Abstract
Background:
Although growth hormone (GH) has essential roles in the growth of animals, it has no growth-promoting effect during infancy period. The molecular mechanism underlying lack of growth-promoting effect of GH during infancy period remains unclear. Important signaling pathways are mediated by GH, including Janus kinase 2 (JAK2), extracellular signal-regulated kinase 1/2 (ERK1/2), signal transducers, and activators of transcription 5, 3, and 1 (STATs 5, 3 and 1).Objectives:
This study explored the underlying molecular mechanisms driving to the lack of growth-promoting effect of GH in the early stage of life by in vivo assessment of intracellular signal response (STAT5/ 3/ 1, JAK2 and ERK1/ 2) to GH at different physiological stages.Methods:
In this study, five age groups of rats (1-, 4-day-old, and 1-, 2-, 3-week-old) were selected. The rats were anesthetized using pentobarbital (100 mg/kg) and then received the rat GH (2mg/kg) via inferior vena cava injection. The control rats were injected with normal saline during the same period. The intracellular signal response to GH was assessed by Western blot analysis.Results:
JAK2 and STAT5 were expressed in 1-day and 4-day-old newborn rats and their expression levels were comparable with the levels of the 1-, 2-, and 3-week-old rats; however, JAK2/STAT5 phosphorylation was not observed in 1-day-old and 4-day-old newborn rats after stimulation with GH in the liver. Similar to JAK2 and STAT5, we did not detect STAT3/1 activation during infancy stages although basic STAT3 and STAT1 were also expressed in hepatocytes from newborn rats. In addition we detected ERK1/2 activation in 4-day-old, 1-, 2-, and 3-week-old rats but not in 1-day-old rats.Conclusions:
JAK2, STAT5, STAT3, STAT1, and ERK1/2 were not simultaneously activated by GH in newborn rats; this finding may be one of the underlying mechanism of GH insensitivity in newborn rats.Keywords
Growth Hormone Growth-Promoting New-Born Rats Signaling Pathways
1. Background
Growth hormone (GH) has essential biological activities and physiological functions (1-5). A series of studies showed that GH has more than 300 physiological functions (6). Growth hormone receptor (GHR) is widely distributed in the body and more abundant in the liver (7). When GH binds to the GHR expressed on the cell membranes, GHR itself and the intracellular GHR-associated Janus kinase2 (JAK2) are activated, after which, several down-stream intracellular signaling molecules, such as signal transducers and activators of transcription (STAT) are phosphorylated. These phosphorylated signaling molecules form dimers and then transfer to the nucleus, where they perform their physiological functions (8-12).
GH plays an important role in the growth of animals. Liu et al. reported that GH regulation modulating animal growth and development is phase-dependent (13). It was reported that the postnatal growth of rodents shows two phases. The first phase lasts up to two weeks postpartum and is GH-independent, whereas the second phase occurs during the third week and is GH-dependent (14, 15). In addition, mammalian (such as pigs) growth and development modulated by GH is presented in two phases. One phase is during babyhood (from two days to five weeks) and is GH-insensitive. Another phase is pubertal (after five weeks) and is GH-sensitive (16). However, until now, the reason that GH has no role in the growth and development of newborn animals remains unclear. There are several hypotheses about this phenomenon. First, the newborns' GHR content is lower than it is in adult animals, which may provide an explanation for GH-insensitivity in newborns (17-19). Furthermore, Martinez et al. showed that GH did not activate STAT5 in newborn mice, indicating that mice are insensitive to GH during this phase (20). In addition, Ocaranza et al. also found that GH did not trigger STAT5 and JAK2 in skin fibroblasts from infants compared to prepubertal boys (21). However, these studies only evaluated one signaling pathway, STAT5, and it remains unclear whether other pathways are responsive to GH.
In summary, GH functions are very extensive, since GHR is expressed in almost all tissues and it has been reported that GH has more than 300 functions and roles (6). However, until now, the reason why GH has no function in promoting the growth and development of newborn animals remains unclear. Therefore, it is very significant to explore the reason why GH cannot exhibit its bioactivities in infancy period, which is helpful to further understand the mechanisms of physiological action of GH.
