Abstract
Background:
Coronavirus disease 2019 (COVID-19) has emerged as the most significant health crisis in recent years, leading to over 6 million deaths globally due to the disease.Objectives:
Given the prevalence of multisystem inflammatory syndrome in children (MISC) following the COVID-19 pandemic, this study aims to examine the imaging findings and prognoses of clinical and subclinical myocarditis in children with MISC through cardiac magnetic resonance (CMR) imaging.Patients and Methods:
This prospective cohort study carried out over eighteen months from May 2021 to November 2022, included 14 patients who underwent CMR imaging. A census of all eligible patients during the study period served as the sampling method. Inclusion criteria were patients with confirmed COVID-19 infection through serological tests, polymerase chain reaction (PCR), or recent exposure to COVID-19 patients. Exclusion criteria included patients with a history of congenital heart disease (CHD) or pulmonary disease. Additional diagnostic tests performed included blood sample tests, chest X-ray (CXR), electrocardiogram (ECG), and echocardiography. CMR imaging was conducted on patients with cardiac involvement. A diagnosis of myocardial inflammation was made if a patient met at least two of the Lake Louise Criteria. The Chi-square, Fisher's exact, and Mann-Whitney tests were used to examine the relationship between quantitative variables and treatment outcomes. Additionally, the Wilcoxon signed rank, and McNemar’s tests assessed changes in echocardiography findings from admission to follow-up. A significance level of 0.05 was set.Results:
Among the 14 patients studied, 8 (57.10%) were girls and 6 (42.90%) were boys. The average age was 6.03 ± 3.71 years. The median time to CMR imaging after symptom onset was 4 weeks (interquartile range (IQR): 2 - 12, range: 30). Global function assessment using left ventricular ejection fraction (LVEF) showed that 5 (35.70%), 3 (21.40%), and 6 (42.90%) patients had normal function, mild dysfunction, and significant LV dysfunction, respectively. 71.40% of patients who recovered had mild tricuspid regurgitation (TR) and no cardiomegaly. Significant differences in mean values of polymorphonuclear neutrophil (PMN) (37.71 ± 11.75 vs. 81.44 ± 13.06), lymphocytes (48.71 ± 20.08 vs. 12.51 ± 7.26), hemoglobin (Hb) (12.60 ± 1.55 vs. 10.10 ± 1.62), mean corpuscular volume (MCV) (85.90 ± 5.67 vs. 79.37 ± 5.23), erythrocyte sedimentation rate (ESR) (8.86 ± 13.60 vs. 30.29 ± 21.33), and C-reactive protein (CRP) (18.91 ± 27.25 vs. 100.57 ± 85.67) were observed between non-recovered and recovered patients, respectively (P < 0.05). However, no statistically significant association was found between other variables, including N-terminal pro–B-type natriuretic peptide (NT-proBNP), D-dimer, and Troponin I (TPI), with treatment outcomes (P > 0.05).Conclusion:
Our findings indicate that a negative COVID-19 test does not exclude an established clinical COVID-19 infection in children with MISC. The results suggest that all children with MISC and a history of COVID-19 infection should undergo assessment for myocardial fibrosis, regardless of ejection fraction (EF) as determined by Echocardiography, laboratory tests, and COVID-19 test results. Strain analysis, conducted during both the acute phase and subsequent follow-ups through CMR imaging or Echocardiography, is recommended to enhance the understanding of the prognosis.Keywords
1. Background
The coronavirus disease 2019 (COVID-19), first identified in late 2019, has swiftly become a global pandemic affecting people of all ages. It has become a major health concern in recent years, leading to over 6 million deaths worldwide. Beyond the high mortality rate, COVID-19 has also caused long-term health complications for survivors and placed immense strain on healthcare systems around the world. While individuals of all ages are susceptible to severe illness and death, those over 60 years old and those with pre-existing medical conditions are at a significantly higher risk. Notably, severe cases among children are less common (1), with over 90% of pediatric cases exhibiting asymptomatic, mild, or moderate symptoms (2). However, a small percentage of children develop severe symptoms marked by hyper-inflammation, leading to multi-organ involvement similar to macrophage activation syndrome (MAS), toxic shock syndrome (TSS), and Kawasaki disease (KD) (3). This condition, known as multisystem inflammatory syndrome in children (MISC) (4), is a rare but serious complication that typically arises 2 - 6 weeks following infection with COVID-19 (4). The diagnostic criteria for MISC include a fever lasting more than 24 hours, involvement of two or more organ systems (cardiac, renal, respiratory, hematologic, gastrointestinal, dermatologic, or neurological), and the absence of any plausible alternative diagnoses (4). Cardiac involvement in MISC is a common and serious concern, leading to fatalities even after recovery from COVID-19, underscoring the need for further research to enhance our understanding and management of this condition. Cardiovascular complications, including myocarditis, have been linked to increased mortality due to permanent damage to the heart muscle, development of arrhythmias, sudden cardiac death (SCD), or chronic heart failure (5). Despite these observations, our knowledge of the cardiac implications of MISC remains incomplete, with a significant gap in understanding the long-term cardiac effects and the prognosis for children with MISC experiencing clinical and subclinical myocarditis. Cardiovascular magnetic resonance (CMR) imaging has detected abnormal findings in adults, even those asymptomatic for COVID-19 (6). Importantly, a significant number of children who have recovered from MISC show abnormal CMR imaging results, such as myocardial edema and late gadolinium enhancement (LGE), with varying severity levels (7).
2. Objectives
This study aimed to examine the imaging findings and prognosis of clinical and subclinical myocarditis in children with MISC using cardiac MRI imaging.
3. Patients and Methods
This study was a prospective cohort investigation conducted at Imam Reza, Sheikh, and Akbar Hospitals in Mashhad, Iran.
3.1. Selection Criteria
It encompassed all hospitalized pediatric patients aged 21 years or younger diagnosed with MISC between May 2021 and May 2022. The inclusion of patients followed the case definitions set forth by the New York State Department of Health (NYSDOH) and the Centers for Disease Control and Prevention (CDC) for MISC.
Patients were included if they had a confirmed infection of COVID-19, verified by serological tests or polymerase chain reaction (PCR). Furthermore, inclusion criteria extended to patients who recently had contact with individuals diagnosed with COVID-19. The specific criteria from the NYSDOH and CDC for diagnosing MISC were employed, encompassing various clinical signs and symptoms such as fever, laboratory evidence of inflammation, and severe illness necessitating hospitalization. A team of clinicians reviewed and confirmed each patient's eligibility, ensuring a precise application of these criteria to minimize ambiguity in patient selection and reduce the potential for bias.
Patients with a history of congenital heart disease (CHD) or pulmonary disease were excluded from the study. The rationale for excluding patients with CHD was that this pre-existing condition could potentially confound the results of the study. CHD could influence the cardiac parameters under investigation, making it challenging to ascertain if observed abnormalities were solely attributable to MISC. Similarly, patients with a history of pulmonary disease were excluded to avoid confounding effects on respiratory symptoms and inflammatory markers associated with MISC. These exclusions aimed to ensure that the study outcomes accurately reflected the impact of MISC on the patient population without being influenced by these pre-existing conditions. A thorough review of each patient's medical history was conducted to adhere to these criteria.
