)05016-3.
-
27.
Paoletti F, Mocali A. Determination of superoxide dismutase activity by purely chemical system based on NAD(P)H oxidation. Methods Enzymol. 1990;186:209-20. [PubMed ID: 2233293]. https://doi.org/10.1016/0076-6879(90)86110-h.
-
28.
Wendel A. Glutathione peroxidase. Methods Enzymol. 1981;77:325-33. [PubMed ID: 7329310]. https://doi.org/10.1016/s0076-6879(81)77046-0.
-
29.
Dresner-Pollak R, Gabet Y, Steimatzky A, Hamdani G, Bab I, Ackerman Z, et al. Human parathyroid hormone 1-34 prevents bone loss in experimental biliary cirrhosis in rats. Gastroenterology. 2008;134(1):259-67. [PubMed ID: 18061175]. https://doi.org/10.1053/j.gastro.2007.10.025.
-
30.
Tag CG, Sauer-Lehnen S, Weiskirchen S, Borkham-Kamphorst E, Tolba RH, Tacke F, et al. Bile duct ligation in mice: induction of inflammatory liver injury and fibrosis by obstructive cholestasis. J Vis Exp. 2015;(96). [PubMed ID: 25741630]. [PubMed Central ID: PMC4354634]. https://doi.org/10.3791/52438.
-
31.
Arduini A, Serviddio G, Tormos AM, Monsalve M, Sastre J. Mitochondrial dysfunction in cholestatic liver diseases. Front Biosci (Elite Ed). 2012;4(6):2233-52. [PubMed ID: 22202034]. https://doi.org/10.2741/539.
-
32.
Tahan G, Tarcin O, Tahan V, Eren F, Gedik N, Sahan E, et al. The effects of N-acetylcysteine on bile duct ligation-induced liver fibrosis in rats. Dig Dis Sci. 2007;52(12):3348-54. [PubMed ID: 17436097]. https://doi.org/10.1007/s10620-006-9717-9.
-
33.
Li P, Yang Y, Lin Z, Hong S, Jiang L, Zhou H, et al. Bile Duct Ligation Impairs Function and Expression of Mrp1 at Rat Blood-Retinal Barrier via Bilirubin-Induced P38 MAPK Pathway Activations. Int J Mol Sci. 2022;23(14). [PubMed ID: 35887010]. [PubMed Central ID: PMC9318728]. https://doi.org/10.3390/ijms23147666.
-
34.
Cho I, Koo BN, Kam EH, Lee SK, Oh H, Kim SY. Bile duct ligation of C57BL/6 mice as a model of hepatic encephalopathy. Anesth Pain Med (Seoul). 2020;15(1):19-27. [PubMed ID: 33329785]. [PubMed Central ID: PMC7713851]. https://doi.org/10.17085/apm.2020.15.1.19.
-
35.
Amalia PM, Possa MN, Augusto MC, Francisca LS. Quercetin prevents oxidative stress in cirrhotic rats. Dig Dis Sci. 2007;52(10):2616-21. [PubMed ID: 17431769]. https://doi.org/10.1007/s10620-007-9748-x.
-
36.
El-Lakkany NM, Seif El-Din SH, Sabra AA, Hammam OA, Ebeid FA. Co-administration of metformin and N-acetylcysteine with dietary control improves the biochemical and histological manifestations in rats with non-alcoholic fatty liver. Res Pharm Sci. 2016;11(5):374-82. [PubMed ID: 27920819]. [PubMed Central ID: PMC5122826]. https://doi.org/10.4103/1735-5362.192487.
-
37.
Sadeghi H, Jahanbazi F, Sadeghi H, Omidifar N, Alipoor B, Kokhdan EP, et al. Metformin attenuates oxidative stress and liver damage after bile duct ligation in rats. Res Pharm Sci. 2019;14(2):122-9. [PubMed ID: 31620188]. [PubMed Central ID: PMC6791170]. https://doi.org/10.4103/1735-5362.253359.