2. Objectives
The mechanism by which GH functions to promote the growth and development in newborn animals remains elusive. We postulated that it is related to variations in the signaling response to GH during the somatic growth in rats because the functions of GH are mediated primarily by intracellular signaling molecules. The liver is the major target organ of GH (22). The current study was designed to evaluate the activation of JAK2, ERK1/2, STAT5, STAT3, and STAT1 in the liver of rats at different time points following GH stimulation. We found that newborn rats lacked an intracellular signaling response to GH, which might explain why GH has no impact on the postnatal growth and development.
3. Methods
3.1. Antibodies and Reagents
Rat GH, HRP-conjugated goat anti-mouse, and anti-rabbit antibodies were obtained from Sigma (USA). Total STAT1 and phospho-STAT1 (Tyr701), total STAT3 and phospho-STAT3 (Tyr705), total STAT5 and phospho-STAT5 (Tyr694), total ERK1/2 and phospho-ERK1/2 (Thr202/Tyr204), and total JAK2 and phospho-JAK2 (Tyr1007/1008) were obtained from Cell Signaling Technology (USA). The enhanced chemiluminescence (ECL) and Difco skim milk were purchased from Pierce (USA). Polyvinylidene fluoride (PVDF) membranes were obtained from Millipore (Bedford, MA, USA). The above-mentioned antibodies and reagents were used for Western blot analysis. Anti-GHR antibody and Fluorescein isothiocyanate (FITC) were obtained from Sigma (USA) and used for indirect immunofluorescence assay. Unless otherwise stated, other reagents were purchased from Sigma-Aldrich (USA). The above-mentioned reagents were used for cell culture.
3.2. Animals
Healthy male Sprague-Dawley (SD) rats (in the same batch) at the ages of one, four, seven, 14, and 21 days were selected randomly. They were purchased from the Animal Experiment Center at Jilin University. The average weights of these rats were eight, 14, 23, 40, and 55 g, respectively. The rats were placed in stable conditions with a 12-h light and 12-h dark cycle photoperiod. Additionally, they were given free access to water and a nutritionally balanced diet. The rats were divided into five ages (n = 6/group): 1-day-old, 4-day-old, 1-week-old, 2-week-old, and 3-week-old. Treatment groups received rat GH (n=3/group) and control groups were injected with normal saline (n = 3/group). The research was conducted in accordance with the guidelines of the Review Committee for the Use of Animal Subjects of Jilin Agricultural University.
3.3. Isolation and Culture of Primary Rat Hepatocytes
The rats were anesthetized using pentobarbital (100 mg/kg). The abdominal cavity was opened, the livers were fully exposed, and the inferior vena cava and hepatic portal vein were exposed. The livers were perfused according to our previous methods with slight modifications (5, 23). First, D-Hanks’ buffer was infused into the inferior vena cava for 10 min and the hepatic portal vein was cut open. Then, Hanks’ buffer containing 0.025% (w/v) IV collagenase was perfused for 10 min. The livers were excised, removed to a culture dish, minced, and passed through a 70-micron sterile nylon mesh. The resulting cell suspensions were centrifuged and the precipitate was resuspended in the culture medium. The viability of rat hepatocytes was assessed as approximately 85% by trypan blue dye exclusion. The cells were cultured with the maintenance medium (penicillin, streptomycin, and DMEM with 1% FBS) for the experiment.
3.4. Tissue Collection
The rats of different ages (1-, 4-day-old, and 1-, 2- and 3-week-old) (n = 6/group) were anaesthetized using pentobarbital (100 mg/kg) (5, 11) and then received 2 mg rat GH per kilogram (20, 24, 25) of body weight by inferior vena cava injection (n = 3/group) between the hours of 8 o’clock and 11 o’clock in the morning (24). The control groups were injected with normal saline during the same period (n = 3/group). The rats were sacrificed by exsanguination via cardiac puncture 25 min after the injections, and the livers were removed and placed into liquid nitrogen for 1 or 2 min and then stored at -80 °C.
3.5. Protein Extraction
The processes of protein extraction referred to our previous methods (12). Briefly, a total of 100 mg tissue was ground in liquid nitrogen. The tissue was disrupted in 0.5 - 1 ml precooled cell lysis buffer (RIPA kit purchased from Pierce) (with 1000 µl phosphatase inhibitors, 100 µl proteinase inhibitor, and 1000 µl PMSF per 100 ml lysis buffer). The samples were shaken at 4 °C for 10 - 15 min and centrifuged at 10,000 rpm and 4 °C for 5 min. The protein level was measured using the Coomassie brilliant blue method. According to the protein level, 80 µg of total protein was mixed with loading buffer (5X). They were heated to 100 °C for 5 min for protein denaturation and stored at -20 °C until immunoblotting.