3.2. Sample Size Justification
The sampling method involved conducting a census of all eligible patients during the study period. The sample size was determined by the number of patients who met the inclusion criteria and underwent cardiac magnetic resonance (CMR) imaging at Imam Reza, Sheikh, and Akbar Hospitals in Mashhad, Iran, during the specified timeframe. As part of our prospective cohort study, we aimed to include all eligible cases within this period. We recognize that the sample size may limit the study's statistical power and its applicability to a broader population. Nonetheless, considering the rarity of MISC, our study offers valuable insights into this condition within the studied population. Future studies, ideally multicentric and with larger sample sizes, are necessary to corroborate and build upon our findings.
3.3. Clinical and Laboratory Evaluation
The primary outcome of this study focused on the relationship between various clinical and laboratory variables (including N-terminal pro–B-type natriuretic peptide [NT-proBNP], D-dimer, troponin I, polymorphonuclear neutrophil [PMN], lymph, hemoglobin [Hb], mean corpuscular volume [MCV], erythrocyte sedimentation rate [ESR], C-reactive protein [CRP], tricuspid regurgitation, aortic regurgitation [AI], pericardial effusion, pleural effusion, mitral regurgitation [MR], and electrocardiogram [ECG] results) and the outcome of treatment. The secondary outcomes examined the differences in these variables between patients who recovered and those who did not. These outcomes were selected to provide a thorough understanding of MISC's effects on patients’ health. Blood samples were collected from patients upon admission and analyzed in the hospital’s central laboratory, including Complete Blood Count with Differential (CBC Diff), ESR, CRP, lactate dehydrogenase (LDH), D dimer, NTproBNP, cardiac troponin, creatine kinase-myoglobin binding (CK-MB), alanine aminotransferase (ALT), aspartate transaminase (AST), blood urea nitrogen (BUN), creatinine (Cr), ferritin, fibrinogen, and procalcitonin, using the hospital’s standard protocols. The findings are detailed in Tables 1 and 2.
Comparison of Demographic and Clinical Characteristics between Patients with and Without Recovery a, b
Variables | Recovery | Test Results | |
---|---|---|---|
No | Yes | ||
Gender | χ2 = 0.00; P = 0.99 | ||
Female | 4 (57.10) | 4 (57.10) | |
Male | 3 (42.90) | 3 (42.90) | |
Exposure | χ2 = 1.07; P = 0.29 | ||
No | 0 (0.00) | 1 (14.30) | |
Yes | 7 (100.00) | 6 (85.70) | |
Treatment type | χ2 = 6.53; P = 0.11 | ||
Heart failure treatment+ inotropic agents +IVIg + pulse methylprednisolone | 2 (28.60) | 3 (42.90) | |
Heart failure treatment + inotropic agents + IVIg + pulse methylprednisolone | 0 (0.00) | 2 (28.60) | |
IVIg + pulse methylprednisolone | 1 (14.30) | 2 (28.60) | |
Heart failure treatment | 4 (57.10) | 0 (0.00) | |
Chest X-ray | χ2 = 7.77; P = 0.02 c | ||
No cardiomegaly | 0 (0.00) | 5 (71.40) | |
Cardiomegaly | 7 (100.00) | 2 (28.60) | |
Age, y | 6.49 ± 4.33 | 5.57 ± 3.25 | Z = - 0.38; P = 0.70 |
Weight, kg | 21.00 ± 10.01 | 25.00 ± 12.07 | Z = - 0.70; P = 0.48 |
Body surface area (BSA) | 0.84 ± 0.30 | 0.92 ± 0.29 | Z = - 0.70; P = 0.48 |
Pulse rate | 117.86 ± 22.70 | 138.57 ± 31.71 | Z = - 1.28; P = 0.19 |
Respiratory rate | 30.00 ± 8.02 | 32.86 ± 10.52 | Z = - 0.51; P = 0.60 |
Systolic blood pressure (SBP) | 88.57 ± 6.26 | 90.29 ± 11.57 | Z = - 0.78; P = 0.43 |
Diastolic blood pressure (DBP) | 62.14 ± 6.36 | 57.57 ± 9.37 | Z = - 1.11; P = 0.26 |
Variables | Recovery | Test Results | |
---|---|---|---|
No | Yes | ||
NTproBNP | χ2 = 2.77; P = 0.28 | ||
< 1000 | 0 (0.00) | 1 (14.30) | |
1000 - 5000 | 2 (28.60) | 4 (57.10) | |
> 5000 | 5 (71.40) | 2 (28.60) | |
D-dimer | χ2 = 1.50; P = 0.63 | ||
< 1000 | 3 (42.90) | 1 (14.30) | |
1000 - 2000 | 1 (14.30) | 2 (28.60) | |
> 2000 | 3 (42.90) | 4 (57.10) | |
TPI | χ2 = 2.80; P = 0.26 | ||
Negative | 3 (42.90) | 6 (85.70) | |
Positive | 4 (57.10) | 1 (14.30) | |
SPO2 | 96.00 ± 3.16 | 96.43 ± 2.57 | Z = - 0.06; P = 0.94 |
WBC | 10.67 ± 6.65 | 12.18 ± 7.31 | Z = - 0.83; P = 0.40 |
PMN | 37.71 ± 11.75 | 81.44 ± 13.06 | Z = - 3.00; P = 0.003 b |
Lymph | 48.71 ± 20.08 | 12.51 ± 7.26 | Z = - 2.74; P = 0.006 b |
Hb | 12.60 ± 1.55 | 10.10 ± 1.62 | Z = - 2.36; P = 0.01 b |
MCV | 85.90 ± 5.67 | 79.37 ± 5.23 | Z = - 2.37; P = 0.01 b |
Plt | 226428.57 ± 62406.88 | 185857.14 ± 96340.41 | Z = - 0.19; P = 0.56 |
ESR | 8.86 ± 13.60 | 30.29 ± 21.33 | Z = - 2.49; P = 0.01 b |
CRP | 18.91 ± 27.25 | 100.57 ± 85.67 | Z = - 2.23; P = 0.02 b |
PT | 15.05 ± 3.99 | 13.85 ± 0.89 | Z = - 0.19; P = 0.84 |
PTT | 27.86 ± 2.41 | 32.86 ± 7.17 | Z = - 1.28; P = 0.19 |
INR | 1.31 ± 0.46 | 1.11 ± 0.12 | Z = - 0.98; P = 0.32 |
AST | 593.57 ± 1392.35 | 79.14 ± 51.79 | Z = - 0.12; P = 0.89 |
ALT | 910.29 ± 2240.41 | 63.57 ± 36.74 | Z = - 0.12; P = 0.89 |
ALP | 441.43 ± 160.22 | 435.14 ± 71.92 | Z = - 0.97; P = 0.33 |
BUN | 37.43 ± 18.66 | 36.57 ± 15.36 | Z = 0.00; P = 0.99 |
Cr | 0.69 ± 0.22 | 0.59 ± 0.08 | Z = - 0.78; P = 0.43 |
LDH | 983.71 ± 1163.67 | 600.71 ± 150.05 | Z = - 0.25; P = 0.79 |
Alb | 3.48 ± 0.80 | 2.94 ± 0.47 | Z = - 1.66; P = 0.09 |
D-dimer | 1848.00 ± 1714.84 | 2619.43 ± 1562.89 | Z = - 0.83; P = 0.40 |
Additionally, on the first day of admission, each patient underwent a standard posterior-anterior view chest X-ray, ECG, and echocardiography. Based on the clinical and paraclinical observations, patients showing signs of cardiac involvement were subject to CMR imaging. Myocardial inflammation was diagnosed in patients meeting at least two of the Lake Louise criteria.
