Abstract
Background:
In the past several years, emerging imaging techniques, such as computed tomography angiography (CTA), Doppler ultrasound, and magnetic resonance imaging (MRI), have been used for investigating the anatomy and perfusion of perforator complexes. Preoperative CTA can provide explicit information on perforator flaps, which not only helps surgeons to evaluate the optimal design of flaps with respect to the lateral circumflex femoral artery (LCFA) territory and the concomitant venous drainage pattern, but also reduces postoperative complications and secondary operation rates.Objectives:
This study aimed to evaluate the clinical feasibility of a low-dose CTA protocol with adaptive statistical iterative reconstruction (ASIR) for patients scheduled for anterolateral thigh perforator flap (ALTPF) surgery.Patients and Methods:
This prospective randomized controlled trial was conducted in a single institution from August 2016 to July 2017. A total of 60 inpatients scheduled for the ALTPF surgery were randomly allocated into three groups (n = 20 per group): Group A, filtered back projection (FBP) reconstruction with a tube voltage of 120 kVp; group B, ASIR with a tube voltage of 100 kVp; and group C, ASIR with a tube voltage of 80 kVp. The vessel attenuation value, signal-to-noise ratio (SNR), contrast-to-noise ratio (CNR), and figure of merit (FOM) were compared between the three groups. Additionally, a four-point Likert scale was used to evaluate the subjective quality of images. The scan length, dose-length product (DLP), CT dose index (CTDI), and effective dose (ED) were also recorded and compared.Results:
The age, sex, and body mass index (BMI) were not significantly different between the three groups (P > 0.05 for all). The FOM of images in the three segments was significantly higher in group C compared to the other two groups (P < 0.001 for all). The results of subjective evaluation revealed no poor-quality images, and the Likert score did not significantly differ between the groups. Compared to group A and group B, significant reductions were observed in CTDI, DLP, and ED in group C.Conclusion:
The 80-kVp protocol with ASIR not only reduced the radiation dose, but also exhibited lower performance compared to the 120-kVp protocol with FBP and the 80-kVp protocol with ASIR; it also yielded relatively satisfactory image quality.Keywords
1. Background
Since the introduction of free anterolateral thigh perforator flap (ALTPF) by Koshima et al. for reconstruction purposes in 1993 (1), it has become a versatile technique, showing promising clinical efficacy in neck and head soft-tissue reconstructive microsurgeries due to the large blood supply area and limited harmful effects (2). Therefore, harvesting the lateral circumflex femoral artery (LCFA) perforator flaps depends on the surgeon’s years of experience and operative skills. In the past, even highly skilled and experienced surgeons could not always harvest the vessels accurately (3), which restrained the clinical application of this technique to some extent.
Computed tomography angiography (CTA) is considered the gold standard for navigation of deep inferior epigastric perforator flaps to increase the safety and precision of surgery (4). However, the scanned area is large, and patients are usually exposed to high-dose radiation because of the large scale of lower limb vessels. To overcome these shortcomings, application of a low tube Kilovoltage peak (kVp) has been regarded as a reliable approach to reduce the radiation dose; nevertheless, it inevitably compromises the image quality (5). Therefore, a CTA protocol, which can reduce the radiation dose while maintaining the image quality, is warranted. An emerging technique, called adaptive statistical iterative reconstruction (ASIR), which is an important iterative reconstruction (IR) technique, has been widely studied for reducing the image noise and improving the image quality (6). However, application of ASIR with a low tube Kilovoltage peak (kVp) is limited in ALTPF.
2. Objectives
This study aimed to assess the clinical feasibility of a low-dose CTA protocol for ALTPF, using a combined radiation dose-saving strategy, involving ASIR and a tube voltage of 80 kVp.
