Abstract
Background:
Polycystic ovary syndrome (PCOS) is one of the most common endocrine disorders in women during the reproductive age. Considering disorders reported in women with PCOS, e.g. infertility, pregnancy complications (premature delivery, caesarean section and spontaneous miscarriages), and hormonal disorders, we hypothesized that uterine contractions in PCOS rats may be different from controls. We aimed to compare uterine contractions in PCOS rats with controls.Methods:
Rats in the experimental group were subcutaneously injected with 5 mg of free testosterone on gestational day 20, while controls received solvent. The contractions of isolated uterus in offspring of both groups were recorded by the power lab system, after exposure to carbachol and oxytocin. Two-way analysis of variance was used to compare results between 2 groups.Results:
PCOS rats showed more irregular uterine contractions compared to controls. After exposure to carbachol, frequency, and resting tone in the PCOS rats were significantly increased compared to controls (P = 0.004, P = 0.02, respectively). After exposure to oxytocin, the frequency, resting tone and amplitude of rhythmic contractions did not differ between the 2 groups.Conclusions:
Our study indicates irregular uterine contractions and different mechanical responses of isolated uterus in PCOS rats compared to controls.Keywords
1. Background
Polycystic ovary syndrome (PCOS), one of the most common endocrine disorders in women of reproductive age, has an estimated prevalence varying from 3% - 20%, depending on the diagnostic criteria used (1-3). According to the Rotterdam criteria, PCOS is diagnosed by any 2 or all 3 of the following criteria: hyperandrogenemia, oligo-anovulation, and polycystic ovaries (4).
Precise and adequate uterine contractile activity is vital for many reproductive functions, including sperm transportation, successful embryo transport and implantation, and also pregnancy and parturition (5); therefore, the normal function of uterus smooth muscle is important. Abnormal uterine contractility may underlie important clinical pathologies such as infertility, implantation failure, ectopic pregnancies, spontaneous miscarriages, preterm birth, and caesarean section; some of these disorders are reported in PCOS women (6-12).
There is a clinical relationship between uterine contractility and steroid hormones (13, 14).
Progesterone and estrogen are key regulators of myometrial growth and contractility and uterine peristalsis (15). Progesterone and estrogen play an important role in determining the contractile state of the pregnancy myometrium and the timing and process of parturition (15). In addition, recent studies have reported a shorter gestation and preterm delivery in hyperandrogenic PCOS women that may be due to the effects of androgen on myometrial contractions.
There is limited data concerning uterine contractility in PCOS women. Considering the ethical and practical limitations in human studies, animal models of PCOS are useful resources for further studies about uterine contractility in this syndrome.
We previously introduced a rat model of PCOS, induced by prenatal exposure to a single dose of testosterone that exhibited reproductive, ovarian, and metabolic disturbances similar to human PCOS, with minimum reproductive system morphological abnormalities (16, 17). Following reported disorders in PCOS women such as, infertility, pregnancy complications, and hormonal disorders, we hypothesized that uterine contractions in PCOS rats may be different from controls, in this study, we aimed to compare uterine contractions in PCOS rats with controls in adulthood.
2. Methods
2.1. Animals and Care
A total of 14 female Wistar rats were obtained from the Research Institute for Endocrine Sciences (RIES) animal center of Shahid Beheshti University of Medical Sciences (SBUMS), Tehran, Iran. One pair of male (120 - 130 days of age) and female (75 - 95 days of age) rats were kept in a polypropylene cage (43 cm × 30 cm × 15 cm) overnight in standard animal housing conditions (12 hours - 12 hours light/dark cycles and controlled temperature of 22 ± 3°C, relative humidity 45% - 55%), and water and food were available ad libitum. Observation of a vaginal plug after mating was considered as the 1st day of pregnancy. Pregnant rats were randomly divided into 2 groups, the experimental and control groups (7 rats in each group).
