Abstract
Background:
Postmenopausal osteoporosis is a major cause of morbidity in postmenopausal females. Transforming growth factor β1 (TGF-β1) and interleukin 18 (IL-18) play complex roles in normal bone metabolism, and in pathophysiology of postmenopausal osteoporosis.Objectives:
The aim of this study was to design an analytic cross sectional study in order to further clarify the role of TGF-β1 and IL-18 in osteoporosis of postmenopausal females.Methods:
A cross sectional study including 65 postmenopausal osteoporotic females as cases and 69 postmenopausal females of similar age without osteoporosis as controls was conducted. Dual energy X-ray absorptiometry (DXA) was used to determine bone mass density (BMD) of participants and T-scoring was applied to establish whether the patient has osteoporosis or not. Serum TGF-β1 and IL-18 levels were measured by quantitative sandwich Enzyme linked immunosorbent assay (ELISA).Results:
Serum TGF-β1 levels were significantly higher in osteoporotic postmenopausal females than non-osteoporotic individuals (23.8 vs. 15.8 ng/mL; P = 0.009). There was no difference between IL-18 levels in the sera of osteoporotic and non-osteoporotic postmenopausal females in this study. There was a positive correlation between body mass index (BMI) and serum level of TGF-β1 (P = 0.04).Conclusions:
Our study demonstrated that TGF-β1 serum levels is higher in osteoporotic postmenopausal females than non-osteoporotic ones, and probably aberrant increase in TGF-β1 in postmenopausal females can result in uncoupled bone resorption and formation, which leads to osteoporosis.Keywords
1. Background
Postmenopausal osteoporosis is a skeletal disorder in which the bone mass is decreased and micro architecture of the bone is weakened. It is a major cause of morbidity in postmenopausal women and obviously estrogen deficiency plays a key role in bone loss and fragility in this group. However, recently it has been shown that several cytokines and immune factors are involved in the pathogenesis of postmenopausal osteoporosis, and thus it can be considered as an immune disease (1, 2).
Throughout life, bone remodeling continues as a result of osteoblasts and osteoclasts functions and interactions. Osteoblasts are generated from stem cells in the bone marrow, called Mesenchymal stem cells (MSCs), whereas osteoclasts arise from hematopoietic stem cells (HSCs). An exquisite balance is needed in osteoblast and osteoclast functions in order to maintain bone architecture and strength (3, 4).
The transforming growth factor (TGF)-β molecules are a super family of cytokines, including TGF-β, bone morphogenetic proteins (BMPs) and activins, which regulate the growth and differentiation of osteoblasts and osteoclasts (5). The TGF-β1, TGF-β2 and TGF-β3 are closely related mammalian isoforms, with TGF-β1 being the most abundant isoform in the bone (6). There is accumulating evidence demonstrating the role of TGF-β1 in bone development, mineral storage and generation of hematopoietic cells. The TGF-β1 augments proliferation and inhibits apoptosis of osteoblasts, and is a chemoattractant molecule for osteoblast precursors (MSCs) (7, 8). However, there are studies demonstrating that TGF-β1 inhibits osteoblast proliferation and mineralization in later phases, and in late phase of their differentiation, osteoblasts seem to be less sensitive to TGF-β1, and in final differentiation stages (mature osteoblasts) TGF-β1 opposes BMP actions (9, 10). Transforming Growth Factor-β1 has a complex impact on osteoclasts, and recruits them from bone marrow and spleen to the bone, enhances their maturation but also induces their apoptosis (11, 12). There are studies demonstrating paradoxical effects of TGF-β1 on osteocalst differentiation, in concordance to its concentration. Lower levels of TGF-β1 promote differentiation while higher doses restrain differentiation of osteoclasts (13, 14).