3. Patients and Methods
3.1. Participants
This prospective, randomized controlled trial was conducted on consecutively selected patients, who were scheduled for the ALTPF surgery and CTA examination of both lower limb arterioles in Wuxi No.9 People's Hospital, affiliated to Soochow University (Suzhou, China) from August 2016 to July 2017. The inclusion criteria were as follows: (1) age range of 15 - 70 years; (2) body mass index (BMI) of 18 - 27 kg/m2; and (3) having indications for surgery. On the other hand, the exclusion criteria were as follows: (1) allergy to the contrast agent; (2) diagnosis of serious internal diseases, such as heart and kidney diseases; and (3) having contraindications for ALTPF transplantation. The patients were randomized into three groups using a random number generator (Figure 1).
The process of patient enrollment and grouping
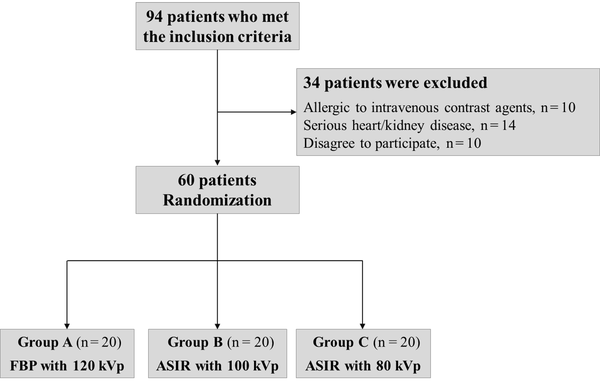
This study was reviewed and approved by the Ethics Committee of Wuxi No.9 People’s Hospital (No. 201509) and performed in accordance with the ethical standards of the Declaration of Helsinki. Informed consent was also obtained from all individual participants. This randomized controlled trial was registered in the Chinese Clinical Trial Registry (ChiCTR2300068024).
3.2. CT Scan Protocol
All CT examinations were performed using a GE Optima CT660 128-Row Multislice Spiral CT Scanner (Lightspeed Ultra, GE Medical Systems, Milwaukee, WI, USA). The scanning scope ranged from a 5-cm distance from the upper side of the anterior superior iliac spine to a 10-cm distance from the inferior pole of the patellar tendon. The contrast solution was Omnipaque, injected at 350 mgI/mL (standard, 1.8 mL/kg, dosage < 120 mL). All the patients were scanned in a manually triggered sequential scanning mode. The regions of interest (ROI size, 10 mm²) were located on the lower side of the abdominal aorta. When the CT attention value of the ROI reached 150 Hounsfield units (HU), scanning was triggered. After successful scanning, data were uploaded to AW Server 2.0 3D Image Workstation for further processing.
There were three imaging protocols in this study. Group A received the standard radiation dose with a fixed tube voltage of 120 kVp and automatic tube current modulation. The images in group A were reconstructed based on the filtered back projection (FBP) algorithm. In group B, a tube voltage of 100 kVp was fixed with automatic tube current modulation, and the images were reconstructed using the ASIR algorithm. In group C, a lower tube voltage (80 kVp) was fixed with automatic tube current modulation, and the ASIR algorithm was applied for images reconstruction. The layer thickness was 5.0 mm, the pitch was 1.5, and the image reconstruction layer thickness was 0.625 mm.
3.3. Image Analysis
The mean vessel/tissue attenuation value was calculated by averaging all mean attenuation values measured in three ROIs per patient. The background image noise was defined as the standard deviation (SD) of CT value measured in the adjacent muscle (7). Next, to quantitatively evaluate the image quality, the signal-to-noise ratio (SNR) (7), contrast-to-noise ratio (CNR) (8), and figure of merit (FOM) were calculated for radiation exposure adjustments in the three protocols (9). The SNR, CNR, and FOM were calculated as follows:
Finally, a subjective image analysis was performed blindly by two independent experienced senior radiologists. The image reviewers initially received standard instructions and evaluated the general quality of images according to a four-point Likert scale (10).
3.4. Measurement of Radiation Dose
The scanning length and CT dose index (CTDI) were recorded for each group. The dose-length product (DLP) values were also calculated by multiplying the CTDI and scanning length. The ED was calculated according to the DLP values, based on the following equation:
where κ equals 0.019.