-
38.
Fahmy SR. Anti-fibrotic effect of Holothuria arenicola extract against bile duct ligation in rats. BMC Complement Altern Med. 2015;15:14. [PubMed ID: 25652675]. [PubMed Central ID: PMC4328034]. https://doi.org/10.1186/s12906-015-0533-7.
-
39.
Mohamed AA, Saad DA, Francis MM, Mohamed FA. Possible Protective Effect of Onion Supplementation on Hepatic Functional and Structural Alterations Induced by Cholestasis. Med J Cairo Univ. 2020;87(5):3491-9.
-
40.
Mottaghipisheh J, Taghrir H, Boveiri Dehsheikh A, Zomorodian K, Irajie C, Mahmoodi Sourestani M, et al. Linarin, a Glycosylated Flavonoid, with Potential Therapeutic Attributes: A Comprehensive Review. Pharmaceuticals (Basel). 2021;14(11). [PubMed ID: 34832886]. [PubMed Central ID: PMC8621830]. https://doi.org/10.3390/ph14111104.
-
41.
Koubaa FG, Chaabane M, Turki M, Ayadi FM, El Feki A. Anti-oxidant and hepatoprotective effects of Salvia officinalis essential oil against vanadium-induced oxidative stress and histological changes in the rat liver. Environ Sci Pollut Res Int. 2021;28(9):11001-15. [PubMed ID: 33106906]. https://doi.org/10.1007/s11356-020-11303-z.
-
42.
Ara C, Kirimlioglu H, Karabulut AB, Coban S, Ay S, Harputluoglu M, et al. Protective effect of resveratrol against oxidative stress in cholestasis. J Surg Res. 2005;127(2):112-7. [PubMed ID: 16083749]. https://doi.org/10.1016/j.jss.2005.01.024.
-
43.
Mehrabi S, Askarpour E, Mehrabi F, Jannesar R. Effects of hydrophilic extract of Nasturtium officinale on prevention of ethylene glycol induced renal stone in male Wistar rats. J Nephropathol. 2016;5(4):123-7. [PubMed ID: 27921023]. [PubMed Central ID: PMC5125058]. https://doi.org/10.15171/jnp.2016.23.
-
44.
Shen K, Feng X, Pan H, Zhang F, Xie H, Zheng S. Baicalin Ameliorates Experimental Liver Cholestasis in Mice by Modulation of Oxidative Stress, Inflammation, and NRF2 Transcription Factor. Oxid Med Cell Longev. 2017;2017:6169128. [PubMed ID: 28757911]. [PubMed Central ID: PMC5516718]. https://doi.org/10.1155/2017/6169128.
-
45.
Terzioglu D, Uslu L, Simsek G, Atukeren P, Erman H, Gelisgen R, et al. The Effects of Hyperbaric Oxygen Treatment on Total Antioxidant Capacity and Prolidase Activity after Bile Duct Ligation in Rats. J Invest Surg. 2017;30(6):376-82. [PubMed ID: 27922759]. https://doi.org/10.1080/08941939.2016.1257666.
-
46.
Hua W, Zhang S, Lu Q, Sun Y, Tan S, Chen F, et al. Protective effects of n-Butanol extract and iridoid glycosides of Veronica ciliata Fisch. Against ANIT-induced cholestatic liver injury in mice. J Ethnopharmacol. 2021;266:113432. [PubMed ID: 33011367]. https://doi.org/10.1016/j.jep.2020.113432.
-
47.
Eidi A, Mortazavi P, Moghadam JZ, Mardani PM. Hepatoprotective effects of Portulaca oleracea extract against CCl4-induced damage in rats. Pharm Biol. 2015;53(7):1042-51. [PubMed ID: 25472695]. https://doi.org/10.3109/13880209.2014.957783.
-
48.