3.6. Western Blotting
A total of 80 μg total protein was loaded onto 4% to 10% SDS-PAGE gels. Then, the protein samples were transferred to PVDF membranes (0.22 μm). The membranes were incubated with 5% skim milk for 1 h at 37 °C. The membranes were then washed three times for 10 min each in TBST buffer (0.1% Tween 20, 0.1 M NaCl, 10 mM Tris-HCl, pH 7.2 - 7.4). After washing, the membranes were incubated overnight at 4 °C with phospho- or total-STAT1, STAT3, JAK2, phospho-STAT5a/b, ERK1/2, total-STAT5a/b, or ERK1/2 antibodies according to the manufacturer’s specifications. In the next day, the membranes were incubated with HRP-conjugated secondary antibody at 37 °C for 1 h after three washes for 10 min each in TBST buffer. Immunoreactive proteins on the membranes were detected using the ECL detection system.
The membranes were stripped for 30 min at 37 °C by incubation in stripping buffer for reblotting. The blots were washed, then reblotted, and immunolabelled as described above.
3.7. Densitometric Analysis
The densitometric analysis of the immunoreactive protein bands was performed using Quantity One® software (developed by BioRad Technical Service Department, USA; LSG. TechServ. US@BioRad.com).
3.8. Indirect Immunofluorescence Assay
GHR expression in hepatocytes from newborn rats was evaluated by indirect immunofluorescence assay with slight modifications (26). Briefly, the primary rat hepatocytes were fixed with 4% paraformaldehyde and permeabilized with Trixon-100. Then, the hepatocytes were blocked with 3% BSA for 1 h. After washing the cells with PBS three times, they were incubated with anti-GHR antibody. Subsequently, the cells were washed and treated with FITC-conjugated secondary antibody at 37 °C for 1h in the dark. After washing, the cell nuclei were stained with Propidium Iodide (PI) (red) for 15 min at room temperature. The cells were observed by a laser scanning confocal microscope (CLSM, Olympus FV1000).
3.9. Statistical Analysis
Data are presented as mean values ± standard error (S.E.). Statistical analysis was performed using independent-sample T test with the Statistical Analysis System (SAS) software (SAS version 9.0, Institute Inc., Cary, NC, USA). P < 0.05 was considered statistically significant.
4. Results
4.1. GHR Expression on Hepatocytes from Newborn Rats
First, we evaluated GHR expression on hepatocytes obtained from newborn rats after stimulation with GH, as shown in Figure 1. We observed GHR expression on hepatocytes from newborn rats, which was mainly localized in the cell membranes and cytoplasm. The control antibody showed no positive staining (data not shown).
Evaluation of GHR expression on hepatocytes obtained from new-born rats
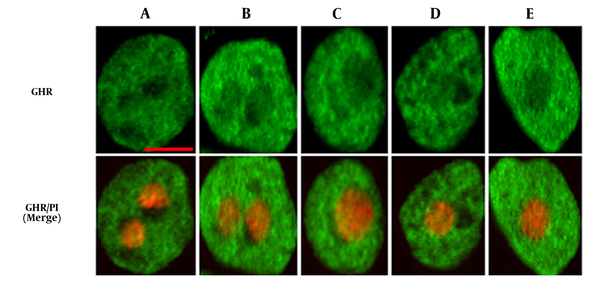
4.2. Basal Expression Level of JAK2, STAT5/3/1, and ERK1/2
To assess the basal expression level of intracellular signaling proteins (JAK2, STAT5/3/1, and ERK1/2) at each age point, the Western blot analyses were performed. We determined that the basal expression levels of JAK2, STAT5/3/1, and ERK1/2 were similar in all groups.
4.3. JAK2 Phosphorylation
We detected the phosphorylation of the intracellular signaling protein JAK2 in postnatal rats between one day and three weeks of age after GH stimulation. JAK2 phosphorylation in newborns (Figure 2) showed that the ratio of phospho-JAK2/JAK2 was similar within the first week, both in the absence or presence of GH. However, at one week, two weeks and three weeks of age, we evaluated JAK2 activation and found that the phosphorylation level of JAK2 was maximal in 3-week-old rats.