More than a few studies tried to clarify the role of TGF-β1 in the pathogenesis of osteoporosis. In a study conducted by Akinci et al. (15) on males with osteoporosis, they demonstrated that TGF-β1 level is decreased in the sera of these cases. In a rat model, Estai et al. (5) suggested that treatment with estrogen improved the strength of the bone in estrogen-deficient rats by inducing the expression of TGF-β1. In another study by Kim et al. (16), it was implied that TGF-β1 positively inhibits bone resorption in rats. In contrary to these studies, Georgescu et al. (17) stated that there is no evidence for a major role of TGF-β1 as a determinant of bone mass in osteoporotic males or postmenopausal females.
Interleukin 18 (IL-18) is another molecule, the impacts of which on the osteoclasts need more investigation. There are studies reporting that IL-18 inhibits osteoclastic formation and bone resorptive activity (18, 19), while others demonstrate that IL-18 can stimulate osteoclast formation indirectly (20, 21).
2. Objectives
Transforming growth factor-β1 and IL-18 play complex roles in normal bone metabolism, and in pathophysiology of postmenopausal osteoporosis. The major goal of this study was to compare the TGF-β1 and IL-18 levels in the sera of postmenopausal females with and without osteoporosis. Besides, we tried to demonstrate if there is any association between the level of these molecules in the serum and the clinicopathological features of postmenopausal osteoporotic females, such as age, body mass index (BMI), parity, smoking and vitamin D supplementation.
3. Methods
3.1. Study Design, Participants and Data Collection
An analytic cross sectional study was conducted in order to further clarify the role of TGF-β1 and IL-18 in osteoporosis of postmenopausal females. The study included 65 postmenopausal females with osteoporosis as cases and 69 postmenopausal females of similar age without osteoporosis as controls. The cases and controls were matched for age and BMI. Participants were recruited from the gynecology department of a hospital affiliated with Shiraz University of Medical Science, and were included in the study if they had had amenorrhea for at least one year.
Dual energy X-ray absorptiometry (DXA) (Lunar Corporation, Madison, Wisconsin, USA) of lumbar spine and femoral neck was used to determine bone mass density (BMD) of participants and T-scoring was applied to establish whether the patient has osteoporosis or not. T-scoring determines the number of units (standard deviation) the bone density is below or above the average bone density of a 30-year-old healthy adult with the same gender (22). Patients were considered osteoporotic if their bone density was 2.5 SD or more below the young adult mean (T-score < -2.5) and participants with a T-score of -1 and above were considered to be normal in bone mass densitometry and were selected as controls. Osteopenic individuals (-2.5< T-score <-1) were not included in this study.
Demographics of the participants (including age, weight and height) and other variables (including age of menarche, age of menopause, parity, smoking, calcium and vitamin D consumption) were collected through a complete interview and physical examination and they are summarized in Table 1. Patients with a history of hysterectomy, unilateral/bilateral oophorectomy, atherosclerotic vascular diseases, diabetes mellitus, familial hyperlipidemia, severe and chronic systemic diseases, chronic hypertension, endocrine diseases and immune deficiency were excluded from the study. The patients with metabolic bone diseases or any secondary cause of osteoporosis such as thyroid and parathyroid diseases, malignancies, hypercortisolism (primary or secondary) and those on steroids, heparin and chemotherapy, were also excluded.
Baseline Characteristics of Osteoporotic and Non-Osteoporotic Participants
All individuals included in this study were informed and their consent to participate was taken in written form. Declaration of Helsinki was considered in this study and the research protocol was approved by the ethics committee of Shiraz University of Medical Science.
3.2. Preparation of Blood Sample and Assay for Transforming Growth Factor-β1 and Interleukin-18
Blood samples were drawn from 134 participants and were collected in sterile clean, dry tubes. Sera were rapidly separated after coagulation and were kept frozen at -70°C until time of assay for IL-18 and TGF-β1. Serum TGF-β1 and IL-18 level was measured by the commercial enzyme linked immunosorbent assay (ELISA) kit (R&D systems, Abingdon, UK), according to the manufacturers’ guidelines. The sensitivity of the assay for TGF-β1 was 15.4 pg/mL and for IL-18 was 25 pg/mL. In order to evaluate intra-assay precision, five serum samples of known concentration (R&D Systems’ Quantikine® kits, Abingdon, UK) were tested on one plate. The average of intra-assay coefficient of variability (CV) for TGF-β1 was 5.2% and for IL-18 was 5.7%. Five samples, of known concentration, were tested in separate assays to assess inter-assay precision, and average of high and low control for TGF-β1 and IL-18 was 6.1% and 5.8%, respectively.
