Abstract
Background:
Fibromyalgia is recognized as a chronic pain syndrome. Widespread pain is a common symptom in fibromyalgia, indicating a potential dysfunction in the processing of painful sensations in the central nervous system.Objectives:
This study aimed to investigate changes in the severity of clinical symptoms in fibromyalgia patients and to evaluate the apparent diffusion coefficients and metabolites in the brain of these patients.Patients and Methods:
This cross-sectional study included 28 female outpatients with complaints of widespread pain, who were diagnosed with fibromyalgia syndrome. Magnetic resonance imaging, magnetic resonance spectroscopy, and diffusion tensor examinations were applied to evaluate patients diagnosed with fibromyalgia before treatment.Results:
The mean age of the patients included in this study was 39.1 ± 8.6 years (range, 24 - 55 years). A statistically significant strong positive correlation was found between the scores of the visual analog scale and the fibromyalgia impact questionnaire. Besides, significant associations were found between the scores of the visual analog scale and fibromyalgia impact questionnaire and the results of fractional anisotropy, apparent diffusion coefficient, and peak values of some metabolites in spectroscopy.Conclusion:
The results of this study suggest that metabolites play an inhibitory or excitatory role in the central pain mechanisms of fibromyalgia as a chronic pain syndrome.Keywords
Fibromyalgia Brain Magnetic Resonance Spectroscopy Diffusion Tensor Imaging Pain
1. Background
Fibromyalgia syndrome (FMS) is a chronic pain syndrome, which affects 2 - 4% of the population in developed countries. However, the underlying cause of this syndrome remains unknown. Widespread pain is a common symptom of FMS, indicating a potential dysfunction in the processing of painful sensations in the central nervous system (1-4). Diffusion-weighted imaging (DWI) is a physiological imaging sequence sensitive to water movement in tissues at the cellular level. Moreover, diffusion tensor (DT) imaging indicates the microscopic structure and integrity of the white matter, and fractional anisotropy (FA) represents the amount and direction of diffusion in the target region. The FA values are indicators of the integrity of the white matter; a reduction in these values reflects damage to the white matter (5). Additionally, magnetic resonance spectroscopy (MRS) is a non-invasive technique, indicating the biochemical tissue components and providing a graphic representation of the range and number of metabolites in the specified tissue in an in vivo environment (6).
2. Objectives
This study aimed to investigate the correlation between the severity of clinical symptoms and the FA value, apparent diffusion coefficient (ADC), and metabolites in the brain of FM patients.
3. Patients and Methods
The local ethics committee approved this cross-sectional study (Decision No.: E.31554 HRU, date: 19.07.05). The study was performed on 28 female outpatients with complaints of widespread pain, who were diagnosed with FMS. The inclusion criteria were as follows: Age range of 18 - 55 years; meeting the 2010 American College of Rheumatology (ACR) diagnostic criteria for FMS; and voluntary participation in the study. On the other hand, patients were excluded from the study if they had a history of systemic disease (e.g., hypertension, diabetes, and cardiovascular or renal disease) or malignancy, an active infection, a history of neuromuscular, autoimmune, or psychiatric disorders, and pregnancy or breastfeeding.
In the first examination of FMS patients, the severity of pain was evaluated using a visual analog scale (VAS), and their functional status was determined using the Fibromyalgia Impact Questionnaire (FIQ). Generally, VAS is an easily applicable scale with global acceptance, which is used to measure the severity of pain. This scale, by converting values that cannot be measured numerically to numbers, facilitates an evaluation of subjective pain sensation and represents the pain severity. Pain is measured based on a 10-cm horizontal line, marked in equal sections of 1 cm, and the respondents are instructed to mark their level of pain on this line (0, no pain; 10, intolerable pain) (4, 5). The pain severity in the current study was classified as follows: 0 – 3, mild; 4 - 6, moderate; and 7 - 10, severe (7).
Moreover, the FIQ was used in this study to determine the quality of life and functional status of FMS patients. This scale, which is widely used for the evaluation of FMS patients, was developed by Burckhardt et al. (8). Also, the validity and reliability studies of the Turkish version of this questionnaire were conducted by Sarmer et al. (9). The FIQ generally consists of 10 items. The first item examines 11 activities of daily living (shopping, cooking, washing, vacuuming, making the bed, walking a few hundred meters, visiting friends/family, gardening, driving, and ascending/descending stairs), each scored from zero to three (0, always; 1, usually; 2, sometimes; and 3, never). The rest of the items examine well-being in the past week, inability to work, pain level, fatigue, morning stiffness, morning tiredness, anxiety, and depression.