3.5. Statistical Analysis
Continuous variables are expressed as mean and SD, and categorical variables are expressed as absolute number and percentage. The inter-observer variability between two independent observers was examined based on Cohen’s kappa coefficient for the evaluation of CT image quality. For normally distributed variables, comparisons were performed between the three groups using one-way analysis of variance (ANOVA), followed by Tukey’s post-hoc test. To compare non-normally distributed variables and ranked data between the three groups, Kruskal-Wallis test was used. Chi-square or Fisher’s exact test was also applied for comparing proportions between the groups. A two-tailed P-value less than 0.05 was considered statistically significant.
4. Results
4.1. Characteristics of the Study Population
The average age of the study population was 38 years (SD, 12.10), and their mean BMI was 22.19 kg/m2 (SD, 1.78). The characteristics of the patients in each group are summarized in Table 1. There was no significant difference in terms of age, sex, and BMI between the three groups (P > 0.05 for all).
Characteristics of the Study Population in the Groups a
Group A | Group B | Group C | P-value | |
---|---|---|---|---|
Number of patients | 20 | 20 | 20 | |
Age, y | 36.6 ± 14.47 | 38.90 ± 10.11 | 39.35 ± 11.76 | 0.749 |
Sex (male/female) | 11/9 | 13/7 | 12/8 | 0.812 |
BMI, kg/m2 | 22.08 ± 1.30 | 22.29 ± 1.90 | 22.20 ± 2.13 | 0.943 |
4.2. CT Attenuation Values and Image Noise
As shown in Table 2, significant differences were observed in the vessel CT values between the three groups (P < 0.001 for all), with group C (ASIR-80 kVp) showing the highest values. Except for the CT values in the tissue around the femoral artery (FA) (P = 0.493), the CT values of background tissues were significantly different between the three groups in both the iliac artery (IA) and the descending branch of LCFA (P < 0.05). The image noise was also evaluated in the three groups in various positions, and no significant differences were found between the three protocols regarding image noise at FA and the descending branch of LCFA, although a relatively higher noise was observed in the IA in group B (P = 0.046).
Comparison of CT Values Measured in Different Arterial Segments Between the Groups a
Region | Parameter | Group A | Group B | Group C | P-value |
---|---|---|---|---|---|
IA | Vessel (HU) | 288.70 ± 37.79 | 323.25 ± 35.64* | 494.42 ± 62.09# | < 0.001 |
Tissue (HU) | 61.27 ± 3.19 | 65.93 ± 14.76* | 70.22 ± 6.08# | < 0.001 | |
Image noise (HU) | 4.84 ± 6.99 | 8.08 ± 10.58* | 6.89 ± 6.50# | 0.046 | |
FA | Vessel (HU) | 316.18 ± 41.00 | 334.37 ± 27.67* | 512.54 ± 68.31# | < 0.001 |
Tissue (HU) | 55.76 ± 8.31 | 57.61 ± 6.35 | 61.28 ± 13.49# | 0.493 | |
Image noise (HU) | 6.89 ± 10.98 | 6.20 ± 6.51 | 4.18 ± 2.28# | 0.634 | |
Descending branch of LCFA | Vessel (HU) | 285.66 ± 36.35 | 303.33 ± 47.92* | 514.90 ± 68.77# | < 0.001 |
Tissue (HU) | 65.88 ± 5.53 | 62.99 ± 6.45* | 70.98 ± 9.81# | 0.005 | |
Image noise (HU) | 5.93 ± 10.08 | 9.71 ± 11.78* | 6.94 ± 7.73 | 0.469 |
4.3. Objective Quality Evaluation
The representative images of the anterolateral thigh perforator of different groups are shown in Figure 2. Besides, the objective quality of the images was examined from different aspects. The SNR, CNR, and FOM of each image were also calculated and compared. As shown in Figure 3A and B, there was no significant difference regarding SNR and CNR between the three groups in the three evaluated segments. However, group C showed a higher CNR in the descending branch of LCFA compared to group B (group C, 125.36 ± 102.30 vs. group B, 70.51 ± 55.87; P = 0.064). Since SNR and CNR may be influenced by effective radiation, the FOM was also calculated to adjust for the radiation dose and to compare the image quality. As shown in Figure 3C, the FOM of images was significantly higher in group C compared to the other two groups in all three evaluated segments (P < 0.001 for all).