2.2. Hormonal Treatment for Producing a rat Model of PCOS and Animal Maintenance
Seven pregnant rats in the experimental group received 5 mg of free testosterone (T1500; Sigma, Steinheim, Germany) dissolved in a 500μl of sesame oil (S3547, Sigma, Steinheim, Germany) and benzyl benzoate (B6630, Sigma, Steinheim, Germany) with a ratio of 4:1 by subcutaneous injection on the 20th day of pregnancy (16). A total of 7 pregnant rats in the control group were simultaneously injected with only 500 µL of solvent (sesame oil and benzyl benzoate). After a weaning period, female offspring of experimental and control groups were kept in groups of 4 per cage with ad libitum food and water. Prenatally androgenized (PNA) rats (rat model of PCOS) (n = 7) and controls (n = 7) between 100 - 110 days of age (in adulthood) were assessed for uterine contractility.
2.3. Isolated Tissue Bath Protocol and Determination of Mechanical Responses
Mechanical responses (resting tone, amplitude and frequency of rhythmic contractions) of isolated uterus were recorded in PCOS rats and controls, in the estrus phase, recognized by vaginal smear (18).
PCOS rats and the controls were anaesthetized by i.p. injection of pentobarbital sodium (P3761; Sigma) (60 mg/kg), the abdomen of animals was opened, both uterine horns were extracted (following extraction of uterine horns, rats were killed by heart incision) and placed in ice-cold modified Krebs’ solution (NaHCO3, MgCl2, NaH2PO4, NaCl, KCl, CaCl2, Glucose, and Ethylenediaminetetraacetic acid (EDTA) (pH = 7.4), bubbled with 95% O2 and 5% CO2, the fat was removed, the right horn of uterus was cut into longitudinal strips, 2 mm in width and 5 mm in length. In order to measure mechanical responses of isolated uterus, each uterine strip was vertically mounted in an organ bath containing modified Krebs’ solution, continuously bubbled with 95% O2 and 5% CO2 and warmed to 37°C. The length of the strips was adjusted several times until a stable tension of 1 g was attained (5). The isolated tissue bath was used to measure isometric contractility of longitudinal muscle using a transducer and changes in isometric force were recorded on an AD instruments power lab and data were saved electronically and quantified using the program Chart 7.0.
Prior to starting the experimental protocol, strips were allowed to equilibrate for at least 60 minutes in the bathing solution, and the solution was replaced every 15 minutes. KCl (60 mM), then, was added to the bathing solution to obtain reference contractions and the responses were recorded for 3 minutes; uteri were washed by replacing the bathing solution with fresh Krebs, until tone returned to baseline contraction status and then different doses of contractile agonists including carbachol (CCh, a cholinergic muscarinic agonist) and oxytocin (OXY), (CCh: 0.0008 g/mL and OXY: 5 IU/mL) were added to the bathing solution, respectively.
A 3-minute period of contractions to each dose of CCh was established and there was a 3-minute period of washout between different doses until the tone returned to the baseline; doses used for CCh included, 0.5, 1, 2, 3, 4, 5, and 6 (µM), in that order, with an increasing trend. OXY was then investigated in the same manner, doses including 0.1, 0.5, 1, 3, 5 and 10 (mU/mL), were added to the bathing solution. The KCl challenge was repeated at the end of the experiments to verify the viability of the tissues during the experiment.
CCh and OXY-induced uterine contractions were assessed by changes in the resting tone, amplitude of rhythmic contractions (maximal contraction minus basal resting tone), and frequency of rhythmic contractions (the number of rhythmic contractions), during 3-minute period of contraction after adding each dose of contractile agonists. Increases in resting tone and amplitude of rhythmic contractions were reported as percentages of 60 mM KCl-induced contractions.
2.4. Statistical Analysis
Data analysis was performed using the SPSS 15.0 PC package (SPSS Inc., Chicago, IL, USA). All data are expressed as mean ± SEM. Two-way analysis of variance (ANOVA) was used to compare results between the PCOS rats and controls, P values < 0.05 were considered significant.
3. Results
Uterine contractions were more irregular (higher frequency and resting tone, irregular pulses and pulse in washout period) in PCOS rats than controls, after exposure to both contractile agonists (CCh and OXY) (Figures 1 and 2).
Uterine contractions after exposure to carbachol (CCh). Uterine contractions in PCOS rats: A, in controls B, doses of CCh: 1 = 0.5, 2 = 1, 3 = 2, 4 = 3, 5 = 4, 6 = 5 and 7 = 6 (µM).