3.4. Follow-Up
All patients in the study were followed up for a period of six months. During this follow-up period, each patient underwent another echocardiography. The results of these follow-up echocardiograms are displayed in Tables 3 and 4. This follow-up process enabled us to monitor the patients' progress over time and to document any relevant outcomes or events.
Comparison of Echocardiography Findings on Admission and in Follow-up Between Patients with and Without Recovery a
Variables | Echocardiography Findings on Admission | Echocardiography Findings in Follow-up | ||||
---|---|---|---|---|---|---|
Recovery | Test Results | Recovery | Test Results | |||
No | Yes | No | Yes | |||
Aortic regurgitation | χ2 = 3.81; P = 0.19 | - | - | - | ||
No or trivial | 7 (100.00) | 4 (57.10) | ||||
Mild | 0 (0.00) | 3 (42.90) | ||||
Pericardia effusion | χ2 = 0.31; P = 0.99 | - | - | - | ||
No | 4 (57.10) | 5 (71.40) | ||||
Mild | 3 (42.90) | 2 (28.60) | ||||
Pleural effusion | χ2 = 0.66; P = 0.99 | - | - | - | ||
No | 3 (42.90) | 4 (57.10) | ||||
Mild | 3 (42.90) | 2 (28.60) | ||||
Severe | 1 (14.30) | 1 (14.30) | ||||
Electrocardiogram | χ2 = 0.28; P = 0.99 | - | - | - | ||
Normal | 3 (42.90) | 4 (57.10) | ||||
Abnormal | 4 (57.10) | 3 (42.90) | ||||
TR | χ2 = 7.66; P = 0.02 b | χ2 = 4.56; P = 0.13 | ||||
No or trivial | 1 (14.30) | 0 (0.00) | 0 (0.00) | 2 (28.60) | ||
Mild | 0 (0.00) | 5 (71.40) | 1 (33.30) | 5 (71.40) | ||
Moderate | 6 (85.70) | 2 (28.60) | 2 (66.70) | 0 (0.00) | ||
MR | χ2 = 1.72; P = 0.56 | χ2 = 4.70; P = 0.07 | ||||
No or trivial | 0 (0.00) | 1 (14.30) | 0 (0.00) | 3 (42.90) | ||
Mild | 1 (14.30) | 2 (28.60) | 1 (33.30) | 4 (57.10) | ||
Moderate | 6 (85.70) | 4 (57.10) | 2 (66.70) | 0 (0.00) | ||
EF (M-mode), % | 21.71 ± 14.62 | 56.14 ± 14.17 | Z = - 2.82; P = 0.005 b | 33.33 ± 17.00 | 64.42 ± 2.63 | Z = - 2.42; P = 0.01 b |
TAPSE | 1.10 ± 0.24 | 1.93 ± 0.36 | Z = - 2.82; P = 0.005 b | 1.36 ± 0.32 | 2.03 ± 0.13 | Z = - 2.43; P = 0.01 b |
Ao_VTI | 10.57 ± 2.24 | 19.00 ± 5.41 | Z = - 2.43; P = 0.01 b | 15.16 ± 6.60 | 20.92 ± 2.09 | Z = - 1.38; P = 0.16 |
E_A | 2.06 ± 0.86 | 1.73 ± 0.46 | Z = - 0.51; P = 0.60 | 1.50 ± 0.56 | 1.81 ± 0.45 | Z = - 0.58; P = 0.55 |
E_Em | 13.10 ± 8.66 | 10.94 ± 5.96 | Z = - 1.08; P = 0.27 | 9.66 ± 1.52 | 9.18 ± 1.60 | Z = - 0.23; P = 0.81 |
LVEDd_cm | 5.37 ± 1.01 | 4.59 ± 0.36 | Z = - 1.15; P = 0.24 | - | - | - |
EF_sympson | 13.86 ± 14.17 | 50.14 ± 9.33 | Z= - 3.01; P = 0.003 b | - | - | - |
Em_cm_s | 5.80 ± 2.35 | 9.50 ± 2.81 | Z = - 2.23; P = 0.02 b | - | - | - |
E_cm_s | 80.92 ± 19.36 | 92.00 ± 12.76 | Z = - 1.28; P = 0.20 | - | - | - |
Echocardiography Findings and Admission | Follow-up | Test Results | ||
---|---|---|---|---|
No or Trivial | Mild | Moderate | ||
TR | χ2 = 3.00; P = 0.39 | |||
No or trivial | 0 (0.00) | 0 (0.00) | 1 (10.00) | |
Mild | 1 (10.00) | 4 (40.00) | 0 (0.00) | |
Moderate | 1 (10.00) | 2 (20.00) | 1 (10.00) | |
MR | χ2 = 6.00; P = 0.11 | |||
No or trivial | 0 (0.00) | 1 (10.00) | 0 (0.00) | |
Mild | 0 (0.00) | 2 (20.00) | 0 (0.00) | |
Moderate | 3 (30.00) | 2 (20.00) | 2 (20.00) |
3.5. Echocardiography
Echocardiography was performed in accordance with ASE guidelines using Philips Affinity 70 and Philips Epic echocardiography systems. The coronary artery dimension was evaluated using the Boston Z score. Measurements, such as LVEF (M-Mode), LVEF (Simpson), left ventricular end-diastolic diameter (LVEDd), tissue Doppler imaging of mitral valve inflow (TDI MV) (Em/Am), mitral E-wave velocity (MV E), Mitral Em-wave velocity (MV Em), MV(E/A), MV(E/Em), aortic velocity time integral (AO VTI), tricuspid annular plane systolic excursion (TAPSE), TR pressure gradient (PPG), and Pulmonic Valve Regurgitation PPG, were taken. All measurements were reviewed by subspecialty experts in echocardiography.
3.6. Cardiac Magnetic Resonance
The cardiac MRI exams were conducted with a 1.5 T scanner from GE Healthcare using a 16-phased-array cardiac coil and retrospective vector cardiography gating. Images were captured during breath-holds at end-expiration with the patient in a supine position. For patients unable to hold their breath, CMR imaging was performed under light general anesthesia (chloral hydrate) administered by an anesthesiologist, allowing for free breathing and ECG triggering.