Sample images of the anterolateral thigh perforator (ALTPF) in different groups. A, B, and C, Show representative images of ALTPF measurements in a patient from group A (filtered back projection [FBP] with 120-kVp tube voltage), a patient from group B (adaptive statistical iterative reconstruction [ASIR] with 100-kVp tube voltage), and a patient from group C (ASIR with 80-kVp tube voltage). Yellow arrows indicate the perforator in each group. The images show an overall similar quality in different scan protocols.
![Sample images of the anterolateral thigh perforator (ALTPF) in different groups. A, B, and C, Show representative images of ALTPF measurements in a patient from group A (filtered back projection [FBP] with 120-kVp tube voltage), a patient from group B (adaptive statistical iterative reconstruction [ASIR] with 100-kVp tube voltage), and a patient from group C (ASIR with 80-kVp tube voltage). Yellow arrows indicate the perforator in each group. The images show an overall similar quality in different scan protocols. Sample images of the anterolateral thigh perforator (ALTPF) in different groups. A, B, and C, Show representative images of ALTPF measurements in a patient from group A (filtered back projection [FBP] with 120-kVp tube voltage), a patient from group B (adaptive statistical iterative reconstruction [ASIR] with 100-kVp tube voltage), and a patient from group C (ASIR with 80-kVp tube voltage). Yellow arrows indicate the perforator in each group. The images show an overall similar quality in different scan protocols.](https://services.brieflands.com/cdn/serve/31642/b0fba8a7cd9e3567d7e08141154c6fe816e02205/iranjradiol-20-1-129458-i002-preview.png)
Comparison of objective image quality. A, Comparison of signal-to-noise ratio (SNR) in different arterial segments between the groups. B, Comparison of contrast-to-noise ratio (CNR) values in different arterial segments between the groups. C, Comparison of figure of merit (FOM) in different arterial segments between the groups. Comparison of the three groups is performed by one-way analysis of variance (ANOVA), followed by Tukey’s post-hoc test (∗P < 0.05, ∗∗P < 0.01, ∗∗∗P < 0.001).
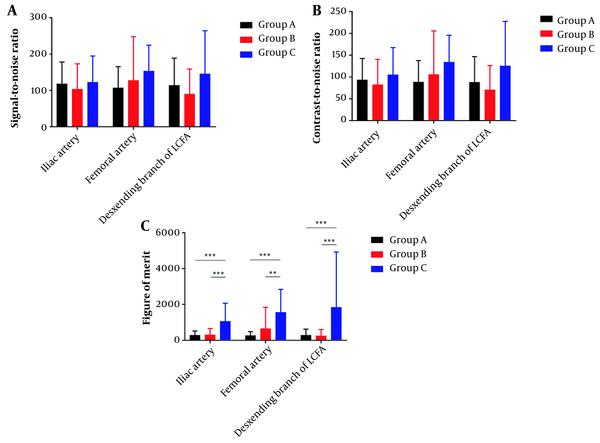
4.4. Subjective Quality Evaluation
As summarized in Table 3, there were no poor-quality images in the three groups. The Likert score for the FBP, ASIR-100 kVp, and ASIR-80 kVp protocols showed no significant differences in any of the segments (P > 0.05 for all); therefore, the ASIR-80 kVp protocol is not inferior to the other two protocols in terms of the overall image quality. Moreover, substantial agreement was found between the two observers regarding the score of image quality (kappa coefficient, 0.76), indicating moderate consistency.