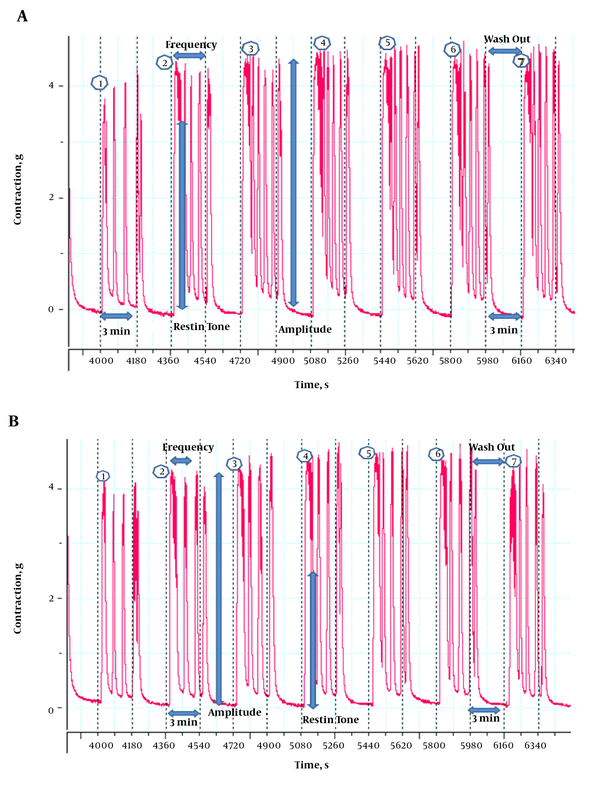
Uterine contractions after exposure to oxytocin (OXY). Uterine contractions in PCOS rats: A, in controls B, doses of OXY: 1 = 0.1, 2 = 0.5, 3 = 1, 4 = 3, 5 = 5, and 6 = 10 (mU/mL).
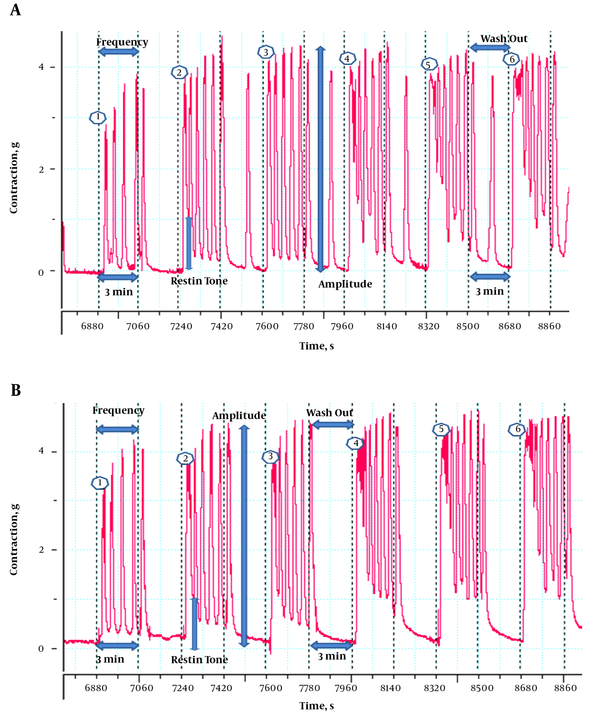
3.1. Carbachol
After exposure of isolated uterus to CCh, resting tone and frequency of rhythmic contractions increased significantly in the uterus of PCOS rats compared to controls (P = 0.02 and P = 0.004, respectively), (Figure 3A and B). In PCOS rats, resting tone showed an increasing trend until the 4th dose, after which it indicated a stationary state (with small changes) (Figure 3A); in addition, in PCOS rats, despite the increasing dose of CCh, frequency of rhythmic contractions showed minor changes, after the 3rd dose (Figure 3B), while in controls a more regular trend was observed, for both parameters (resting tone and frequency).
Uterine contractions after exposure to carbachol. Data are expressed as mean ± SEM. Comparison of resting tone: A, frequency of rhythmic contractions; B, and amplitude of rhythmic contractions; C, between PCOS rats and controls. CCh doses: 0.5, 1, 2, 3, 4, 5, and 6 (µM). * P < 0.05, ** P < 0.01.