Vertical long-axis cine images were obtained to better visualize the left ventricle by aligning the apex of the left ventricle with the center of the mitral valve. From these images, the horizontal long-axis plane was derived by aligning the midpoint of the apex and valve. These images served as the reference for a stack of contiguous short-axis slices, starting from the atrioventricular groove and ending at the apex. Depending on the length of the left ventricle's long axis, 8-12 contiguous slices were taken to encompass the entire left ventricle. A segmented, balanced steady-state free precession imaging sequence (FIESTA) with specific parameters (time of repetition [TR] / echo time [TE] / flip angle [FA]; 1.6/1.3/65; slice thickness: 8 mm; spacing: 8; field of view [FOV]: 380; voxel size: 1.7 × 2; BW: 67 KHz/Px, temporal resolution: 20) was utilized to acquire these images.
TT1 double inversion recovery fast spin echo (T1 DIR FSE) images were captured from the same locations as those in the Short Axis (SAX) image stack. Their parameters were as follows: TE/FA at 750/min/90, with a slice thickness of 8 mm, FOV of 380, voxel size of 2 × 1.8, ETL of 24, and BSP-TE of 379. To evaluate myocardial edema, T2 DIR FSE images in the SAX view were obtained with the parameters TR/TE/FA at 900/102/140, slice thickness at 8 mm, FOV of 450, voxel size of 1.9 × 1.8, and BW of 62 KHz/Px.
Myocardial tagging images were acquired from basal to apical segments in the SAX view using complementary spatial modulation of magnetization (CSPAMM). Phase contrast imaging for flow assessment was performed at the levels of the ascending and descending aorta, superior vena cava, main pulmonary artery, mitral valve, and tricuspid valve. Myocardial delayed enhancement (MDE) images in SAX, four-chamber, and two-chamber views were captured 10 to 20 minutes after administering an intravenous bolus dose of 0.1 mmol/kg Gadoterate meglumine (Dotarem®, Guerbet, France). The scanning parameters were set as TR/ TE/FA at 780/min/25, with a slice thickness of 8 mm, FOV of 400, voxel size of 2.1 × 1.6 × 8, BW of 25 - 31 KHz/Px, and Number of excitation (NEX) at 2. The inversion time was adjusted between 400 and 450 ms to nullify the signal from the viable myocardium.
3.7. CMR Imaging Post-processing
An experienced level II reader, utilizing commercially available software (CVi42, Circle Cardiovascular Imaging, Calgary), performed all offline analyses. For regional analyses, the left ventricle (LV) was segmented according to a 16-segment model (the AHA/ACC 17-segment model minus the apical cap segment). To assess the function and mass of the left ventricle, the end-diastolic and end-systolic cine frames for each slice were identified, and the endocardial and epicardial borders were manually traced. The volumes for both the end-diastolic and end-systolic phases were calculated using the summation of discs method.
To assess the volume and function of the left ventricle, we manually outlined the borders of the short-axis cine images during end-diastole (when the left ventricular cavity is at its largest) and end-systole (when the left ventricular cavity is at its smallest). This process enabled us to determine the left ventricular end-diastolic volume and left ventricular end-systolic volume. These measurements were then used to calculate the left ventricular stroke volume (the difference between the end-diastolic and end-systolic volumes) and the left ventricular ejection fraction (LVEF), which is the stroke volume divided by the end-diastolic volume, then multiplied by 100%. The papillary muscles were included in the calculation of the left ventricle mass but were excluded from the volume calculations of the left ventricle. We assessed the thickness of the heart walls using a modified centerline method. Wall thickening was calculated by subtracting the end-diastolic wall thickness from the end-systolic wall thickness, dividing this by the end-diastolic wall thickness, and then multiplying by 100. Plots of radial wall thickness and thickening will be generated to observe changes in regional wall geometry based on the randomization strategy. LGE images were examined to identify myocardial scarring (infarcted tissue). The endocardial and epicardial borders were manually traced on each slice, and the myocardium showed signal intensity of more than two standard deviations above the mean signal intensity of the normal myocardium, which was identified as a hyperenhanced area. Myocardial delayed enhancement (MDE) was classified as subendocardial, mid-wall, or transmural. A comprehensive analysis of global, segmental, and multi-segmental myocardial strain in circumferential, radial, and longitudinal directions was conducted.
3.8. Ethics
This study was approved by the Ethics Committee of Mashhad University of Medical Sciences under the code IR.MUMS.REC.1401.285.
3.9. Statistical Analysis
The study reported quantitative data as means with standard deviations (SDs), and qualitative data were presented as frequency percentages. The Chi-square and Fisher's exact tests were used to compare the frequency distribution of qualitative variables between the two treatment outcomes (recovered and non-recovered). Additionally, the comparison of quantitative variables at admission and during follow-up was conducted using the non-parametric Wilcoxon signed-rank test. The non-parametric Mann-Whitney test was utilized to compare the means of quantitative variables in relation to treatment outcome (recovered and non-recovered). McNemar's test was applied to examine changes in qualitative echocardiography findings (MR and TR) from admission to follow-up. All analyses were carried out using IBM SPSS Statistics for Windows, Version 20.0, Armonk, NY: IBM Corp. The level of significance was set at 0.05.
4. Results
4.1. Patients’ Demographics
In this study, 14 patients who met the inclusion criteria were included. The mean age of the patients under study was 6.03 ± 3.71 years, with 8 (57.10%) cases being girls, having a mean age of 5.69 ± 3.86 years, and 6 (42.90%) cases being boys, with a mean age of 6.48 ± 3.81 years. There was no significant difference in the mean ages of boys and girls (P > 0.05).
4.2. Treatment Outcomes
Among the patients who recovered, 4 (57.10%) were girls and 3 (42.90%) were boys. There was no statistically significant association between gender and treatment outcome (P > 0.05). Similarly, there was no significant association between variables such as exposure, type of treatment, and chest X-ray (CXR) results with the treatment outcome (recovered and non-recovered) (P > 0.05). The mean ages of patients with and without recovery were 5.57 ± 3.25 years and 6.49 ± 4.33 years, respectively, showing no significant difference in age between the treatment outcomes (P > 0.05). Moreover, no significant differences were found between the recovered and non-recovered groups in terms of mean weight, body surface area (BSA), and vital signs (P > 0.05), as shown in Table 1.
4.3. Laboratory Findings
The laboratory findings related to treatment outcomes are detailed in Table 2. Among the 7 patients who recovered, a majority (57.10%) had NTproBNP levels between 1000 and 5000 and D-dimer levels higher than 2000. Furthermore, 6 (85.70%) of the recovered patients had negative TPI results. Fisher's exact test indicated that there was no statistically significant association between NTproBNP, D-dimer, and TPI levels and treatment outcomes (P > 0.05). According to the Mann-Whitney test, there was a statistically significant difference in the means of PMN, lymph, Hb, MCV, ESR, and CRP between recovered and non-recovered patients (P < 0.05). However, no significant differences were found in the mean values of oxygen saturation (SPO2), white blood cell (WBC), platelets (Plt), prothrombin time (PT), partial thromboplastin Time (PTT), international normalized ratio (INR), AST, ALT, alkaline phosphatase (ALP), BUN, Cr, LDH, albumin (Alb), and D-dimer between the recovered and non-recovered groups (P > 0.05).