Distribution of Different Subjective Scores of Image Quality in Different Groups a
Parameters | Group A, No. (%) | Group B, No. (%) | Group C, No. (%) | P-value |
---|---|---|---|---|
IA | ||||
Excellent (score 4) | 16 (80) | 15 (75) | 12 (60) | 0.31 (0.310) |
Good (score 3) | 4 (20) | 5 (25) | 7 (30) | |
Moderate (score 2) | 0 (0) | 0 (0) | 1 (5) | |
Poor (score 1) | 0 (0) | 0 (0) | 0 (0) | |
FA | ||||
Excellent (score 41) | 15 (75) | 13 (65) | 10 (50) | 0.19 (0.189) |
Good (score 3) | 5 (25) | 7 (35) | 8 (40) | |
Moderate (score 2) | 0 (0) | 0 (0) | 2 (10) | |
Poor (score 1) | 0 (0) | 0 (0) | 0 (0) | |
Descending branch of LCFA | ||||
Excellent (score 4) | 17 (85) | 14 (70) | 12 (60) | 0.17 (0.174) |
Good (score 3) | 3 (15) | 6 (30) | 6 (30) | |
Moderate (score 2) | 0 (0) | 0 (0) | 2 (10) | |
Poor (score 1) | 0 (0) | 0 (0) | 0 (0) |
4.5. Radiation Dose Comparison
According to Table 4, there was no significant difference in the scanning length between the three groups. However, when the tube voltage decreased from 120 kVp to 100 and 80 kVp, the ED significantly decreased by 16.45% and 64.99%, respectively. Compared to group A and group B, significant reductions were observed in group C regarding CTDI (group C: 11.95 ± 1.02 mGy vs. group A: 35.18 ± 3.40 mGy and group B: 28.31 ± 2.18 mGy), DLP (group C: 729.45 ± 33.22 mGy/cm vs. group A: 22083.64 ± 170.43 mGy/cm and group B: 1720.15 ± 121.78 mGy/cm), and ED (group C: 13.86 ± 0.63 mSv vs. group A: 39.59 ± 3.24 mSv and group B: 32.68 ± 2.31 mSv). It can be concluded that the 80-kVp CTA protocol with ASIR could significantly reduce the radiation dose for candidates of ALTPF surgery in the preoperative localization of target vessels.
Comparison of Radiation Dose Between the Groups a
Radiation parameters | Group A | Group B | Group C | P-value |
---|---|---|---|---|
Scan length, cm | 59.40 ± 3.39 | 61.25 ± 3.97 | 61.25 ± 3.77 | 0.184 |
CTDI, mGy | 35.18 ± 3.40 | 28.31 ± 2.18* | 11.95 ± 1.02# | < 0.001 |
DLP, mGy/cm | 2083.64 ± 170.43 | 1720.15 ± 121.78* | 729.45 ± 33.22# | < 0.001 |
ED, mSv | 39.59 ± 3.24 | 32.68 ± 2.31* | 13.86 ± 0.63# | < 0.001 |
5. Discussion
In this study, a new protocol was evaluated for the preoperative evaluation of lower limb arteries in patients seeking the ALTPF surgery. Although by decreasing the tube voltage, the image noise might slightly increase, the 80-kVp protocol with ASIR yielded a lower image quality, regardless of the type of evaluation (objective or subjective). Besides, the 80-kVp protocol with ASIR could significantly reduce the radiation dose for patients and improve the FOM of the images.
Over the past years, CTA has been widely applied for analyzing the source, territory, and variations of lower limb arteries for preparation of ALTPF surgery (11). However, the large scale of the scanning area raises concerns regarding exposure to high levels of ionizing radiation, which remains an important issue in clinical imaging (12). Major efforts have been made to reduce the radiation dose while maintaining the image quality for diagnostic purposes. Generally, radiologists try to reduce the radiation dose by decreasing the tube voltage or current (13), although compared to the former, the latter is less efficient. Since the radiation dose is positively correlated with the square of voltage, a decrease in tube voltage can lead to remarkable dose reductions; meanwhile, the image quality may be affected by the increased noise. Based on the current results, by decreasing the voltage, the background image noise increased, which is consistent with previous research (14).