The total score was determined for the first item of FIQ (11 activities, rated on a 0 - 3 scale); to normalize it, it was divided by 11 and then multiplied by 3.33. For the second item of the questionnaire, as the number of days the patient felt well was negatively proportional to the disease severity, the number of days stated by the patient was subtracted from seven and then multiplied by 1.43 to obtain a normalized value. The third item, which represented the number of days the patient was unable to work over the last week because of FMS symptoms, was normalized by multiplying by 1.43. The total score of the questionnaire was determined as the sum of the scores of items 4 - 10 plus the normalized scores of the first three items. The maximum total score was 100, with higher scores indicating a lower quality of life and functional status.
The magnetic resonance imaging (MRI), MRS, and DT imaging examinations were performed for the participants before treatment. The MRS and DT examinations of all the patients were performed on a 3 Tesla MRI unit (Magnetom Skyra, Siemens Healthcare, Germany) with a standard head coil. All MR examinations were first evaluated regarding pathologies, and patients with conventional MR examinations within normal limits were included in the study. After non-contrast brain MRI, single-voxel MRS of the thalamus was performed bilaterally at both a short echo (repetition time [TR], 2000 ms; echo time [TE], 30 ms) and a long echo (TR, 2000 ms; TE, 135 ms). Additionally, sagittal T1-weighted images and axial and coronal T2-weighted images were acquired for guiding volume selection. The right hand, as the dominant hand, was used for all the patients. The spectra were determined based on the point resolved spectroscopy (PRESS) method. Following water signal suppression with the chemical shift selective technique, spectroscopic data were obtained.
For the DT examination, T1-weighted 3D magnetization prepared rapid gradient echo (MPRAGE) imaging was performed for all the patients. The imaging parameters were as follows: TE, 92.0 ms; TR, 3700 ms; flip angle (FA), 7; and inversion time (TI), 1250 ms. On the ADC and FA maps, measurements were performed from the bilateral posterior limbs of internal capsule, thalami, corpus callosum genu, and splenium. The data were analyzed on a Leonardo workstation (Siemens, Forcheim, Germany), equipped with the manufacturer’s supplied software package.
Moreover, in the present study, the metabolite signals included N-acetylaspartate (NAA; 2.04 ppm), choline (Cho; 3.24 ppm), creatine (Cr; 3.05 ppm), glutamate-glutamine (Glx; 3.80 ppm), myo-inositol (MI; 3.58 ppm), and lactate (1.33 ppm). The ratio of these metabolites to Cr (Cho/Cr, NAA/Cr, Glx/Cr, and MI/Cr) was calculated in this study. Additionally, the metabolite concentrations of NAA, MI, Cho, and Cr were measured (Figure 1A - C).
A, Long-echo-time spectroscopy of a 26-year-old control woman; B, Short-echo-time spectroscopy of a 29-year-old patient (the N-acetylaspartate/Creatine (NAA/Cr) ratio was estimated at 1.84 with an increasing trend); C, Diffusion tensor (DT) color fractional anisotropy (FA) map of a 31-year-old female patient.