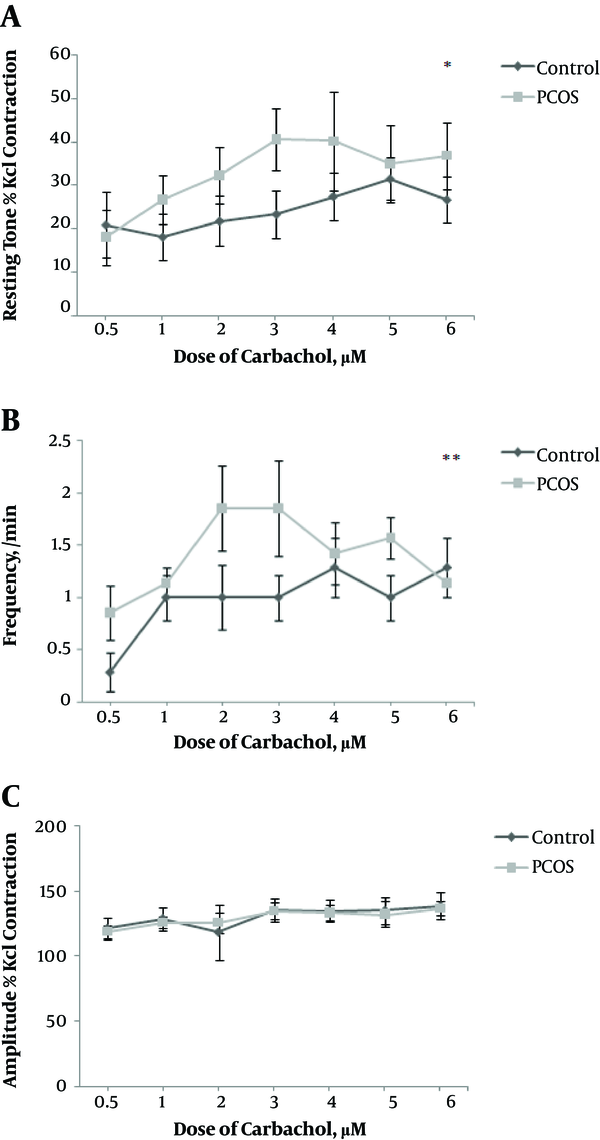
After exposure of isolated uterus to CCh, no significant difference was observed in the amplitude of rhythmic contractions between the 2 groups (Figure 3C).
3.2. Oxytocin
No significant differences were observed in the resting tone, frequency, and amplitude of rhythmic contractions between the 2 groups, after exposure of isolated uterus to OXY (Figure 4A, B and C).
Uterine contractions after exposure to oxytocin. Data are expressed as mean ± SEM. Comparison of resting tone: A, frequency of rhythmic contractions, B, and amplitude of rhythmic contractions, C, between PCOS rats and controls. OXY doses: 0.1, 0.5, 1, 3, 5, and 10 (mU/mL).