4.4. Echocardiography Findings on Admission
As shown in Table 3, at the time of admission, 71.40% of patients with mild TR recovered, demonstrating a statistically significant association between TR and treatment outcome (P < 0.05). However, no statistically significant association was found between AI, pericardial effusion, pleural effusion, MR, and ECG findings and treatment outcome (P > 0.05). Additionally, a statistically significant difference was noted in the mean values of EF (M-mode), TAPSE, Ao_VTI, EF_sym, and Em_cm_s between the recovered and non-recovered patients (P < 0.05). Nevertheless, no statistically significant difference was observed in the means of other echocardiographic findings between the recovered and non-recovered groups (P > 0.05).
4.5. Echocardiography Findings at Follow-up
Table 3 reveals that, at the time of follow-up, most of the patients who recovered exhibited mild TR and MR. However, no statistically significant association was found between these variables and treatment outcome (P > 0.05). Additionally, a statistically significant difference was observed between the mean values of EF (M-mode) and TAPSE among the recovered and non-recovered groups (P < 0.05). Yet, no significant difference was noted between the two groups in terms of the mean values for Ao_VTI, E_A, and E_Em (P > 0.05).
There was no statistically significant change in echocardiography findings concerning TR and MR from admission to follow-up time (P > 0.05), as presented in Table 4.
Figure 1 indicates no significant difference in the mean values of EF (49.50 ± 16.66 at admission vs. 55.10 ± 17.16 at follow-up), TAPSE (1.72 ± 0.47 at admission vs. 1.83 ± 0.37 at follow-up), Ao_VTI (16.60 ± 6.11 at admission vs. 19.20 ± 4.51 at follow-up), E_A (1.74 ± 0.45 at admission vs. 1.74 ± 0.46 at follow-up), and E_Em (11.07 ± 4.94 at admission vs. 9.33 ± 1.51 at follow-up) between the admission and follow-up times (P > 0.05).
Comparing the quantitative echocardiography findings at the time of admission with the follow-up time. AO_VTI, aortic velocity time integral; E_A, mitral EA-wave velocity; E_EM, mitral E-Em-wave velocity; TAPSE, tricuspid annular plane systolic excursion; EF, ejection fraction.
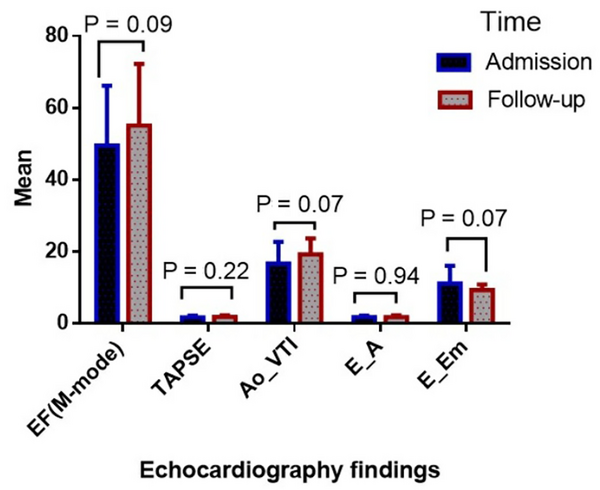
4.6. Cardiac Magnetic Resonance Imaging Findings
The results from CMR imaging conducted on all patients diagnosed with myocarditis are documented in Table 5. The median time to MRI was 4 weeks (IQR: 2 - 12 weeks, Range: 30 weeks) following the onset of illness symptoms. T2 DIR FSE images were used to assess myocardial edema in all patients, with none showing signs of myocardial edema. Consequently, since myocardial edema was not observed in any patient, early gadolinium enhancement (EGE) was not assessed.
Cardiac Magnetic Resonance Imaging Results on the Volume, Function, and Late Gadolinium Enhancement Pattern
ID | Indexed LV End-Diastolic Volume (ILVEDV, mL/m2) | Indexed LV End-Systolic Volume (ILVESV, mL/m2) | Indexed LV Systolic Volume ILVSV, mL/m2) | LVEF (%) | Peak Longitudinal strain (%) | Peak Circumferential strain (%) | Peak Radial strain (%) | LGE | Pericardial Effusion | Time to CMR Imaging |
---|---|---|---|---|---|---|---|---|---|---|
1 | 163.00 | 150.00 | 13.00 | 8.00 | 3.00 | 4.00 | 3.00 | LGE | No | 3 mo |
2 | 45.80 | 42.50 | 32.80 | 7.10 | 3.00 | 4.00 | 3.00 | LGE | No | 3 mo |
3 | 211.00 | 186.00 | 25.40 | 12.00 | 1.00 | 3.00 | 4.00 | LGE | No | 2 mo |
4 | 62.70 | 25.90 | 36.70 | 58.60 | 15.00 | 17.00 | 27.00 | No LGE | No | 8 mo |
5 | 65.00 | 23.60 | 36.30 | 64.00 | 15.00 | 17.00 | 27.00 | No LGE | No | 2 w |
6 | 44.60 | 15.50 | 29.00 | 65.00 | 14.00 | 16.00 | 20.00 | No LGE | No | 2 w |
7 | 83.00 | 39.00 | 44.00 | 53.00 | 1.00 | 3.00 | 4.00 | LGE | No | 1 mo |
8 | 61.60 | 21.10 | 40.50 | 65.70 | 14.50 | 18.00 | 14.50 | LGE | No | 2 w |
9 | 68.00 | 32.00 | 45.90 | 52.00 | 11.00 | 16.00 | 14.00 | No LGE | Yes | 2 w |
10 | 68.10 | 14.90 | 53.20 | 78.00 | 14.00 | 18.00 | 26.00 | LGE | Yes | 2 w |
11 | 99.00 | 77.00 | 22.00 | 22.00 | 1.00 | 4.00 | 3.00 | LGE | No | 1 mo |
12 | 158.00 | 115.00 | 42.60 | 27.00 | 1.00 | 3.00 | 4.00 | LGE | No | 4 mo |
13 | 225.10 | 218.00 | 6.60 | 3.00 | 1.00 | 3.00 | 4.00 | LGE | Yes | 2 mo |
14 | 59.90 | 22.60 | 32.20 | 53.00 | 11.00 | 14.00 | 16.00 | LGE | No | 2 w |
Mean ± SD | 101.05 ± 61.59 | 70.22 ± 68.91 | 32.87 ± 12.89 | 40.60 ± 26.13 | 7.53 ± 6.33 | 10.00 ± 6.89 | 12.10 ± 9.74 | - | - |
4.7. Global Function Measurement Using LVEF
The assessment of global function via LVEF showed that five patients had normal function (EF > 55%), three patients exhibited mild dysfunction (EF 50 - 55%), and six patients demonstrated significant left ventricular (LV) dysfunction (EF < 50%). LGE and pericardial effusion were present in 10 (71.43%) and 3 (21.43%) patients, respectively, with patterns of LGE frequently observed in the basal and mid-septal segments.