3.3. Statistical Methods
We used statistical package for social science (SPSS) 17 for data analysis. In order to determine if the variables are normally distributed or not, we used the Shapiro-Wilk test. Data with normal distribution were presented as mean ± standard deviation (SD), and otherwise as median. Mann-Whitney U test and Kruskal-Wallis test was used to compare the difference between groups. Pearson correlation was utilized to correlate bivariates. Two-tailed P < 0.05 was considered statistically significant.
4. Results
A total of 134 post-menopausal females, who were referred to the department of gynecology of Shiraz University hospitals, participated in this study. Sixty-five of the participants were osteoporotic in bone mineral densitometry. Mean age of the participants was 53.5 ± 6.8 years and mean of menopause age was 47.5 ± 4.1 years. The mean BMI in both cases and control groups was 26.5 ± 4.0 kg/m2.
As the TGF-β1 and IL-18 levels in the sera of both cases and controls were not normally distributed (Shapiro-Wilk test, P = 0.00), Mann-Whitney U test was employed to compare the circulating levels of TGF-β1 and IL-18 between these two groups. Serum TGF-β1 levels were significantly higher in osteoporotic postmenopausal females than non-osteoporotic individuals (Figure 1; 23.8 vs. 15.8 ng/mL; P = 0.009).
Error Bar Presenting Serum TGF-β1 Levels Were Significantly Higher in Osteoporotic Patients (P < 0.05)
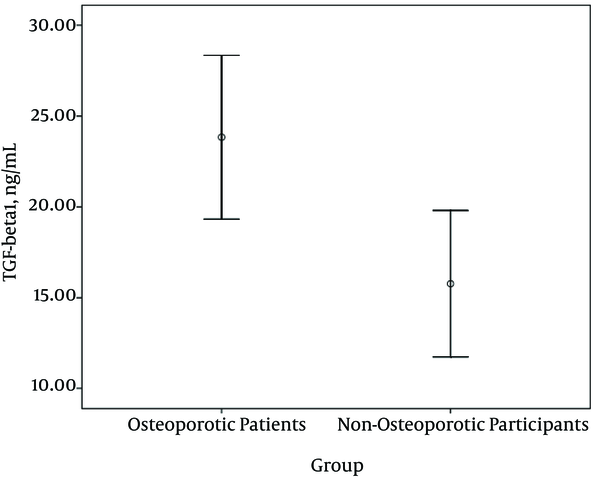
Receiver operating characteristic (ROC) curve analysis was established to further investigation of the accuracy of TGF-β1 levels to discriminate patients with osteoporosis from control subjects. Area under the curve for TGF-β1 was 0.71 (Figure 2; P = 0.04). This means on average, an osteoporotic postmenopausal female will have a higher circulating TGF-β1 level than 71% of the non-osteoporotic postmenopausal females.