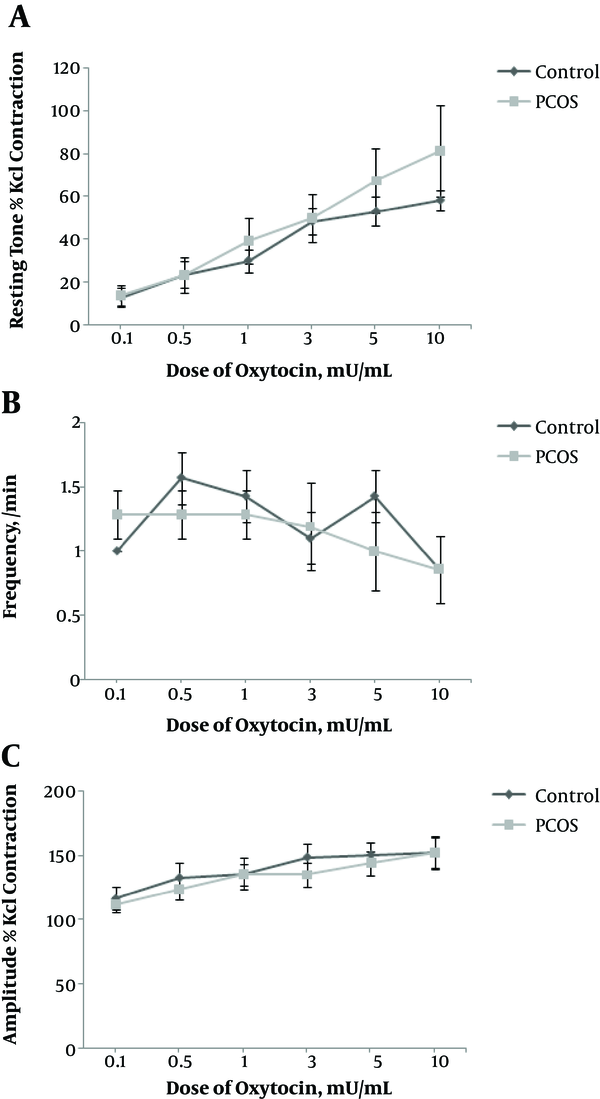
With increasing dose of OXY, resting tone increased in PCOS rats, while in controls the changes of resting tone were less (Figure 4A).
In controls, with different doses of OXY, the frequency showed more changes compared to PCOS rats. In PCOS rats, at doses of 0.1, 0.5, and 1 (mU/mL), frequency remained unchanged, while decreased at higher doses (Figure 4B). In this study, despite increasing doses of OXY, frequency of rhythmic contractions decreased, possibly indicating OXY resistance in the PCOS rats, a finding that needs to be further studied.
4. Discussion
In this study, we compared the mechanical responses of isolated uterus (resting tone, amplitude and frequency of rhythmic contractions) after exposure to CCh and OXY between PCOS rats and controls. Our findings showed that, after exposure to CCh and OXY (contractile agonists), uterine contractions were more irregular (higher frequency and resting tone, irregular pulses and pulse in washout period) in the PCOS rats, compared to controls. CCh caused 2 types of responses in the uterus of PCOS rats; significant increases in resting tone and frequency of rhythmic contractions compared to controls. On the other hand, after exposure to OXY no significant differences were observed in mechanical responses of isolated uterus between 2 groups.
It has been shown that CCh and OXY increase cytosolic Ca2+ levels in myometrial cells (19, 20), leading to contraction of the uterus. Increased cytosolic free Ca2+ concentration has been considered as the primary signal for myometrium contraction (21-23).
In many smooth muscle tissues, mixed populations of muscarinic receptors are present. In rat myometrium, muscarinic M2, and M3 receptors have been identified (24, 25). CCh, through activation of muscarinic receptors, leads to cytosolic Ca2+ increase.
In the present study, after exposure to CCh, the frequency of isolated uterus increased in the PCOS rats, compared to controls; higher frequency of contractions observed in the PCOS rats is similar to those of a previous study conducted on humans reporting faster peristaltic frequency in women with PCOS compared to controls (26).
OXY is largely produced by the hypothalamus-pituitary and other tissues including the corpus luteum, adrenal medulla, and placenta (27-30); OXY effects are demonstrated via specific oxytocin receptors (OXYRs), expressed in the uterus, mammary gland, and brain.
Despite some changes observed in mechanical responses of the uterus in PCOS rats after exposure to CCh (frequency and resting tone), there were no significant differences in resting tone, amplitude, and frequency of rhythmic contractions of uterus between the 2 groups after exposure to OXY.
These inconsistencies in the responses to two contractile agonists (CCh and OXY) that observed between two groups of our study may be explained by differences in the number of receptors, the sensitivity of receptors, alterations in Ca2+ channels, the effects of hormones (e.g. high levels of androgen) on CCh receptors-upregulation in PCOS, up-regulation of phospholipase C1 (PLC1), and phospholipase C3 (PLC3) enzymes; the PLC signaling pathway plays an important role in the regulation of uterine contractility (31). One of the most important roles of OXY is initiation and maintenance of mammalian labor (19, 32); it has been suggested that at the end of pregnancy, uterine myometrium becomes extremely sensitive to OXY due to a rapid increase in the number of OXYRs (33-35). In addition, it has been reported that oxytocin induces greater force in pregnant myometrium than in non-pregnant myometrium (36). In the present study, non-significant differences in mechanical responses of isolated uterus to OXY may be due to use myometrium of non-pregnant rats, dosage of OXY, and duration of exposure. However, these need to be further researched.