Image reconstruction algorithms play a critical role in image quality. The iterative reconstruction, as a noise reduction technique, facilitates exposure to a lower radiation dose (15). Compared to FBP reconstruction, ASIR ensures the image quality, while reducing the radiation dose (16). As a hybrid IR algorithm, ASIR yields blended FBP and IR images, with IR percentages of 0 to 100%, where the percentage represents the contribution of ASIR to the final image (5, 10). The present results showed the increasing trend of CNR in the descending branch of LCFA in group C, although there was no significant difference in SNR between the three groups. The application of ASIR technique increased the FOM of images, with group C showing the highest FOM in all three arterial sections.
Considering the radiation dose, lower exposure was achieved in group B and group C in the present study. When the tube voltage decreased from 120 kVp to 100 and 80 kVp, the ED significantly decreased by 16.45% and 64.99%, respectively. It is worth mentioning that the subjective image quality of the three groups was not significantly different, and there were no poor-quality images in any of the groups. Based on these results, a small reduction in voltage might not exert negative effects on the image quality when combined with the ASIR algorithm.
In conclusion, because of variability in the LCFA, operation mainly relies on the surgeon’s experience and skills. However, if there is an unexpected variation in the artery, the operation time will be prolonged, which may increase the risk of complications. The preoperative CTA detection can help surgeons to accurately localize the perforator and optimally design the operation. In the present study, the concordance rate of perforator detection between the CTA and surgical confirmation was as high as 96.7% in group C. Accordingly, application of a low-dose CTA protocol with ASIR for lower limb arterial examinations can effectively guide the ALTPF surgery.
References
-
1.
Koshima I, Fukuda H, Yamamoto H, Moriguchi T, Soeda S, Ohta S. Free anterolateral thigh flaps for reconstruction of head and neck defects. Plast Reconstr Surg. 1993;92(3):421-8. discussion 429-30. [PubMed ID: 8341740].
-
2.
Song D, Pafitanis G, Pont LEP, Yang P, Koshima I, Zhang Y, et al. Chimeric thoracoacromial artery perforator flap for one-staged reconstruction of complex pharyngoesophageal defects: A single unit experience. Head Neck. 2018;40(2):302-11. [PubMed ID: 29024231]. https://doi.org/10.1002/hed.24962.
-
3.
Shen Y, Lu LG, Low DW, Zhou HH, Li J, Sun J. Perforator navigation using color Doppler ultrasound and three-dimensional reconstruction for preoperative planning of optimal lateral circumflex femoral artery system perforator flaps in head and neck reconstruction. J Plast Reconstr Aesthet Surg. 2019;72(6):990-9. [PubMed ID: 30880047]. https://doi.org/10.1016/j.bjps.2018.12.025.
-
4.
Keys KA, Louie O, Said HK, Neligan PC, Mathes DW. Clinical utility of CT angiography in DIEP breast reconstruction. J Plast Reconstr Aesthet Surg. 2013;66(3):e61-5. [PubMed ID: 23084649]. https://doi.org/10.1016/j.bjps.2012.09.025.
-
5.
Zhu Z, Zhao Y, Zhao X, Wang X, Yu W, Hu M, et al. Impact of preset and postset adaptive statistical iterative reconstruction-V on image quality in nonenhanced abdominal-pelvic CT on wide-detector revolution CT. Quant Imaging Med Surg. 2021;11(1):264-75. [PubMed ID: 33392027]. [PubMed Central ID: PMC7719942]. https://doi.org/10.21037/qims-19-945.
-
6.
Zhu Z, Zhao XM, Zhao YF, Wang XY, Zhou CW. Feasibility Study of Using Gemstone Spectral Imaging (GSI) and Adaptive Statistical Iterative Reconstruction (ASIR) for Reducing Radiation and Iodine Contrast Dose in Abdominal CT Patients with High BMI Values. PLoS One. 2015;10(6). e0129201. [PubMed ID: 26079259]. [PubMed Central ID: PMC4469609]. https://doi.org/10.1371/journal.pone.0129201.