4.8. Specific Case Findings
Cardiac magnetic resonance imaging revealed severe and extensive subendocardial to transmural fibrosis across all segments from the base to the apex of the left ventricle and in the basal right ventricular free wall in patient 11. This patient exhibited significant biventricular dilatation, severe systolic dysfunction, significant biatrial enlargement, hyper-trabeculation of the LV and RV myocardium, and severe global hypokinesia to akinesia, leading to a diagnosis of extensive transmural myocardial infarction.
4.9. Patients Outcomes
Among the 14 patients studied, unfortunately, patients 2 and 3 passed away. Patients 12 and 13 received heart transplants, and despite treatment for heart failure, patients 1, 7, and 11 showed no improvement after 6 months of follow-up. The remaining patients demonstrated an acceptable EF during their follow-up echocardiography.
5. Discussion
Only a few months after the COVID-19 pandemic began, a novel disease with clinical features similar to Kawasaki disease was reported (8). MISC was first identified in April 2020 (9). While most cases of pediatric COVID-19 are mild or asymptomatic, there have been instances of severe disease and fatalities, often linked to MISC (10). The severity in patients was attributed to the development of MISC (11), which can present with a variety of gastrointestinal, dermatological, cardiovascular, respiratory, neurological, and renal manifestations. However, cardiovascular involvement remains the most concerning aspect of MISC (11, 12).
Our study determined that a negative COVID test does not negate an established clinical COVID-19 infection. Every child with MISC and a history of COVID-19 infection should undergo assessment for myocardial fibrosis, regardless of echocardiography (EF), laboratory examinations, and COVID-19 test outcomes. Strain analysis is essential in all cases, whether during the acute phase or at subsequent follow-ups, using either CMR or echocardiography. It is hypothesized that a delayed hyperimmune response to SARS-CoV-2 exposure may cause cardiac complications in cases that are either mild or test negative for the virus (13).
We evaluated myocardial edema in all patients using T2 DIR FSE images and found no instances of myocardial edema. This absence may be attributable to two factors: Firstly, the initial treatment with corticosteroids as per the treatment protocol for patients diagnosed with myocarditis, and secondly, the passage of at least one month from the onset of COVID-19 infection to the time of CMR imaging. Given the lack of observed myocardial edema, EGE was not assessed. Our CMR imaging findings suggest that COVID-19 can lead to fibrosis across all myocardial layers, including the sub-endocardium, sub-epicardium, transmural, or mid-wall (Figure 2).
A, late gadolinium enhancement (LGE), Short Axis View (SAX) view: The yellow arrows point towards the LGE/fibrosis regions. The yellow regions highlight the areas of LGE and fibrosis. The green and red contours represent the Epicardial and Endocardial contouring, respectively. B, LGE; 4-chamber view: The yellow arrows indicate the LGE/fibrosis regions in a four-chamber view of the heart.
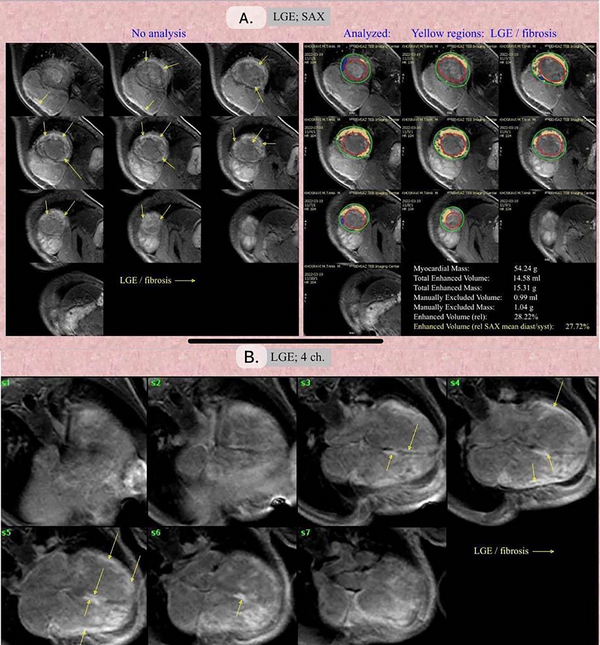
Previous studies by Huang et al. and Puntmann et al. have reported myocardial fibrosis induced by COVID-19 as detected through CMR imaging (14, 15). Both ischemic and non-ischemic cardiomyopathies should be considered potential complications of COVID-19 infection. A normal coronary angiogram cannot exclude the ischemic origin of dilated cardiomyopathy in patients with a history of COVID-19, categorized as myocardial infarction with nonobstructive coronary arteries (MINOCA) or myocardial infarction with normal coronary arteries (MINCA).
A lower TAPSE score, indicative of right ventricular dysfunction, was linked to poorer recovery outcomes. The specific mechanism of cardiac involvement in MISC remains unclear due to limited experimental studies and MISC cases. Nonetheless, several potential mechanisms have been proposed. One theory is that SARS-CoV-2 binds to cells expressing the viral receptor, particularly the angiotensin-converting enzyme 2 (ACE2), leading to the downregulation of myocardial pathways. Another hypothesis posits that the virus may directly invade cells, causing myocarditis (16). Additionally, the primary mechanism is thought to be related to inflammatory cytokines, with studies indicating that IL-10 and TNF-alpha are distinctly elevated markers in MISC compared to COVID-19 (17). TNF-alpha, a cell signaling protein produced by a wide range of cell types, including macrophages and cardiac myocytes, has been implicated in various cardiac effects (18). Janczewski et al. observed that TNF-alpha induced myocyte hypertrophy, alterations in Ca2+ handling, and contractile function, resulting in ventricular dysfunction in an animal model (19). Moreover, arrhythmogenesis has been reported following incubation of rat myocytes with TNF-alpha (20). IL-10, produced in COVID-19 by cells such as monocytes and macrophages, may play a similar role in cardiac dysfunction, with elevated serum levels noted in myocarditis and cardiomyopathies (21). Santoro et al. found that high serum levels of IL-10 were associated with poor outcomes in Takotsubo cardiomyopathy (22).
Furthermore, our study found that higher levels of erythrocyte sedimentation rate (ESR) and CRP were present in patients with more favorable follow-up outcomes. CRP levels are indicative of COVID-19 severity, with higher levels associated with more severe manifestations of the disease (23). Similarly, ESR has been noted to behave similarly in severe COVID-19 cases (24). It has been discussed that inflammatory conditions may exacerbate adverse follow-up events through cardiac involvement. Our findings suggest that not only do elevated ESR and CRP levels correlate with increased inflammation, but lower levels of these markers, while seemingly indicative of less disease severity, are also associated with poorer prognoses.
Cardiac MRI findings indicated that cardiac dysfunction occurs in patients with MISC regardless of the severity of the disease. Our discovery of an association between lower levels of CRP and ESR with poor prognosis suggests that mild or moderate cases may be at a higher risk of mortality due to neglect. Consequently, we recommend that every child diagnosed with MISC undergo an assessment for cardiac dysfunction independent of laboratory examinations and COVID-19 test outcomes.