We did not observe significant differences between our own study groups in amplitude of rhythmic contractions after exposure of isolated uterus to CCh and OXY, a finding is similar to that of another previous study, which showed no difference in amplitude of peristalsis contractions in PCOS women, compared to controls (26).
To our knowledge, it is the 1st study to investigate the contractions of isolated uterus in PCOS rats, compared to controls. This model may contribute to a better understanding of the cellular and molecular mechanisms involved in uterine contractions in PCOS, the study of which is impossible in humans. However, some limitations of our study include; 1, not evaluating uterine contractility in tissue from pregnant rats due to ethical limitations; this may affect the interpretation of our results and 2, the lack of similar studies for interpretation our data.
Further detailed intracellular signaling investigations suggested for future studies are the examination of expression of CCh and OXY receptors genes, histological examinations of uterus in PCOS rats, and examination of uterus contractions during longer time periods and with higher doses of contractile agonists.
4.1. Conclusions
Our study indicates more irregular uterine contractions and different mechanical responses of isolated uterus in PCOS rats compared to controls. One of the reasons for infertility and pregnancy complications (e.g. premature delivery, caesarean section and spontaneous miscarriages) in women with PCOS may be due to disturbed uterine contractions; a hypothesis however that needs to be further investigated in the future.
Acknowledgements
References
-
1.
Carmina E, Azziz R. Diagnosis, phenotype, and prevalence of polycystic ovary syndrome. Fertil Steril. 2006;86 Suppl 1:S7-8. [PubMed ID: 16798288]. https://doi.org/10.1016/j.fertnstert.2006.03.012.
-
2.
Franks S. Polycystic ovary syndrome. N Engl J Med. 1995;333(13):853-61. [PubMed ID: 7651477]. https://doi.org/10.1056/NEJM199509283331307.
-
3.
Ehrmann DA. Polycystic ovary syndrome. N Engl J Med. 2005;352(12):1223-36. [PubMed ID: 15788499]. https://doi.org/10.1056/NEJMra041536.
-
4.
Rotterdam EAPCWG. Revised 2003 consensus on diagnostic criteria and long-term health risks related to polycystic ovary syndrome (PCOS). Hum Reprod. 2004;19(1):41-7. [PubMed ID: 14688154]. https://doi.org/10.1093/humrep/deh098.
-
5.
Darios ES, Seitz B, Watts SW. Smooth muscle pharmacology in the isolated virgin and pregnant rat uterus and cervix. J Pharmacol Exp Ther. 2012;341(3):587-96. [PubMed ID: 22366660]. https://doi.org/10.1124/jpet.111.191031.
-
6.
Qin JZ, Pang LH, Li MJ, Fan XJ, Huang RD, Chen HY. Obstetric complications in women with polycystic ovary syndrome: a systematic review and meta-analysis. Reprod Biol Endocrinol. 2013;11:56. [PubMed ID: 23800002]. https://doi.org/10.1186/1477-7827-11-56.
-
7.
Fuchs AR, Behrens O, Maschek H, Kupsch E, Einspanier A. Oxytocin and vasopressin receptors in human and uterine myomas during menstrual cycle and early pregnancy. Hum Reprod Update. 1998;4(5):594-604. [PubMed ID: 10027613]. https://doi.org/10.1093/humupd/4.5.594.
-
8.
Goldenberg RL. The management of preterm labor. Obstet Gynecol. 2002;100(5 Pt 1):1020-37. [PubMed ID: 12423870]. https://doi.org/10.1097/00006250-200211000-00033.
-
9.
Goldenberg RL, Hauth JC, Andrews WW. Intrauterine infection and preterm delivery. N Engl J Med. 2000;342(20):1500-7. [PubMed ID: 10816189]. https://doi.org/10.1056/NEJM200005183422007.
-
10.