-
7.
Bodelle B, Wichmann JL, Klotz N, Lehnert T, Vogl TJ, Luboldt W, et al. Seventy kilovolt ultra-low dose CT of the paranasal sinus: first clinical results. Clin Radiol. 2015;70(7):711-5. [PubMed ID: 25912259]. https://doi.org/10.1016/j.crad.2015.03.002.
-
8.
Cordts I, Deschauer M, Lingor P, Burian E, Baum T, Zimmer C, et al. Radiation dose reduction for CT-guided intrathecal nusinersen administration in adult patients with spinal muscular atrophy. Sci Rep. 2020;10(1):3406. [PubMed ID: 32099042]. [PubMed Central ID: PMC7042284]. https://doi.org/10.1038/s41598-020-60240-x.
-
9.
Utsunomiya D, Oda S, Funama Y, Awai K, Nakaura T, Yanaga Y, et al. Comparison of standard- and low-tube voltage MDCT angiography in patients with peripheral arterial disease. Eur Radiol. 2010;20(11):2758-65. [PubMed ID: 20571804]. https://doi.org/10.1007/s00330-010-1841-4.
-
10.
Lim K, Kwon H, Cho J, Oh J, Yoon S, Kang M, et al. Initial phantom study comparing image quality in computed tomography using adaptive statistical iterative reconstruction and new adaptive statistical iterative reconstruction v. J Comput Assist Tomogr. 2015;39(3):443-8. [PubMed ID: 25654782]. https://doi.org/10.1097/RCT.0000000000000216.
-
11.
Schaverien M, Saint-Cyr M, Arbique G, Hatef D, Brown SA, Rohrich RJ. Three- and four-dimensional computed tomographic angiography and venography of the anterolateral thigh perforator flap. Plast Reconstr Surg. 2008;121(5):1685-96. [PubMed ID: 18453993]. https://doi.org/10.1097/PRS.0b013e31816b4587.
-
12.
Sharara SM, Monnin SR, Rubio M, Khouzam RN, Ragheb SR. Can Radiation Dose Burden of CT Angiography be Reduced While Still Accurately Diagnosing Etiology of Acute Chest Pain? Curr Probl Cardiol. 2021;46(4):100766. [PubMed ID: 33385751]. https://doi.org/10.1016/j.cpcardiol.2020.100766.
-
13.
Li X, Yang K, Liu B. Characterization of radiation dose from tube current modulated CT examinations with considerations of both patient size and variable tube current. Med Phys. 2017;44(10):5413-22. [PubMed ID: 28681439]. https://doi.org/10.1002/mp.12460.
-
14.
Yanaga Y, Awai K, Nakaura T, Utsunomiya D, Funama Y, Date S, et al. Hepatocellular carcinoma in patients weighing 70 kg or less: initial trial of compact-bolus dynamic CT with low-dose contrast material at 80 kVp. AJR Am J Roentgenol. 2011;196(6):1324-31. [PubMed ID: 21606296]. https://doi.org/10.2214/AJR.10.4545.
-
15.
Cho YJ, Schoepf UJ, Silverman JR, Krazinski AW, Canstein C, Deak Z, et al. Iterative image reconstruction techniques: cardiothoracic computed tomography applications. J Thorac Imaging. 2014;29(4):198-208. [PubMed ID: 24662334]. https://doi.org/10.1097/RTI.0000000000000041.
-
16.
Takahashi M, Kimura F, Umezawa T, Watanabe Y, Ogawa H. Comparison of adaptive statistical iterative and filtered back projection reconstruction techniques in quantifying coronary calcium. J Cardiovasc Comput Tomogr. 2016;10(1):61-8. [PubMed ID: 26276567]. https://doi.org/10.1016/j.jcct.2015.07.012.