Activation of JAK2 in postnatal rats at 1-day, 4-days, 1- week, 2-weeks and 3-weeks
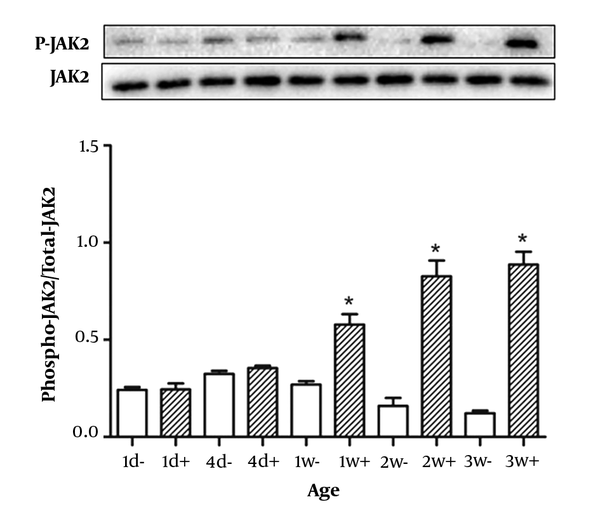
4.4. STAT5/3/1 Phosphorylation
We assessed the phosphorylation of the intracellular signaling protein STAT5 in postnatal rats between one day and three weeks of age. As indicated in Figure 3A, we found that STAT5 was activated in the 1-, 2-, and 3-week-old rats. It was highly significantly different compared to the negative controls. Furthermore, the response of STAT5 protein activation was not observed within the first week in the newborn rats under the same cell culture conditions, which suggests that STAT5 has no response in rats within the first week.
Abundance of phosphorylated proteins in livers of different age group rats (1-day-old, 4-day-old, 1-week-old, 2-week-old and 3-week-old) in response to GH
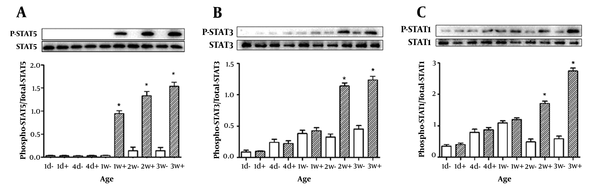
As shown in Figure 3B, no differences were found for STAT3 phosphorylation in 1-day, 4-days, and 1-week newborn rats, which suggests that STAT3 has no response in rats during the second week. It also suggests that STAT3 has no response in rats during the second week after birth. In addition, phospho-STAT3 was observed in 2- and 3-week-old rats, which indicates that the signaling response has occurred.
Similar to STAT3, the GH-induced phosphorylation of STAT1 could not be detected within the second week of newborn rats, which suggests that STAT1 also has no response within the second week. However, we detected phospho-STAT1 in 2-week-old and 3-week-old rats, as shown in Figure 3C. The expression level of phosphorylated STAT1 was maximal at three weeks of age.
4.5. ERK1/2 Activation
We could detect the activation of ERK1/2 in 4-day, 1-week, 2-week and 3-week rats by Western blot analysis (Figure 4). Furthermore, we did not detect ERK1/2 phosphorylation by the fourth day, which suggests that ERK1/2 has no response in the fourth day after birth. This result indicates that the ERK1/2 signal was responsive to GH four days after birth.
The ERK1/2 protein could not be activated in postnatal rats at 1-day but was activated in 4-day, 1-week, 2-weeks and 3- weeks rats
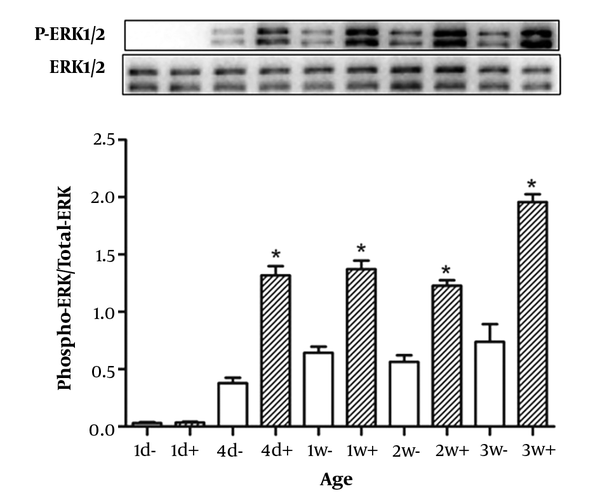
5. Discussion
GH plays an important role in the animal somatic growth and development. However, current information indicates that the postnatal physiological functions exhibited by GH show phase-dependent properties. Postnatal rodents, such as mice, exhibit two phases of rapid growth and development. The first and the most rapid phase is perinatal, which is GH-independent. Moreover, after the first phase, the second phase occurs that is GH-dependent. Subsequently, the mice enter a “plateau of growth” with low-speed growth. Researchers have long-term studies in this phenomenon, but until now, it has remained unclear. Therefore, we explored why newborn rats are not responsive to GH from the perspective of intracellular signaling pathways.