Receiver Operating Characteristics (ROC) Curve for Serum Transforming Growth Factor-β1 Levels in Sera of Postmenopausal Females
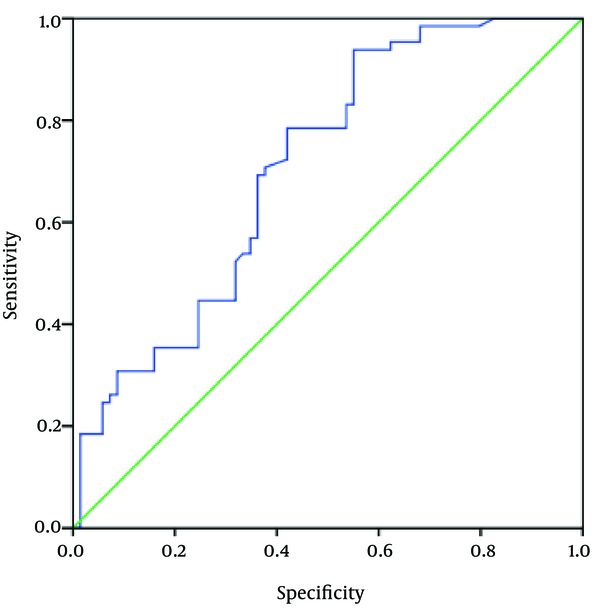
There was a positive correlation between TGF-β1 serum levels and BMI in osteoporotic postmenopausal females and the serum levels of TGF-β1 were significantly higher in participants with BMI > 25 kg/m2 (Table 2), 16.4 vs. 25.4 ng/mL; P = 0.04). No other statistically significant correlation was found between circulating TGF-β1 levels and age, age of menarche or menopause, parity, smoking, vitamin D and calcium consumption in both case and control groups.
Association of Serum Transforming Growth Factor β1 (TGF-β1) Levels With Different Clinical Variables in Osteoporotic Patients
Variable | No. (%) | Tgf-β1, (ng/mL)a | P Value |
---|---|---|---|
Smoker | 0.76b | ||
Yes | 22 (33.85) | 26.09 | |
No | 43 (66.15) | 22.70 | |
Calcium-vitamin D supplementation | 0.69b | ||
Yes | 20 (30.80) | 24.83 | |
No | 45 (69.20) | 23.38 | |
Body mass index (BMI), kg/m2 | 0.04b,c | ||
BMI < 25 | 11 (16.92) | 16.42 | |
BMI > 25 | 54 (83.08) | 25.35 | |
Parity | 0.43d | ||
0 | 7 (10.77) | 21.97 | |
1 | 3 (4.62) | 20.04 | |
2 | 10 (15.38) | 25.76 | |
> 3 | 45 (69.23) | 23.96 |
No statistically significant difference between IL-18 levels in the sera of cases and controls was found. Also we found no significant correlation between serum levels of IL-18 and TGF-β1, age of the participant, age of menopause or menarche, parity, tobacco use, parity and consumption of calcium and vitamin D supplements, in both groups. Neither IL-18 levels nor TGF-β1 levels were related to the severity of osteoporosis in the patient group.
5. Discussion
In this study, we demonstrated that TGF-β1 levels in the sera of postmenopausal osteoporotic females were significantly higher than non-osteoporotic postmenopausal controls. Our observation opposes the results of several studies that have reported TGF-β1 has a protective role against osteoporosis and aids bone healing (3-5, 7, 11, 22). However, there are several other studies consistent with our results. Cheng et al. (23) suggested that TGF-β1 levels increase during postmenopausal period and decrease during old age. Most of the osteoporotic patients in our study were in their early menopausal phases. Also, in a rodent study conducted by Ota et al. (24), it was demonstrated that TGF-β1 increases osteoclast production and cultures with suppressed TGF-β activation keep their capacity to raise mineralization.
The impact of immune system on bone formation and remodeling has led to considering osteoporosis as a chronic immune disease (25). In postmenopausal females, aging and estrogen deficiency are the major cause of decreased bone mineral density. Many estrogen-dependent growth factors and cytokines are involved in bone remodeling such as IL-1, IL-6, insulin-like growth factor I and II, colony stimulating factor, osteoprotegrin and TGF-β (26, 27). Transforming Growth Factor-β1 has been shown to interact with hormones and soluble factors, like estrogen and vitamin D and there are studies demonstrating that estrogen stimulates TGF-β1 production, and promotes osteoblast proliferation and maturation. Also, estrogen causes osteoclast apoptosis through a TGF-β-dependent pathway (28).