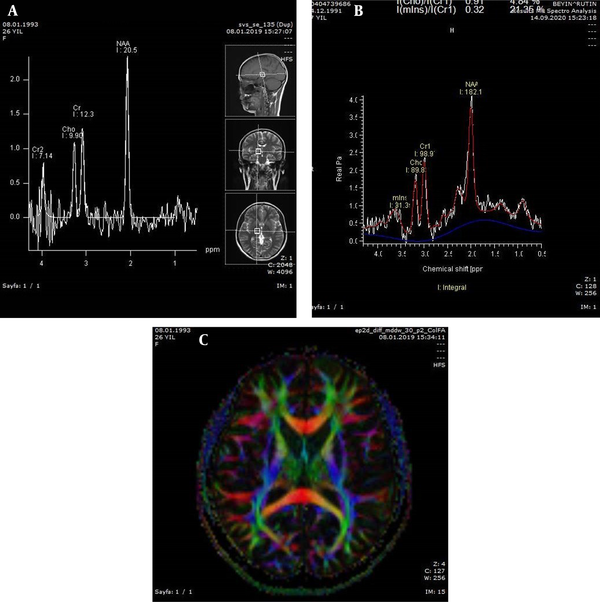
The collected data were statistically analyzed in IBM SPSS Version 20.0 (IBM Corp. Released 2011. IBM SPSS Statistics for Windows, Version 20.0. Armonk, NY: IBM Corp.). The results are presented as mean ± standard deviation (SD), median, minimum and maximum, or number (n) and percentage (%). The normal distribution of continuous variables was assessed using Shapiro-Wilk test when the sample size was < 50 and using Kolmogorov-Smirnov test when the sample size was ≥ 50. For comparison of two independent groups of data, independent samples t-test was performed when the data had a normal distribution, while Mann-Whitney U test was performed when the data did not have a normal distribution. On the other hand, for comparison of two dependent groups of data, paired samples t-test was used when the normal distribution conditions were met, while Wilcoxon test was applied when the data did not show a normal distribution. Moreover, for comparison of two groups of quantitative data, Pearson’s correlation test was performed when the data had a normal distribution; otherwise, Spearman’s correlation test was applied. Only statistically significant results of these tests are presented in this article. A P-value less than 0.05 was considered statistically significant.
4. Results
The conventional MRI findings of the participants were morphologically within normal limits. The mean age of the patients was 39.1 ± 8.6 years (range, 24 - 55 years). A significant positive correlation was found between the VAS and FIQ scores (r = 0.710, P < 0.001). A moderate positive correlation was also found between the left thalamus MI/Cr ratio and the scores of VAS (r = 0.404, P = 0.033) and FIQ (r = 0.390, P = 0.040). Besides, a moderate positive correlation was found between the FIQ score and the FA value of the right posterior limb of internal capsule (r = 0.404, P = 0.033). On the other hand, a statistically significant moderate negative correlation was found between the corpus callosum genu ADC value and the VAS score (r = -0.493, P = 0.008). Also, a statistically significant moderate negative correlation was observed between the left thalamus NAA/Cho ratio and the FIQ score (r = -0.437, P = 0.020) (Table 1).
Significant Correlation Coefficients of Visual Analog Scale (VAS) Scores with Magnetic Resonance Spectroscopy (MRS) Values of Metabolites a
Spearman’s rho | VAS | FIQ |
---|---|---|
MI/Cr ratio | ||
R | 0.404* | 0.390* |
P | 0.033 | 0.040 |
N | 28 | 28 |
MI | ||
R | 0.332 | 0.518** |
P | 0.084 | 0.005 |
N | 28 | 28 |
Left thalamus, NAA/Cho | ||
R | -0.045 | -0.437 |
P | 0.821 | 0.020 |
N | 28 | 28 |
RICP FA | ||
R | 0.305 | 0.404* |
P | 0.114 | 0.033 |
N | 28 | 28 |
A significant association was found between the mean values of NAA/Cr ratio, glutamate (Glu)/Cr ratio, NAA, Cho, and Glu and the ADC value of the left and right thalami. The mean NAA/Cr ratio was 1.27 ± 0.23 in the right thalamus and 1.48 ± 0.25 in the left thalamus (P = 0.002), and the mean Glu/Cr ratio was 1.44 ± 0.34 in the right thalamus and 1.66 ± 0.44 in the left thalamus (P = 0.042). N-acetylaspartate was 181.21 ± 37.30 in the right thalamus and 205.20 ± 37.11 in the left thalamus (P = 0.019); long echo time NAA was 42.47 ± 11.60 in the right thalamus and 51.93 ± 7.83 in the left thalamus (P < 0.001); Cho was 36.02 ± 7.56 in the right thalamus and 41.27 ± 9.11 in the left thalamus (P = 0.022); and Glu was 133.69 ± 37.90 in the right thalamus and 160.38 ± 38.21 in the left thalamus (P = 0.004). Based on the measurements, the thalamus ADC value was 737.51 ± 29.56 in the right thalamus and 755.41 ± 29.71 in the left thalamus (P = 0.053) (Table 2).