Aeschlimann et al. found that 18% of their cohort exhibited CMR imaging evidence of myocardial necrosis, pointing to acute myocarditis as a potential manifestation of MISC linked to SARS-CoV-2 infection. This aligns with our observations of myocardial fibrosis in our patients, supporting the notion that a negative COVID-19 test does not exclude an established clinical COVID-19 infection. Additionally, we noted that COVID-19 could lead to fibrosis across all myocardial layers, including the sub-endocardium, sub-epicardium, transmural, and mid-wall. Variations in findings may be due to differences in patient demographics, disease progression, and the timing of CMR imaging, among other factors. Both studies emphasize the potential cardiac effects of MISC associated with COVID-19 and highlight the need for thorough cardiac evaluations in affected patients (25).
Our findings draw parallels with the study by Valverde et al., which also underscored the significant cardiovascular consequences of MISC in children following COVID-19 infection. However, our research offers further insights into the utility of CMR imaging in detecting myocardial fibrosis in children with MISC. We also observed that a majority of children had significantly elevated levels of N-terminal pro-B-type natriuretic peptide, akin to their results. Despite the involvement of multiple systems, very elevated inflammatory markers, and the need for intensive care, mortality in our cases with MISC associated with COVID-19 remained uncommon (26).
Our study underscores the importance of assessing every child with MISC and a history of COVID-19 infection for myocardial fibrosis, regardless of ejection fraction (as determined by echocardiography), laboratory tests, and COVID-19 test results. Additionally, we advocate for conducting strain analysis during both the acute phase and subsequent follow-ups, using either CMR imaging or echocardiography. While Valverde et al. explored a wide array of cardiovascular manifestations, our research focuses more narrowly on myocardial fibrosis and its identification through CMR imaging (26).
Benvenuto et al., in their study involving 20 MISC patients aged between 9 and 17 years, suggest that despite the acute phase's severe cardiac involvement, the cardiac outcomes tend to be positive. However, our findings present a contrast; among the 14 patients we studied, unfortunately, patients 2 and 3 passed away. Patients 12 and 13 required heart transplants, and despite receiving treatment for heart failure, patients 1, 7, and 11 showed no significant improvement after a 6-month follow-up. The remaining patients exhibited an acceptable ejection fraction in their follow-up echocardiography (27).
The insights from these studies may inform future research and clinical practices in managing this complex condition and evaluating the effectiveness of various diagnostic tools, such as CMR imaging.
Our study recognizes several limitations. Firstly, the small sample size limits the generalizability of our findings. The inability to perform CMR imaging on every patient due to reasons such as parental refusal and patient instability, especially in those requiring intensive care, contributed to this limitation. Secondly, the variability in the time interval between the acute phase of MISC and the cardiac MRI scan introduces uncertainty, as the timing of the scan in relation to the disease course could affect the observed manifestations. Thirdly, the lack of a control group weakens the study. A control group comprising either healthy children or children with similar symptoms but not diagnosed with MISC would have provided a baseline for comparison, thereby enhancing the study's strength. Fourthly, the follow-up period was limited, which restricts insights into the long-term cardiac consequences of MISC.
Despite these limitations, we believe our study makes significant contributions to understanding cardiac involvement in MISC.
In conclusion, our findings indicate that a negative COVID-19 test does not exclude an established clinical COVID-19 infection, and every child with MISC and a history of COVID-19 infection should be evaluated for myocardial fibrosis using CMR, regardless of LVEF as determined by echocardiography, laboratory tests, and COVID-19 test results. This recommendation stems from our observations that myocardial fibrosis can occur in cases with a negative COVID-19 test and normal LVEF. Early identification of myocardial fibrosis is crucial for timely intervention and potentially preventing further complications. We also advise that strain analysis be conducted in all cases, during both the acute phase and subsequent follow-ups, using either CMR imaging or echocardiography, as it offers additional insights into myocardial function and aids in monitoring disease progression. Given the small sample size, absence of a control group, and brief follow-up duration, further research with larger samples, extended follow-ups, and comparative designs is necessary to validate our findings and to investigate the long-term outcomes of myocarditis in children with MISC.
References
-
1.
Williams N, Radia T, Harman K, Agrawal P, Cook J, Gupta A. COVID-19 Severe acute respiratory syndrome coronavirus 2 (SARS-CoV-2) infection in children and adolescents: a systematic review of critically unwell children and the association with underlying comorbidities. Eur J Pediatr. 2021;180(3):689-97. [PubMed ID: 32914200]. [PubMed Central ID: PMC7483054]. https://doi.org/10.1007/s00431-020-03801-6.
-
2.
Dong Y, Mo X, Hu Y, Qi X, Jiang F, Jiang Z, et al. Epidemiology of COVID-19 Among Children in China. Pediatrics. 2020;145(6). [PubMed ID: 32179660]. https://doi.org/10.1542/peds.2020-0702.
-
3.
Verdoni L, Mazza A, Gervasoni A, Martelli L, Ruggeri M, Ciuffreda M, et al. An outbreak of severe Kawasaki-like disease at the Italian epicentre of the SARS-CoV-2 epidemic: an observational cohort study. Lancet. 2020;395(10239):1771-8. [PubMed ID: 32410760]. [PubMed Central ID: PMC7220177]. https://doi.org/10.1016/S0140-6736(20)31103-X.
-
4.
Belhadjer Z, Meot M, Bajolle F, Khraiche D, Legendre A, Abakka S, et al. Acute Heart Failure in Multisystem Inflammatory Syndrome in Children in the Context of Global SARS-CoV-2 Pandemic. Circulation. 2020;142(5):429-36. [PubMed ID: 32418446]. https://doi.org/10.1161/CIRCULATIONAHA.120.048360.
-
5.
Friedman KG, Harrild DM, Newburger JW. Cardiac Dysfunction in Multisystem Inflammatory Syndrome in Children: A Call to Action. J Am Coll Cardiol. 2020;76(17):1962-4. [PubMed ID: 33092731]. [PubMed Central ID: PMC7572056]. https://doi.org/10.1016/j.jacc.2020.09.002.
-
6.
Ng MY, Ferreira VM, Leung ST, Yin Lee JC, Ho-Tung Fong A, To Liu RW, et al. Patients Recovered From COVID-19 Show Ongoing Subclinical Myocarditis as Revealed by Cardiac Magnetic Resonance Imaging. JACC Cardiovasc Imaging. 2020;13(11):2476-8. [PubMed ID: 33153536]. [PubMed Central ID: PMC7455163]. https://doi.org/10.1016/j.jcmg.2020.08.012.
-
7.
Blondiaux E, Parisot P, Redheuil A, Tzaroukian L, Levy Y, Sileo C, et al. Cardiac MRI in Children with Multisystem Inflammatory Syndrome Associated with COVID-19. Radiology. 2020;297(3):E283-8. [PubMed ID: 32515676]. [PubMed Central ID: PMC7294821]. https://doi.org/10.1148/radiol.2020202288.
-
8.
Lu X, Zhang L, Du H, Zhang J, Li YY, Qu J, et al. SARS-CoV-2 Infection in Children. N Engl J Med. 2020;382(17):1663-5. [PubMed ID: 32187458]. [PubMed Central ID: PMC7121177]. https://doi.org/10.1056/NEJMc2005073.
-
9.