Balooch et al. (29) demonstrated that increased TGF-β signaling in genetically modified mouse models, causes decreased mineral component of the bone and weakened mechanical properties. In a rodent model of osteoarthritis, Zhen et al. (30) demonstrated that activated TGF-β1 is increased in subchondral bone, which later becomes osteoporotic. In human beings, high levels of active TGF-β1 cause several skeletal disorders as a result of abnormalities in bone remodeling. Camurati-Engelmann disease (CED) is caused by mutation in TGF-β1 gene, resulting in premature activation of this protein. In the histology bone samples of these patients, trabecular connectivity is decreased despite normal osteoblast and osteoclast numbers. This may be suggestive of uncoupled bone remodeling caused by hyperactive TGF-β1 in CED patients (31, 32).
Aging leads to dramatic bone loss, especially in females during the postmenopausal years and osteoporosis is the result of osteoclast bone resorption being more than osteoblast bone formation. Previously, we mentioned that osteoblasts originate from MSCs. With aging, MSCs also experience senescence and their proliferative capacity and consequently bone formation is decreased (33). As these cells age, they start secreting various factors, including TGF-β1 (34). Also during aging, the concentration of reactive oxygen species (ROS) increases in the bone microenvironment, decreasing osteoblast differentiation and increasing osteoclast activity and releasing more TGF-β1 (35, 36). Transforming Growth Factor-β1 is the second important chemoattractant molecule for osteoclasts and monocytes after M-CSF (12), and bone resorption is accompanied by higher levels of circulating TGF-β1 (37).
Transforming growth factor-β1 released by osteoclasts induces the migration of MSCs to bone resorption sites for osteoblast differentiation and bone formation. However, as the MSCs are old and their capacity to proliferate and differentiate is decreased, elevated TGF-β1 levels leads to recruitment and activation of more osteoclasts and bone resoption outmatches bone formation, which leads to osteoporosis (4).
There was no difference between IL-18 levels in the sera of osteoporotic and non-osteoporotic postmenopausal females in this study. Although in a study conducted by Morita et al. (20), it was demonstrated that IL-18 could inhibit tumor necrotizing factor (TNF)-α induced osteoclastogenesis in mice. In contrary, Dai et al. (21) stated that IL-18 can indirectly stimulate osteoclast formation by up-regulating RANK Ligand production in T-cells of rheumatoid arthritis patients’ synovium. In another study, Maugeri et al. (38) showed that 12 months after treating post-menopausal osteoporotic females with bisphosphonates, BMD and circulating IL-18 were increased. Even though our survey involved relatively small groups, it did not allow us to state that IL-18 should not be considered as a marker of bone resorption, and further investigations are needed. In our study, age, age of menopause and menarche, parity, consumption of calcium and vitamin D had no impact on serum levels of TGF- β1 and IL-18.
This study design did not allow us to derive an etiologic conclusion that the observed higher level of TGF-β1 in osteoporotic females is the cause of bone resorption. We selected our participants from a population of patients referred to the gynecology department, since cases and controls were not derived from a prospective cohort study and nor from the general population thus we should consider this limitation in future studies to better understand the role of TGF-β in pathogenesis of osteoporosis. Our participants were in early postmenopausal ages thus a follow up study with a larger sample size will allow us to better elucidate the role of TGF-β in a time trend analysis.
In conclusion, our study demonstrated that TGF-β1 serum levels is higher in osteoporotic postmenopausal females than non-osteoporotic ones, and probably aberrant increase in TGF-β1 in postmenopausal females can result in uncoupled bone resorption and formation, which leads to osteoporosis. Additional investigations are needed to further clarify the role of TGF-β1 in postmenopausal osteoporosis.
Acknowledgements
References
-
1.
Sun J, Zhang C, Xu L, Yang M, Yang H. The transforming growth factor-beta1 (TGF-beta1) gene polymorphisms (TGF-beta1 T869C and TGF-beta1 T29C) and susceptibility to postmenopausal osteoporosis: a meta-analysis. Medicine (Baltimore). 2015;94(4):461. [PubMed ID: 25634187]. https://doi.org/10.1097/MD.0000000000000461.
-
2.