Gimpl G, Fahrenholz F. The oxytocin receptor system: structure, function, and regulation. Physiol Rev. 2001;81(2):629-83. [PubMed ID: 11274341]. https://doi.org/10.1152/physrev.2001.81.2.629.
-
11.
Birch Petersen K, Pedersen NG, Pedersen AT, Lauritsen MP, la Cour Freiesleben N. Mono-ovulation in women with polycystic ovary syndrome: a clinical review on ovulation induction. Reprod Biomed Online. 2016;32(6):563-83. [PubMed ID: 27151490]. https://doi.org/10.1016/j.rbmo.2016.03.006.
-
12.
Sawada M, Masuyama H, Hayata K, Kamada Y, Nakamura K, Hiramatsu Y. Pregnancy complications and glucose intolerance in women with polycystic ovary syndrome. Endocr J. 2015;62(11):1017-23. [PubMed ID: 26370557]. https://doi.org/10.1507/endocrj.EJ15-0364.
-
13.
Bulletti C, DeZiegler D, Stefanetti M, Cicinelli E, Pelosi E, Flamigni C. Endometriosis: absence of recurrence in patients after endometrial ablation. Hum Reprod. 2001;16(12):2676-9. [PubMed ID: 11726595].
-
14.
Bulletti C, DeZiegler D, Stefanetti M, Cincinelli E, Pelosi E, Flamigni C. Endometriosis: Absence of recurrence in patients after endometrial ablation. Obstet Gynecol Surv. 2002;57(6):359-60. https://doi.org/10.1097/00006254-200206000-00019.
-
15.
Mesiano S, Welsh TN. Steroid hormone control of myometrial contractility and parturition. Semin Cell Dev Biol. 2007;18(3):321-31. [PubMed ID: 17613262]. https://doi.org/10.1016/j.semcdb.2007.05.003.
-
16.
Tehrani FR, Noroozzadeh M, Zahediasl S, Piryaei A, Azizi F. Introducing a rat model of prenatal androgen-induced polycystic ovary syndrome in adulthood. Exp Physiol. 2014;99(5):792-801. [PubMed ID: 24532600]. https://doi.org/10.1113/expphysiol.2014.078055.
-
17.
Noroozzadeh M, Ramezani Tehrani F, Sedaghat K, Godini A, Azizi F. The impact of prenatal exposure to a single dose of testosterone on insulin resistance, glucose tolerance and lipid profile of female rat's offspring in adulthood. J Endocrinol Invest. 2015;38(5):489-95. [PubMed ID: 25527160]. https://doi.org/10.1007/s40618-014-0198-y.
-
18.
Marcondes FK, Bianchi FJ, Tanno AP. Determination of the estrous cycle phases of rats: some helpful considerations. Braz J Biol. 2002;62(4A):609-14. [PubMed ID: 12659010]. https://doi.org/10.1590/S1519-69842002000400008.
-
19.
Szal SE, Repke JT, Seely EW, Graves SW, Parker CA, Morgan KG. [Ca2+]i signaling in pregnant human myometrium. Am J Physiol. 1994;267(1 Pt 1):E77-87. [PubMed ID: 8048517]. https://doi.org/10.1152/ajpendo.1994.267.1.E77.
-
20.
Anwer K, Sanborn BM. Changes in intracellular free calcium in isolated myometrial cells: role of extracellular and intracellular calcium and possible involvement of guanine nucleotide-sensitive proteins. Endocrinology. 1989;124(1):17-23. [PubMed ID: 2491804]. https://doi.org/10.1210/endo-124-1-17.
-
21.
Marc S, Leiber D, Harbon S. Carbachol and oxytocin stimulate the generation of inositol phosphates in the guinea pig myometrium. FEBS Lett. 1986;201(1):9-14. [PubMed ID: 3011507]. https://doi.org/10.1016/0014-5793(86)80561-0.
-
22.
Molnar M, Hertelendy F. Regulation of intracellular free calcium in human myometrial cells by prostaglandin F2 alpha: comparison with oxytocin. J Clin Endocrinol Metab. 1990;71(5):1243-50. [PubMed ID: 2229283]. https://doi.org/10.1210/jcem-71-5-1243.
-
23.