Nassef N, Abu-Shadi E, El Agaty S, Abdel Hamid G. Quercetin mitigates liver injury in a rat model of liver cholestasis. Bulletin of Egyptian Society for Physiological Sciences. 2020;40(1):84-95. https://doi.org/10.21608/besps.2019.14512.1026.
-
49.
Tokac M, Aydin S, Taner G, Ozkardes AB, Yavuz Taslipinar M, Dogan M, et al. Hepatoprotective and antioxidant effects of lycopene in acute cholestasis. Turk J Med Sci. 2015;45(4):857-64. [PubMed ID: 26422858]. https://doi.org/10.3906/sag-1404-57.
-
50.
Xia JL, Dai C, Michalopoulos GK, Liu Y. Hepatocyte growth factor attenuates liver fibrosis induced by bile duct ligation. Am J Pathol. 2006;168(5):1500-12. [PubMed ID: 16651617]. [PubMed Central ID: PMC1606599]. https://doi.org/10.2353/ajpath.2006.050747.
-
51.
Moslemi Z, Bahrami M, Hosseini E, Mansourian M, Daneshyar Z, Eftekhari M, et al. Portulaca oleracea methanolic extract attenuate bile duct ligation-induced acute liver injury through hepatoprotective and anti-inflammatory effects. Heliyon. 2021;7(7). e07604. [PubMed ID: 34355097]. [PubMed Central ID: PMC8322275]. https://doi.org/10.1016/j.heliyon.2021.e07604.
-
52.
Mahboubi M, Feizabadi MM, Haghi G, Hosseini H. [Antimicrobial activity and chemical composition of essential oil from Oliveria decumbens Vent]. Iranian Journal of Medicinal and Aromatic Plants. 2008;24(1):56-65. Persian.
-
53.
Amin G, Sourmaghi MH, Zahedi M, Khanavi M, Samadi N. Essential oil composition and antimicrobial activity of Oliveria decumbens. Fitoterapia. 2005;76(7-8):704-7. [PubMed ID: 16253439]. https://doi.org/10.1016/j.fitote.2005.06.009.
-
54.
Aboelwafa HR, Yousef HN. The ameliorative effect of thymol against hydrocortisone-induced hepatic oxidative stress injury in adult male rats. Biochem Cell Biol. 2015;93(4):282-9. [PubMed ID: 25821896]. https://doi.org/10.1139/bcb-2014-0154.
-
55.
Mortazavi A, Mohammad Pour Kargar H, Beheshti F, Anaeigoudari A, Vaezi G, Hosseini M. The effects of carvacrol on oxidative stress, inflammation, and liver function indicators in a systemic inflammation model induced by lipopolysaccharide in rats. Int J Vitam Nutr Res. 2021:1-11. [PubMed ID: 34024144]. https://doi.org/10.1024/0300-9831/a000711.
-
56.
Houshmand F, Heidarian E, Shirani Faradonbeh A, Najafi Chaleshtori S. Protective effect of carvacrol against hepato-renal toxicity induced by azathioprine in rats. J Shahrekord Univ Med Sci. 2021;23(4):148-53. https://doi.org/10.34172/jsums.2021.25.
-
57.
El-Gendy ZA, Ramadan A, El-Batran SA, Ahmed RF, El-Marasy SA, Abd El-Rahman SS, et al. Carvacrol hinders the progression of hepatic fibrosis via targeting autotaxin and thioredoxin in thioacetamide-induced liver fibrosis in rat. Hum Exp Toxicol. 2021;40(12):2188-201. [PubMed ID: 34155936]. https://doi.org/10.1177/09603271211026729.
-
58.
Aristatile B, Al-Numair KS, Veeramani C, Pugalendi KV. Effect of carvacrol on hepatic marker enzymes and antioxidant status in D-galactosamine-induced hepatotoxicity in rats. Fundam Clin Pharmacol. 2009;23(6):757-65. [PubMed ID: 19650854]. https://doi.org/10.1111/j.1472-8206.2009.00721.x.