Mirza F, Canalis E. Management of endocrine disease: Secondary osteoporosis: pathophysiology and management. Eur J Endocrinol. 2015;173(3):131-51. [PubMed ID: 25971649]. https://doi.org/10.1530/EJE-15-0118.
-
3.
Chen G, Deng C, Li YP. TGF-beta and BMP signaling in osteoblast differentiation and bone formation. Int J Biol Sci. 2012;8(2):272-88. [PubMed ID: 22298955]. https://doi.org/10.7150/ijbs.2929.
-
4.
Crane JL, Cao X. Bone marrow mesenchymal stem cells and TGF-beta signaling in bone remodeling. J Clin Invest. 2014;124(2):466-72. [PubMed ID: 24487640]. https://doi.org/10.1172/JCI70050.
-
5.
Estai MA, Suhaimi F, Das S, Shuid AN, Mohamed Z, Soelaiman IN. Expression of TGF-beta1 in the blood during fracture repair in an estrogen-deficient rat model. Clinics (Sao Paulo). 2011;66(12):2113-9. [PubMed ID: 22189738].
-
6.
Poniatowski LA, Wojdasiewicz P, Gasik R, Szukiewicz D. Transforming growth factor Beta family: insight into the role of growth factors in regulation of fracture healing biology and potential clinical applications. Mediators Inflamm. 2015;2015:137823. [PubMed ID: 25709154]. https://doi.org/10.1155/2015/137823.
-
7.
Kasagi S, Chen W. TGF-beta1 on osteoimmunology and the bone component cells. Cell Biosci. 2013;3(1):4. [PubMed ID: 23321200]. https://doi.org/10.1186/2045-3701-3-4.
-
8.
Jilka RL, Weinstein RS, Bellido T, Parfitt AM, Manolagas SC. Osteoblast programmed cell death (apoptosis): modulation by growth factors and cytokines. J Bone Miner Res. 1998;13(5):793-802. [PubMed ID: 9610743]. https://doi.org/10.1359/jbmr.1998.13.5.793.
-
9.
Maeda S, Hayashi M, Komiya S, Imamura T, Miyazono K. Endogenous TGF-beta signaling suppresses maturation of osteoblastic mesenchymal cells. EMBO J. 2004;23(3):552-63. [PubMed ID: 14749725]. https://doi.org/10.1038/sj.emboj.7600067.
-
10.
Spinella-Jaegle S, Roman-Roman S, Faucheu C, Dunn FW, Kawai S, Gallea S, et al. Opposite effects of bone morphogenetic protein-2 and transforming growth factor-beta1 on osteoblast differentiation. Bone. 2001;29(4):323-30. [PubMed ID: 11595614].
-
11.
Murakami T, Yamamoto M, Ono K, Nishikawa M, Nagata N, Motoyoshi K, et al. Transforming growth factor-beta1 increases mRNA levels of osteoclastogenesis inhibitory factor in osteoblastic/stromal cells and inhibits the survival of murine osteoclast-like cells. Biochem Biophys Res Commun. 1998;252(3):747-52. [PubMed ID: 9837778]. https://doi.org/10.1006/bbrc.1998.9723.
-
12.
Pilkington MF, Sims SM, Dixon SJ. Transforming growth factor-beta induces osteoclast ruffling and chemotaxis: potential role in osteoclast recruitment. J Bone Miner Res. 2001;16(7):1237-47. [PubMed ID: 11450699]. https://doi.org/10.1359/jbmr.2001.16.7.1237.
-
13.
Ota K, Quint P, Weivoda MM, Ruan M, Pederson L, Westendorf JJ, et al. Transforming growth factor beta 1 induces CXCL16 and leukemia inhibitory factor expression in osteoclasts to modulate migration of osteoblast progenitors. Bone. 2013;57(1):68-75. [PubMed ID: 23891907]. https://doi.org/10.1016/j.bone.2013.07.023.
-
14.