Variables | Right-left | t, z | P-value | |||||||
---|---|---|---|---|---|---|---|---|---|---|
R | L | |||||||||
Mean ± standard deviation | Median | Minimum | Maximum | Mean ± standard deviation | Median | Minimum | Maximum | |||
NAA/Cr | 1.27 ± 0.23 | 1.32 | 0.69 | 1.62 | 1.48 ± 0.25 | 1.45 | 0.84 | 2.05 | -3.195 | 0.002 |
NAA/Cho | 1.18 ± 0.28 | 1.21 | 0.20 | 1.61 | 1.31 ± 0.30 | 1.26 | 0.90 | 2.34 | -1.049 | 0.294 |
Glx/Cr | 1.46 ± 0.51 | 1.53 | 0.65 | 2.35 | 1.20 ± 0.49 | 1.25 | 0.29 | 2.20 | 2.002 | 0.050 |
Glu/Cr | 1.44 ± 0.34 | 1.39 | 0.79 | 2.30 | 1.66 ± 0.44 | 1.63 | 1.06 | 2.73 | -2.078 | 0.042 |
MI/Cr | 0.74 ± 0.30 | 0.82 | 0.16 | 1.20 | 0.86 ± 0.31 | 0.88 | 0.21 | 1.51 | -1.486 | 0.143 |
Cho/Cr | 1.17 ± 0.48 | 1.12 | 0.79 | 3.47 | 1.17 ± 0.21 | 1.23 | 0.70 | 1.58 | -1.549 | 0.121 |
NAA | 42.47 ± 11.60 | 3.23 | 68.08 | 51.93 | 7.83 ± 49.76 | 38.75 | 70.38 | -3.597 | 0.000 | 0.000 |
Cr | 33.19 ± 8.02 | 4.65 | 47.47 | 36.01 | 7.34 ± 34.57 | 26.44 | 57.91 | -1.090 | 0.276 | 0.276 |
Cho | 36.02 ± 7.56 | 16.14 | 51.66 | 41.27 | 9.11 ± 41.36 | 18.48 | 63.09 | -2.350 | 0.022 | 0.022 |
Glx | 134.16 ± 45.97 | 55.09 | 218.15 | 127.38 | 42.56 ± 126.21 | 19.81 | 238.04 | 0.573 | 0.569 | 0.569 |
Glu | 133.69 ± 37.90 | 83.81 | 253.68 | 160.38 | 38.21 ± 156.31 | 96.12 | 243.75 | -2.892 | 0.004 | 0.004 |
MI | 69.57 ± 31.61 | 11.44 | 157.53 | 80.06 | 30.00 ± 84.05 | 15.43 | 138.51 | -1.273 | 0.209 | 0.209 |
RICP FA | 728.13 ± 53.18 | 647.00 | 851.00 | 710.39 | 53.43 ± 720.88 | 602.33 | 796.33 | 1.245 | 0.218 | 0.218 |
RT FA | 294.11 ± 33.99 | 236.50 | 390.00 | 281.30 | 34.57 ± 288.17 | 198.50 | 334.50 | -0.869 | 0.385 | 0.385 |
RICP ADC | 704.53 ± 28.59 | 619.50 | 757.00 | 719.86 | 38.77 ± 710.34 | 674.50 | 820.00 | -0.885 | 0.376 | 0.376 |
RT ADC | 737.51 ± 29.56 | 699.00 | 786.67 | 755.41 | 29.71 ± 746.75 | 717.67 | 814.00 | -1.934 | 0.053 | 0.053 |
5. Discussion
Several studies have suggested that widespread pain disorder in FMS originates from a pain processing disorder in the central nervous system (4, 10-13). In a study by Sundgren et al., where the ADC and FA values were compared with the symptom severity of FMS patients, there were basic differences between these patients and the control group; these differences were mostly observed in the right thalamic region (14). Also, in the FMS group, the differences were statistically greater in patients with a more severe clinical pain, and the relationship of these values with disease severity depended on other clinical parameters, but not significantly. These findings revealed that there are minor abnormalities in the neuronal functioning of pain processing regions of the brain in FMS and that the thalamus plays an important role in pain processing in these patients (14).