In previous studies, researchers showed that GH has no growth-promoting effect on somatic growth during the early postnatal period due to low GHR expression or immature GHR forms (17). However, it was reported that GHR is expressed in newborn rats (27) and in the rat fetus (28, 29). In this study, we evaluated GHR expression on hepatocytes and found that it has no significant difference in each growth period of newborn rats. Thus, it may not be the main reason for GH insensitivity in newborn rats. We postulated that the variations in GH sensitivity during somatic growth of rats are also associated with the changes of the other important signaling protein molecules, such as STAT1/3, JAK2, and ERK1/2. Therefore, we used newborn rats as animal models and focused on the activation of intracellular signaling proteins in addition to STAT5.
In this work, we assessed the content of intracellular signaling proteins. Basal STAT3 and STAT1 were expressed during the second week in newborn rats. By contrast, STAT3/STAT1 phosphorylation was only detected in 2-week-old and 3-week-old rats after 20 min of rGH stimulation in hepatocytes (Figures 3B and 3C). It suggests that phospho-STAT3/1 signaling proteins were not detected in 1-, 4-day, and 1-week-old rats, reflecting that the reason for the GH-independent growth in newborn rats is that STAT3 and STAT1 could not be triggered by GH in the liver. In addition, it is well known that suppressor of cytokine signaling (SOCS) family and protein tyrosine phosphatases (PTPs) are involved in negatively regulating GH signaling. Recent studies have shown that Cytokine-induced suppressor (CIS, a membrane of SOCS family) and PTPs were presented with higher levels before one week of age, which declined thereafter (20, 30-35). Therefore, the reason STAT3 and STAT1 could not be triggered by GH in the first few postnatal days maybe due to the high levels of CIS and PTPs. Of course, we cannot rule out other unknown factors.
JAK2 plays key roles in GH signal transduction and GH-induced activation of JAK2 could recruit a variety of signaling proteins (36). The basal JAK2 expressions were detectable in 1-day and 4-day-old newborn rats and were comparable with the 1-, 2-, and 3-week-old rats. Nevertheless, the pattern of JAK2 phosphorylation was different: no JAK2 phosphorylation was observed in 1-day-old and 4-day-old newborn rats (Figure 2). This implies that downstream signaling proteins were not activated in rats during the first postnatal week. In addition, we observed increasing STAT5 activation in 1-, 2-, and 3-week-old rats but not in 1- or 4-day-old rats after stimulation with rat GH (Figure 3A). These results suggest that the JAK2-STAT5 signaling pathway was not activated during the first week, which is consistent with a recent study by Carolina S. Martinez (20).
In addition, we detected the intracellular signaling proteins ERK1/2 phosphorylation in 4-day-old and 1-, 2-, 3-week-old rats, but it was not detected in 1-day-old rats (Figure 4). ERK1/2 signaling cascade is activated by a wide variety of receptors involved in growth and differentiation including receptor tyrosine kinases (RTKs), integrins, and ion channels (37, 38). In the present study, we did not detect ERK1/2 phosphorylation in 1-day-old rats, but it was detected in 4-day-old and 1-, 2-, and 3-week-old rats (Figure 4), indicating that ERK1/2 signaling was not activated by GH in 1-day-old rats. Although ERK1/2 phosphorylation was detected at 4 days of age, it may not be induced specifically by GH. It remains possible that ERK1/2 was activated by another stimulating factor at this time point; indeed, we observed that ERK1/2 could be activated in the absence of GH stimulation (data not shown).