Karst M, Gorny G, Galvin RJ, Oursler MJ. Roles of stromal cell RANKL, OPG, and M-CSF expression in biphasic TGF-beta regulation of osteoclast differentiation. J Cell Physiol. 2004;200(1):99-106. [PubMed ID: 15137062]. https://doi.org/10.1002/jcp.20036.
-
15.
Akinci B, Bayraktar F, Saklamaz A, Demir T, Yener S, Comlekci A, et al. Low transforming growth factor-beta1 serum levels in idiopathic male osteoporosis. J Endocrinol Invest. 2007;30(5):350-5. [PubMed ID: 17598964].
-
16.
Kim CH, Kim YH, Kim YK, Kang BS, Lee TK, Moon SH, et al. IL-1beta induces and TGF-beta reduces vitamin D3-induced bone resorption in mouse calvarial bone cells. Immunol Invest. 2003;32(3):171-86. [PubMed ID: 12916707].
-
17.
Georgescu C, Seck T, Diel I, Minne H, Duncea I, Pfeilschifter J. Bone matrix insulin-like growth factor (IGF)-I, IGF-II and transforming growth factor (TGF)-beta1 levels in men and postmenopausal women with osteoporosis: lack of association with circulating growth factors and bone mineral density. Rev Med Chir Soc Med Nat Iasi. 2004;108(2):281-6. [PubMed ID: 15688799].
-
18.
Horwood NJ, Elliott J, Martin TJ, Gillespie MT. IL-12 alone and in synergy with IL-18 inhibits osteoclast formation in vitro. J Immunol. 2001;166(8):4915-21. [PubMed ID: 11290769].
-
19.
Yamada N, Niwa S, Tsujimura T, Iwasaki T, Sugihara A, Futani H, et al. Interleukin-18 and interleukin-12 synergistically inhibit osteoclastic bone-resorbing activity. Bone. 2002;30(6):901-8. [PubMed ID: 12052461].
-
20.
Morita Y, Kitaura H, Yoshimatsu M, Fujimura Y, Kohara H, Eguchi T, et al. IL-18 inhibits TNF-alpha-induced osteoclastogenesis possibly via a T cell-independent mechanism in synergy with IL-12 in vivo. Calcif Tissue Int. 2010;86(3):242-8. [PubMed ID: 20111957]. https://doi.org/10.1007/s00223-010-9335-6.
-
21.
Dai SM, Nishioka K, Yudoh K. Interleukin (IL) 18 stimulates osteoclast formation through synovial T cells in rheumatoid arthritis: comparison with IL1 beta and tumour necrosis factor alpha. Ann Rheum Dis. 2004;63(11):1379-86. [PubMed ID: 15479886]. https://doi.org/10.1136/ard.2003.018481.
-
22.
WHO scientific group on the assessment of osteoporosis at primary health care level. Brussels: World Health Organization; 2004. Available from: www.who.int.
-
23.
Cheng Q, Tang W, Sheu TJ, Du Y, Gan J, Li H, et al. Circulating TGF-beta1 levels are negatively correlated with sclerostin levels in early postmenopausal women. Clin Chim Acta. 2016;455:87-92. [PubMed ID: 26826396]. https://doi.org/10.1016/j.cca.2016.01.025.
-
24.
Ota K, Quint P, Ruan M, Pederson L, Westendorf JJ, Khosla S, et al. TGF-beta induces Wnt10b in osteoclasts from female mice to enhance coupling to osteoblasts. Endocrinology. 2013;154(10):3745-52. [PubMed ID: 23861379]. https://doi.org/10.1210/en.2013-1272.
-
25.
Charles JF, Nakamura MC. Bone and the innate immune system. Curr Osteoporos Rep. 2014;12(1):1-8. [PubMed ID: 24500569]. https://doi.org/10.1007/s11914-014-0195-2.
-
26.
Hofbauer LC, Khosla S, Dunstan CR, Lacey DL, Boyle WJ, Riggs BL. The roles of osteoprotegerin and osteoprotegerin ligand in the paracrine regulation of bone resorption. J Bone Miner Res. 2000;15(1):2-12. [PubMed ID: 10646108]. https://doi.org/10.1359/jbmr.2000.15.1.2.