In the current study, a positive correlation was observed between clinical pain severity and the FA value of the internal capsule posterior limb; in other words, with an increase in the clinical pain severity, the FA values increased, as well. On the other hand, a negative correlation was found between the VAS score and the corpus callosum genu ADC value; therefore, as pain increased, the ADC value decreased; this finding was speculated to be associated with microstructural changes induced by pain in the white matter structure. Additionally, Lutz et al. investigated the grey and white matter abnormalities in FMS patients and showed that areas of the brain that are known to be functionally linked to the main symptoms of FMS were related to significant changes in the brain microstructure (15). They also indicated a relationship between the degree of microstructural tissue change and the intensity of several main symptoms of FMS (15).
Previous studies examining brain activation related to pain revealed that in healthy individuals, acute pain causes a stimulated response in several brain structures, such as the thalamus, primary and secondary somatosensory cortex, anterior cingulate cortex, and prefrontal cortex (16). In the current study, the spectroscopic examinations of the right and left thalami showed a positive correlation between MI and MI/Cr values and the FIQ score. On MRS, a significant correlation was observed between pain and the concentration of Glu in the insula. Generally, Glu plays a major excitatory role in the pain pathway, which is extremely important in understanding the pain processes of FMS (17). In the present study, the Glu level in the right thalamus was significantly higher than the left thalamus.
Moreover, in a study by Wood et al., hippocampal metabolite abnormalities were investigated in FMS (18). A significant negative correlation was found between a reduced NAA/Cr ratio, as a marker of neuronal integrity in the right hippocampus, and the FIQ score, representing an index of irregularity in basic characteristics, such as physical function, work status, depression, anxiety, sleep, pain, fatigue, and well-being (18). Besides, in a study of chronic lower back pain, Levins et al. found reduced NAA in the dorsolateral prefrontal cortex and anterior insula, along with a reduction in NAA, Glu, and MI in the anterior cingulate cortex; these findings suggested a decline in neuronal vitality (19).
While the prevalence of gamma-aminobutyric acid (GABA), as an inhibitor neurotransmitter, reduces in the anterior insula of FMS patients, an increase has been reported in the stimulation of neurotransmitter glutamate in the amygdala, posterior cingulate cortex, and ventrolateral prefrontal cortex, suggesting the overactivation of neural activity in the limbic region. In patients with spinal injuries and chronic pain, low neuronal metabolite levels (NAA and Glu) suggesting neuroinflammation, together with high glial metabolite levels (MI and Cho), have been found in the anterior cingulate cortex. Also, reduced NAA levels indicating reduced inhibitory neuron function in the thalamus, as a potential mediator of chronic pain, have been reported in the thalamus of patients with complex pain syndrome, as well as the thalamus of diabetic patients with chronic neuropathic pain (19).
The limitations of this study include the low number of patients and the lack of a control group. In conclusion, the results of this study suggested that metabolites contribute to the central pain mechanisms of FMS, as a chronic pain syndrome. These changes were also correlated with the severity of clinical symptoms. By controlling for the ratio of these metabolites in the brain, pain control can be effectively achieved in the future. However, there is a need for more extensive studies in the future to obtain further information related to the pathogenesis of this disease.
References
-
1.
Wolfe F, Ross K, Anderson J, Russell IJ, Hebert L. The prevalence and characteristics of fibromyalgia in the general population. Arthritis Rheum. 1995;38(1):19-28. [PubMed ID: 7818567]. https://doi.org/10.1002/art.1780380104.
-
2.
Lautenbacher S, Rollman GB, McCain GA. Multi-method assessment of experimental and clinical pain in patients with fibromyalgia. Pain. 1994;59(1):45-53. https://doi.org/10.1016/0304-3959(94)90046-9.
-
3.
Kosek E, Hansson P. Modulatory influence on somatosensory perception from vibration and heterotopic noxious conditioning stimulation (HNCS) in fibromyalgia patients and healthy subjects. Pain. 1997;70(1):41-51. https://doi.org/10.1016/s0304-3959(96)03295-2.
-
4.
Petzke F, Clauw DJ, Ambrose K, Khine A, Gracely RH. Increased pain sensitivity in fibromyalgia: effects of stimulus type and mode of presentation. Pain. 2003;105(3):403-13. https://doi.org/10.1016/s0304-3959(03)00204-5.
-
5.