Thornton S, Gillespie JI, Greenwell JR, Dunlop W. Mobilization of calcium by the brief application of oxytocin and prostaglandin E2 in single cultured human myometrial cells. Exp Physiol. 1992;77(2):293-305. [PubMed ID: 1581057]. https://doi.org/10.1113/expphysiol.1992.sp003589.
-
24.
Varol FG, Hadjiconstantinou M, Zuspan FP, Neff NH. Pharmacological characterization of the muscarinic receptors mediating phosphoinositide hydrolysis in rat myometrium. J Pharmacol Exp Ther. 1989;249(1):11-5. [PubMed ID: 2540309].
-
25.
Pennefather JN, Gillman TA, Mitchelson F. Muscarinic receptors in rat uterus. Eur J Pharmacol. 1994;262(3):297-300. [PubMed ID: 7813596]. https://doi.org/10.1016/0014-2999(94)90745-5.
-
26.
Leonhardt H, Gull B, Kishimoto K, Kataoka M, Nilsson L, Janson PO, et al. Uterine morphology and peristalsis in women with polycystic ovary syndrome. Acta Radiol. 2012;53(10):1195-201. [PubMed ID: 23081959]. https://doi.org/10.1258/ar.2012.120384.
-
27.
Ang VT, Jenkins JS. Neurohypophysial hormones in the adrenal medulla. J Clin Endocrinol Metab. 1984;58(4):688-91. [PubMed ID: 6699132]. https://doi.org/10.1210/jcem-58-4-688.
-
28.
Fields PA, Eldridge RK, Fuchs AR, Roberts RF, Fields MJ. Human placental and bovine corpora luteal oxytocin. Endocrinology. 1983;112(4):1544-6. [PubMed ID: 6832059]. https://doi.org/10.1210/endo-112-4-1544.
-
29.
Wathes DC, Swann RW, Pickering BT, Porter DG, Hull MG, Drife JO. Neurohypophysial hormones in the human ovary. Lancet. 1982;2(8295):410-2. [PubMed ID: 6124806].
-
30.
Wathes DC, Swann RW. Is oxytocin an ovarian hormone? Nature. 1982;297(5863):225-7. [PubMed ID: 7078636]. https://doi.org/10.1038/297225a0.
-
31.
Houdeau E, Levy A, Mhaouty-Kodja S. Up-regulation of rat myometrial phospholipases C{beta}1 and C{beta}3 correlates with increased term sensitivity to carbachol and oxytocin. J Endocrinol. 2005;187(2):197-204. [PubMed ID: 16293767]. https://doi.org/10.1677/joe.1.06388.
-
32.
Walter R, Dubois BM, Schwartz IL. Biological significance of the amino acid residue in position 3 of neurohypophyseal hormones and the effect of magnesium on their uterotonic action. Endocrinology. 1968;83(5):979-83. [PubMed ID: 5685578]. https://doi.org/10.1210/endo-83-5-979.
-
33.
Soloff MS, Alexandrova M, Fernstrom MJ. Oxytocin receptors: triggers for parturition and lactation? Science. 1979;204(4399):1313-5. [PubMed ID: 221972]. https://doi.org/10.1126/science.221972.
-
34.
Alexandrova M, Soloff MS. Oxytocin receptors and parturition. I. Control of oxytocin receptor concentration in the rat myometrium at term. Endocrinology. 1980;106(3):730-5. [PubMed ID: 6243547]. https://doi.org/10.1210/endo-106-3-730.
-
35.
Fuchs AR, Helmer H, Behrens O, Liu HC, Antonian L, Chang SM, et al. Oxytocin and bovine parturition: a steep rise in endometrial oxytocin receptors precedes onset of labor. Biol Reprod. 1992;47(6):937-44. [PubMed ID: 1337277]. https://doi.org/10.1095/biolreprod47.6.937.
-
36.
Kim BK, Ozaki H, Hori M, Takahashi K, Karaki H. Increased contractility of rat uterine smooth muscle at the end of pregnancy. Comp Biochem Physiol A Mol Integr Physiol. 1998;121(2):165-73. [PubMed ID: 9883577]. https://doi.org/10.1016/S1095-6433(98)10118-6.