In this work, we observed that most of the growth hormone signaling molecules did not respond to GH in the first few postnatal days, reflecting that GH might have no function in growth promotion in this period of life. Additionally, the pattern of JAK2, STAT3, STAT1, and ERK1/2 phosphorylation exhibited an upward trend with age. The reason for the possible lack of growth hormone effect in the liver hepatocyte in the early life period could be explained by the following: 1) the growth-promoting effect of GH is tissue-specific and is associated with physiological stage (22, 39); 2) the phosphorylation level of the intracellular signaling protein is lower during the first few postnatal days than during the second and third weeks of age, which may lead to the inability of GH to promote growth in early life.
In conclusion, according to the present study, the GH signaling molecules, such as JAK2, STAT3, STAT1, and ERK1/2, were not activated by GH in the liver hepatocytes during the newborn period, indicating that newborn rats hepatocytes are not responsive to GH. It may provide an explanation for why GH has no role in postnatal growth and development. However, the reason that GH cannot activate JAK2, STAT3/1, and ERK1/2 is not fully understood in newborn rats and further studies are necessary, which is also the direction of our next work.
Acknowledgements
References
-
1.
Chia DJ. Minireview: mechanisms of growth hormone-mediated gene regulation. Mol Endocrinol. 2014;28(7):1012-25. [PubMed ID: 24825400]. [PubMed Central ID: PMC4075164]. https://doi.org/10.1210/me.2014-1099.
-
2.
Murphy WJ, Rui H, Longo DL. Effects of growth hormone and prolactin immune development and function[J]. Life Sci. 1995;57(1):1-14.
-
3.
Vijayakumar A, Yakar S, Leroith D. The intricate role of growth hormone in metabolism. Front Endocrinol (Lausanne). 2011;2:32. [PubMed ID: 22654802]. [PubMed Central ID: PMC3356038]. https://doi.org/10.3389/fendo.2011.00032.
-
4.
Lan H, Zheng X, Khan MA, Li S. Anti-idiotypic antibody: A new strategy for the development of a growth hormone receptor antagonist. Int J Biochem Cell Biol. 2015;68:101-8. [PubMed ID: 26369868]. https://doi.org/10.1016/j.biocel.2015.09.004.
-
5.
Li W, Lan H, Liu H, Fu Z, Yang Y, Han W, et al. The activation and differential signalling of the growth hormone receptor induced by pGH or anti-idiotypic monoclonal antibodies in primary rat hepatocytes. Mol Cell Endocrinol. 2013;376(1-2):51-9. [PubMed ID: 23769824]. https://doi.org/10.1016/j.mce.2013.06.008.
-
6.
Waters MJ, Hoang HN, Fairlie DP, Pelekanos RA, Brown RJ. New insights into growth hormone action. J Mol Endocrinol. 2006;36(1):1-7. [PubMed ID: 16461922]. https://doi.org/10.1677/jme.1.01933.
-
7.
Hermansson M, Wickelgren RB, Hammarqvist F, Bjarnason R, Wennstrom I, Wernerman J, et al. Measurement of human growth hormone receptor messenger ribonucleic acid by a quantitative polymerase chain reaction-based assay: demonstration of reduced expression after elective surgery. J Clin Endocrinol Metab. 1997;82(2):421-8. [PubMed ID: 9024230]. https://doi.org/10.1210/jcem.82.2.3718.
-
8.
Brooks AJ, Waters MJ. The growth hormone receptor: mechanism of activation and clinical implications. Nat Rev Endocrinol. 2010;6(9):515-25. [PubMed ID: 20664532]. https://doi.org/10.1038/nrendo.2010.123.
-
9.
Lanning NJ, Carter-Su C. Recent advances in growth hormone signaling. Rev Endocr Metab Disord. 2006;7(4):225-35. [PubMed ID: 17308965]. https://doi.org/10.1007/s11154-007-9025-5.
-
10.
Zhu T, Goh ELK, Graichen R. Signal transduction via the growth hormone receptor[J]. Cellular Signal. 2001;13(9):599-616.
-
11.
Hong P, Lan H, Li Y, Fu Z, Zheng X. Different intracellular signalling properties induced by human and porcine growth hormone. Gen Comp Endocrinol. 2016;229:67-73. [PubMed ID: 26944485]. https://doi.org/10.1016/j.ygcen.2016.02.022.