Brandao LA, Shiroishi MS, Law M. Brain tumors: a multimodality approach with diffusion-weighted imaging, diffusion tensor imaging, magnetic resonance spectroscopy, dynamic susceptibility contrast and dynamic contrast-enhanced magnetic resonance imaging. Magn Reson Imaging Clin N Am. 2013;21(2):199-239. [PubMed ID: 23642551]. https://doi.org/10.1016/j.mric.2013.02.003.
-
6.
Ertekİn E, Özsunar Dayanir Y. [Advanced MRI Techniques in Brain Tumors]. Turkiye Klinikleri J Radiol-Special Topics. 2017;10(2):129-39. Turkish.
-
7.
Leith S, Wheatley RG, Jackson IJ, Madej TH, Hunter D. Extradural infusion analgesia for postoperative pain relief. Br J Anaesth. 1994;73(4):552-8. [PubMed ID: 7999504]. https://doi.org/10.1093/bja/73.4.552.
-
8.
Burckhardt CS, Clark SR, Bennett RM. The fibromyalgia impact questionnaire: Development and validation. J Rheumatol. 1991;18(5):728-33. [PubMed ID: 1865419].
-
9.
Sarmer S, Ergin S, Yavuzer G. The validity and reliability of the Turkish version of the Fibromyalgia Impact Questionnaire. Rheumatol Int. 2000;20(1):9-12. [PubMed ID: 11149662]. https://doi.org/10.1007/s002960000077.
-
10.
Arroyo JF, Cohen ML. Abnormal responses to electrocutaneous stimulation in fibromyalgia. J Rheumatol. 1993;20(11):1925-31. [PubMed ID: 7848390].
-
11.
Lorenz J. Hyperalgesia or hypervigilance? An evoked potential approach to the study of fibromyalgia syndrome. Z Rheumatol. 1998;57 Suppl 2:19-22. [PubMed ID: 10025076]. https://doi.org/10.1007/s003930050228.
-
12.
Petrou M, Foerster B, Fan X. Two D-CSI MR spectroscopy in the evaluation of fibromyalgia patients: a prospective study comparing fi- bromyalgia patients with normal healthy controls. XXX ESNR Annual Meeting, 14th Advanced Course in Diagnostic Neuroradiology. 15-18 September; Barcelona, Spain. European Society of Neuroradiology; 2005.
-
13.
Petrou M, Foerster B, Fan X. Metabolite abnormalities in potential pain processing brain regions of fibromyalgia patients using MR spectroscopy. European Congress of Radiology. 3-7 March; Vienna, Austria. HealthManagement; 2006.
-
14.
Sundgren PC, Petrou M, Harris RE, Fan X, Foerster B, Mehrotra N, et al. Diffusion-weighted and diffusion tensor imaging in fibromyalgia patients: a prospective study of whole brain diffusivity, apparent diffusion coefficient, and fraction anisotropy in different regions of the brain and correlation with symptom severity. Acad Radiol. 2007;14(7):839-46. [PubMed ID: 17574134]. https://doi.org/10.1016/j.acra.2007.03.015.
-
15.
Lutz J, Jager L, de Quervain D, Krauseneck T, Padberg F, Wichnalek M, et al. White and gray matter abnormalities in the brain of patients with fibromyalgia: a diffusion-tensor and volumetric imaging study. Arthritis Rheum. 2008;58(12):3960-9. [PubMed ID: 19035484]. https://doi.org/10.1002/art.24070.
-
16.
No Authors Listed. Pain imaging. Washington, USA: IASP Press; 2000. p. 1-248.
-
17.
Staud R. Brain imaging in fibromyalgia syndrome. Clin Exp Rheumatol. 2011;29(6 Suppl 69):S109-17. [PubMed ID: 22243558].
-
18.
Wood PB, Ledbetter CR, Glabus MF, Broadwell LK, Patterson J2. Hippocampal metabolite abnormalities in fibromyalgia: correlation with clinical features. J Pain. 2009;10(1):47-52. [PubMed ID: 18771960]. https://doi.org/10.1016/j.jpain.2008.07.003.
-
19.
Levins KJ, Drago T, Roman E, Martin A, King R, Murphy P, et al. Magnetic resonance spectroscopy across chronic pain disorders: a systematic review protocol synthesising anatomical and metabolite findings in chronic pain patients. Syst Rev. 2019;8(1):338. [PubMed ID: 31882014]. [PubMed Central ID: PMC6935150]. https://doi.org/10.1186/s13643-019-1256-5.