Riphagen S, Gomez X, Gonzalez-Martinez C, Wilkinson N, Theocharis P. Hyperinflammatory shock in children during COVID-19 pandemic. Lancet. 2020;395(10237):1607-8. [PubMed ID: 32386565]. [PubMed Central ID: PMC7204765]. https://doi.org/10.1016/S0140-6736(20)31094-1.
-
10.
Ludvigsson JF. Systematic review of COVID-19 in children shows milder cases and a better prognosis than adults. Acta Paediatr. 2020;109(6):1088-95. [PubMed ID: 32202343]. [PubMed Central ID: PMC7228328]. https://doi.org/10.1111/apa.15270.
-
11.
Esposito S, Principi N. Multisystem Inflammatory Syndrome in Children Related to SARS-CoV-2. Paediatr Drugs. 2021;23(2):119-29. [PubMed ID: 33479801]. [PubMed Central ID: PMC7819738]. https://doi.org/10.1007/s40272-020-00435-x.
-
12.
Roberts JE, Henderson LA. Clinical features of multisystem inflammatory syndrome in children. Curr Opin Rheumatol. 2021;33(5):378-86. [PubMed ID: 34261116]. [PubMed Central ID: PMC8445592]. https://doi.org/10.1097/BOR.0000000000000818.
-
13.
Ferreira VM, Plein S, Wong TC, Tao Q, Raisi-Estabragh Z, Jain SS, et al. Cardiovascular magnetic resonance for evaluation of cardiac involvement in COVID-19: recommendations by the Society for Cardiovascular Magnetic Resonance. J Cardiovasc Magn Reson. 2023;25(1):21. [PubMed ID: 36973744]. [PubMed Central ID: PMC10041524]. https://doi.org/10.1186/s12968-023-00933-0.
-
14.
Huang L, Zhao P, Tang D, Zhu T, Han R, Zhan C, et al. Cardiac Involvement in Patients Recovered From COVID-2019 Identified Using Magnetic Resonance Imaging. JACC Cardiovasc Imaging. 2020;13(11):2330-9. [PubMed ID: 32763118]. [PubMed Central ID: PMC7214335]. https://doi.org/10.1016/j.jcmg.2020.05.004.
-
15.
Puntmann VO, Carerj ML, Wieters I, Fahim M, Arendt C, Hoffmann J, et al. Outcomes of Cardiovascular Magnetic Resonance Imaging in Patients Recently Recovered From Coronavirus Disease 2019 (COVID-19). JAMA Cardiol. 2020;5(11):1265-73. [PubMed ID: 32730619]. [PubMed Central ID: PMC7385689]. https://doi.org/10.1001/jamacardio.2020.3557.
-
16.
Xiong TY, Redwood S, Prendergast B, Chen M. Coronaviruses and the cardiovascular system: acute and long-term implications. Eur Heart J. 2020;41(19):1798-800. [PubMed ID: 32186331]. [PubMed Central ID: PMC7454513]. https://doi.org/10.1093/eurheartj/ehaa231.
-
17.
Diorio C, Henrickson SE, Vella LA, McNerney KO, Chase J, Burudpakdee C, et al. Multisystem inflammatory syndrome in children and COVID-19 are distinct presentations of SARS-CoV-2. J Clin Invest. 2020;130(11):5967-75. [PubMed ID: 32730233]. [PubMed Central ID: PMC7598044]. https://doi.org/10.1172/JCI140970.
-
18.
Bartekova M, Radosinska J, Jelemensky M, Dhalla NS. Role of cytokines and inflammation in heart function during health and disease. Heart Fail Rev. 2018;23(5):733-58. [PubMed ID: 29862462]. https://doi.org/10.1007/s10741-018-9716-x.
-
19.
Janczewski AM, Kadokami T, Lemster B, Frye CS, McTiernan CF, Feldman AM. Morphological and functional changes in cardiac myocytes isolated from mice overexpressing TNF-alpha. Am J Physiol Heart Circ Physiol. 2003;284(3):H960-9. [PubMed ID: 12578819]. https://doi.org/10.1152/ajpheart.0718.2001.
-
20.
Duncan DJ, Yang Z, Hopkins PM, Steele DS, Harrison SM. TNF-alpha and IL-1beta increase Ca2+ leak from the sarcoplasmic reticulum and susceptibility to arrhythmia in rat ventricular myocytes. Cell Calcium. 2010;47(4):378-86. [PubMed ID: 20227109]. [PubMed Central ID: PMC2877880]. https://doi.org/10.1016/j.ceca.2010.02.002.
-
21.
Izumi T, Nishii M. Diagnostic and prognostic biomarkers in acute myocarditis. Interleukin-10. Herz. 2012;37(6):627-31. [PubMed ID: 22941230]. https://doi.org/10.1007/s00059-012-3661-6.
-
22.
Santoro F, Tarantino N, Ferraretti A, Ieva R, Musaico F, Guastafierro F, et al. Serum interleukin 6 and 10 levels in Takotsubo cardiomyopathy: Increased admission levels may predict adverse events at follow-up. Atherosclerosis. 2016;254:28-34. [PubMed ID: 27680775]. https://doi.org/10.1016/j.atherosclerosis.2016.09.012.
-
23.
Ali N. Elevated level of C-reactive protein may be an early marker to predict risk for severity of COVID-19. J Med Virol. 2020;92(11):2409-11. [PubMed ID: 32516845]. [PubMed Central ID: PMC7301027]. https://doi.org/10.1002/jmv.26097.
-
24.
Ghahramani S, Tabrizi R, Lankarani KB, Kashani SMA, Rezaei S, Zeidi N, et al. Laboratory features of severe vs. non-severe COVID-19 patients in Asian populations: a systematic review and meta-analysis. Eur J Med Res. 2020;25(1):30. [PubMed ID: 32746929]. [PubMed Central ID: PMC7396942]. https://doi.org/10.1186/s40001-020-00432-3.
-
25.
Aeschlimann FA, Misra N, Hussein T, Panaioli E, Soslow JH, Crum K, et al. Myocardial involvement in children with post-COVID multisystem inflammatory syndrome: a cardiovascular magnetic resonance based multicenter international study-the CARDOVID registry. J Cardiovasc Magn Reson. 2021;23(1):140. [PubMed ID: 34969397]. [PubMed Central ID: PMC8717054]. https://doi.org/10.1186/s12968-021-00841-1.
-
26.
Valverde I, Singh Y, Sanchez-de-Toledo J, Theocharis P, Chikermane A, Di Filippo S, et al. Acute Cardiovascular Manifestations in 286 Children With Multisystem Inflammatory Syndrome Associated With COVID-19 Infection in Europe. Circulation. 2021;143(1):21-32. [PubMed ID: 33166189]. https://doi.org/10.1161/CIRCULATIONAHA.120.050065.
-
27.
Benvenuto S, Simonini G, Della Paolera S, Abu Rumeileh S, Mastrolia MV, Manerba A, et al. Cardiac MRI in midterm follow-up of MISC: a multicenter study. Eur J Pediatr. 2023;182(2):845-54. [PubMed ID: 36484863]. [PubMed Central ID: PMC9734924]. https://doi.org/10.1007/s00431-022-04748-6.