-
12.
Lan H, Li W, Fu Z, Yang Y, Wu T, Liu Y, et al. Differential intracellular signalling properties of the growth hormone receptor induced by the activation of an anti-GHR antibody. Mol Cell Endocrinol. 2014;390(1-2):54-64. [PubMed ID: 24755421]. https://doi.org/10.1016/j.mce.2014.04.004.
-
13.
Liu JL, LeRoith D. Insulin-like growth factor I is essential for postnatal growth in response to growth hormone. Endocrinology. 1999;140(11):5178-84. [PubMed ID: 10537147]. https://doi.org/10.1210/endo.140.11.7151.
-
14.
Tang Z, Yu R, Lu Y, Parlow AF, Liu JL. Age-dependent onset of liver-specific IGF-I gene deficiency and its persistence in old age: implications for postnatal growth and insulin resistance in LID mice. Am J Physiol Endocrinol Metab. 2005;289(2):E288-95. [PubMed ID: 15769793]. https://doi.org/10.1152/ajpendo.00494.2004.
-
15.
Lupu F, Terwilliger JD, Lee K, Segre GV, Efstratiadis A. Roles of growth hormone and insulin-like growth factor 1 in mouse postnatal growth. Dev Biol. 2001;229(1):141-62. [PubMed ID: 11133160]. https://doi.org/10.1006/dbio.2000.9975.
-
16.
Ambler GR, Breier BH, Surus A. The interrelationship between and the regulation of hepatic growth hormone receptors and circulating GH binding protein in the pig[J]. Acta Endocrinol. 1992;126(2):155-61.
-
17.
Tiong TS, Herington AC. Ontogeny of messenger RNA for the rat growth hormone receptor and serum binding protein[J]. Molecular Cellular Endocrinolog. 1992;83(2):133-41.
-
18.
Maes M, De Hertogh R, Watrin-Granger P, Ketelslegers JM. Ontogeny of liver somatotropic and lactogenic binding sites in male and female rats. Endocrinology. 1983;113(4):1325-32. [PubMed ID: 6311516]. https://doi.org/10.1210/endo-113-4-1325.
-
19.
Mathews LS, Enberg B, Norstedt G. Regulation of rat growth hormone receptor gene expression. J Biol Chem. 1989;264(17):9905-10. [PubMed ID: 2722883].
-
20.
Martinez CS, Piazza VG, Ratner LD, Matos MN, Gonzalez L, Rulli SB, et al. Growth hormone STAT5-mediated signaling and its modulation in mice liver during the growth period. Growth Horm IGF Res. 2013;23(1-2):19-28. [PubMed ID: 23245546]. https://doi.org/10.1016/j.ghir.2012.11.002.
-
21.
Ocaranza P, Morales F, Matamala A, Gaete X, Roman R, Lammoglia JJ, et al. Growth hormone signaling in fibroblasts from newborn boys and prepubertal boys. Growth Horm IGF Res. 2016;27:18-27. [PubMed ID: 26843474]. https://doi.org/10.1016/j.ghir.2016.01.003.
-
22.
Kopchick JJ, Andry JM. Growth hormone (GH), GH receptor, and signal transduction. Mol Genet Metab. 2000;71(1-2):293-314. [PubMed ID: 11001823]. https://doi.org/10.1006/mgme.2000.3068.
-
23.
Ribaux P, Gjinovci A, Sadowski HB, Iynedjian PB. Discrimination between signaling pathways in regulation of specific gene expression by insulin and growth hormone in hepatocytes. Endocrinology. 2002;143(10):3766-72. [PubMed ID: 12239086]. https://doi.org/10.1210/en.2002-220304.
-
24.
Lan H, Li W, Jiang H, Yang Y, Zheng X. Intracellular signaling transduction pathways triggered by a well-known anti-GHR monoclonal antibody, Mab263, in vitro and in vivo. Int J Mol Sci. 2014;15(11):20538-54. [PubMed ID: 25391041]. [PubMed Central ID: PMC4264182]. https://doi.org/10.3390/ijms151120538.
-
25.
Schmidmaier G, Wildemann B, Heeger J. Improvement of fracture healing by systemic administration of growth hormone and local application of insulin-like growth factor-1 and transforming growth factor-β1. Bone. 2002;31(1):165-72.