-
27.
McLean RR. Proinflammatory cytokines and osteoporosis. Curr Osteoporos Rep. 2009;7(4):134-9. [PubMed ID: 19968917].
-
28.
Hughes DE, Dai A, Tiffee JC, Li HH, Mundy GR, Boyce BF. Estrogen promotes apoptosis of murine osteoclasts mediated by TGF-beta. Nat Med. 1996;2(10):1132-6. [PubMed ID: 8837613].
-
29.
Balooch G, Balooch M, Nalla RK, Schilling S, Filvaroff EH, Marshall GW, et al. TGF-beta regulates the mechanical properties and composition of bone matrix. Proc Natl Acad Sci U S A. 2005;102(52):18813-8. [PubMed ID: 16354837]. https://doi.org/10.1073/pnas.0507417102.
-
30.
Zhen G, Wen C, Jia X, Li Y, Crane JL, Mears SC, et al. Inhibition of TGF-beta signaling in mesenchymal stem cells of subchondral bone attenuates osteoarthritis. Nat Med. 2013;19(6):704-12. [PubMed ID: 23685840]. https://doi.org/10.1038/nm.3143.
-
31.
Whyte MP, Totty WG, Novack DV, Zhang X, Wenkert D, Mumm S. Camurati-Engelmann disease: unique variant featuring a novel mutation in TGFbeta1 encoding transforming growth factor beta 1 and a missense change in TNFSF11 encoding RANK ligand. J Bone Miner Res. 2011;26(5):920-33. [PubMed ID: 21541994]. https://doi.org/10.1002/jbmr.283.
-
32.
Bondestam J, Mayranpaa MK, Ikegawa S, Marttinen E, Kroger H, Makitie O. Bone biopsy and densitometry findings in a child with Camurati-Engelmann disease. Clin Rheumatol. 2007;26(10):1773-7. [PubMed ID: 17206397]. https://doi.org/10.1007/s10067-006-0511-z.
-
33.
Zhou S, Greenberger JS, Epperly MW, Goff JP, Adler C, Leboff MS, et al. Age-related intrinsic changes in human bone-marrow-derived mesenchymal stem cells and their differentiation to osteoblasts. Aging Cell. 2008;7(3):335-43. [PubMed ID: 18248663]. https://doi.org/10.1111/j.1474-9726.2008.00377.x.
-
34.
Kuilman T, Peeper DS. Senescence-messaging secretome: SMS-ing cellular stress. Nat Rev Cancer. 2009;9(2):81-94. [PubMed ID: 19132009]. https://doi.org/10.1038/nrc2560.
-
35.
Manolagas SC. From estrogen-centric to aging and oxidative stress: a revised perspective of the pathogenesis of osteoporosis. Endocr Rev. 2010;31(3):266-300. [PubMed ID: 20051526]. https://doi.org/10.1210/er.2009-0024.
-
36.
Almeida M, Han L, Martin-Millan M, Plotkin LI, Stewart SA, Roberson PK, et al. Skeletal involution by age-associated oxidative stress and its acceleration by loss of sex steroids. J Biol Chem. 2007;282(37):27285-97. [PubMed ID: 17623659]. https://doi.org/10.1074/jbc.M702810200.
-
37.
Tang Y, Wu X, Lei W, Pang L, Wan C, Shi Z, et al. TGF-beta1-induced migration of bone mesenchymal stem cells couples bone resorption with formation. Nat Med. 2009;15(7):757-65. [PubMed ID: 19584867]. https://doi.org/10.1038/nm.1979.
-
38.
Maugeri D, Mamazza C, Lo Giudice F, Puglisi N, Muscoso EG, Rizzotto M, et al. Interleukin-18 (IL-18) and matrix metalloproteinase-9 (MMP-9) in post-menopausal osteoporosis. Arch Gerontol Geriatr. 2005;40(3):299-305. [PubMed ID: 15814163]. https://doi.org/10.1016/j.archger.2004.10.001.