-
26.
Lan HN, Jiang HL, Li W, Wu TC, Hong P, Li YM, et al. Development and Characterization of a Novel Anti-idiotypic Monoclonal Antibody to Growth Hormone, Which Can Mimic Physiological Functions of Growth Hormone in Primary Porcine Hepatocytes. Asian-Australas J Anim Sci. 2015;28(4):573-83. [PubMed ID: 25656185]. [PubMed Central ID: PMC4341108]. https://doi.org/10.5713/ajas.14.0600.
-
27.
Pantaleon M, Whiteside EJ, Harvey MB. Functional growth hormone (GH) receptors and GH are expressed by preimplantation mouse embryos: A role for GH in early embryogenesis?[J]. Proceed Nation Academ Sci. 1997;94(10):5125-30.
-
28.
Phornphutkul C, Frick GP, Goodman HM, Berry SA, Gruppuso PA. Hepatic growth hormone signaling in the late gestation fetal rat. Endocrinology. 2000;141(10):3527-33. [PubMed ID: 11014205]. https://doi.org/10.1210/endo.141.10.7702.
-
29.
Garcia-Aragon J, Lobie PE, Muscat GE, Gobius KS, Norstedt G, Waters MJ. Prenatal expression of the growth hormone (GH) receptor/binding protein in the rat: a role for GH in embryonic and fetal development? Development. 1992;114(4):869-76. [PubMed ID: 1618149].
-
30.
Ram PA, Waxman DJ. Interaction of growth hormone-activated STATs with SH2-containing phosphotyrosine phosphatase SHP-1 and nuclear JAK2 tyrosine kinase. J Biologic Chemistr. 1997;272(28):17694-702.
-
31.
Croker BA, Kiu H, Nicholson SE. SOCS regulation of the JAK/STAT signaling pathway[C]//Seminars in cell & developmental biology. Academic Press. 2008;19(4):414-22.
-
32.
Flores-Morales A, Greenhalgh CJ, Norstedt G, Rico-Bautista E. Negative regulation of growth hormone receptor signaling. Mol Endocrinol. 2006;20(2):241-53. [PubMed ID: 16037128]. https://doi.org/10.1210/me.2005-0170.
-
33.
Greenhalgh CJ, Alexander WS. Suppressors of cytokine signalling and regulation of growth hormone action. Growth Horm IGF Res. 2004;14(3):200-6. [PubMed ID: 15125881]. https://doi.org/10.1016/j.ghir.2003.12.011.
-
34.
Gu F, Dube N, Kim JW, Cheng A, Ibarra-Sanchez Mde J, Tremblay ML, et al. Protein tyrosine phosphatase 1B attenuates growth hormone-mediated JAK2-STAT signaling. Mol Cell Biol. 2003;23(11):3753-62. [PubMed ID: 12748279]. [PubMed Central ID: PMC155228].
-
35.
Pilecka I, Patrignani C, Pescini R, Curchod ML, Perrin D, Xue Y, et al. Protein-tyrosine phosphatase H1 controls growth hormone receptor signaling and systemic growth. J Biol Chem. 2007;282(48):35405-15. [PubMed ID: 17921143]. https://doi.org/10.1074/jbc.M705814200.
-
36.
Herrington J, Carter-Su C. Signaling pathways activated by the growth hormone receptor. Trend Endocrinol Metabolis. 2001;12(6):252-7.
-
37.
Keshet Y, Seger R. The MAP kinase signaling cascades: a system of hundreds of components regulates a diverse array of physiological functions. Methods Mol Biol. 2010;661:3-38. [PubMed ID: 20811974]. https://doi.org/10.1007/978-1-60761-795-2_1.
-
38.
Wainstein E, Seger R. The dynamic subcellular localization of ERK: mechanisms of translocation and role in various organelles. Curr Opin Cell Biol. 2016;39:15-20. [PubMed ID: 26827288]. https://doi.org/10.1016/j.ceb.2016.01.007.
-
39.
Nissen PM, Danielsen VO, Jorgensen PF, Oksbjerg N. Increased maternal nutrition of sows has no beneficial effects on muscle fiber number or postnatal growth and has no impact on the meat quality of the offspring. J Anim Sci. 2003;81(12):3018-27. [PubMed ID: 14677857]. https://doi.org/10.2527/2003.81123018x.