Abstract
Background:
Traumatic brain injury (TBI) can result from blunt trauma or acceleration/deceleration force and is considered to be one of the most important public health problems. Many patients of TBI lose professional competence and independence. They may eventually suffer serious sequelae, such as cognitive impairment, memory loss, and long-term headache. In craniocerebral trauma patients, mild traumatic brain injury accounts for 70% - 80% of the cases. Early diagnosis and intervention can decrease the post-concussional sequelae for mild TBI (mTBI).Objectives:
To investigate the value of diffusion kurtosis imaging (DKI) in mild traumatic brain injury (mTBI) patients.Patients and Methods:
Conventional MRI sequences (T1 and T2 weighted images) and DKI scans of 25 healthy controls and 24 mTBI patients were obtained. Regions of interests (ROIs) were drawn and analyzed on several planes including white matter, deep gray matter nuclei, peri-contusion and contusion lesion on fused fractional anisotropy (FA), mean diffusion (MD) maps, and mean kurtosis (MK) maps, respectively. FA, MD, and MK values were measured in a certain region (he area of bilateral centrum semiovale (CS), corona radiate (CR), caudate nucleus head (CNH), genu of corpus callosum (CCG), corpus callosum splenium (CCS), anterior limb of the internal capsule (ALIC), posterior limb of internal capsule (PLIC), external capsule (EC), lenticular nucleus (LN), and thalamus). Student’s t test was used to compare the average values of FA, MD, and MK between mTBI patients and healthy controls. Paired t-test was also used to compare contusion lesions and mirror symmetrical areas.Results:
FA and MK values were significantly different in all of the white matter and grey matter, while MD values among the various ROIs were insignificantly different in the healthy control group. In mTBI patients, the FA values of genu of corpus callosum, splenium of corpus callosum, and external capsule were decreased. Whereas, MD value of genu of corpus callosum in trauma group was increased. Except for the head of the caudate nucleus and posterior limb of the internal capsule, the MK values of the rest of the ROIs in the trauma group decreased significantly. Moreover, compared with the contra-lateral, there were more significant contusion lesions of FA and MK reductions than increased MD values. FA and MD values of peri-contusion had no significant difference compared with the contra-lateral while MK values decreased significantly.Conclusion:
Compared with conventional MRI sequences, DKI can detect micro white matter injury, and MK parameter is more sensitive than FA and MD. In addition, DKI can find these changes not only in the white matter but also in deep gray matter nucleus, making it a promising imaging tool to assist the detection of mild traumatic brain injury.Keywords
1. Background
Traumatic brain injury (TBI) can result from blunt trauma or acceleration/ deceleration force and is considered to be one of the most important public health problems. The definition of mild traumatic brain injury (mTBI) refers to a less unhealthy clinical state i.e., Glasgow Coma Scale (GCS) score of 13 - 15, and, loss of consciousness < 30 minutes (1, 2). Early diagnosis and intervention can decrease the post-concussional sequelae for mTBI. However, it is difficult to make an early diagnosis by CT and routine MRI (3). There are about 1.1 million cases of traumatic brain injury patients in the United States each year, and 30% of these people experience long-term cognitive impairment; while 20% cannot be employed again, leading to economic losses of about $17 billion (4). Every year, there are more than 40 million global incidents (5). Many TBI patients lose professional competence and independence. They may eventually suffer serious sequelae, such as cognitive impairment, memory loss, and long-term headache, which brings huge economic burden to the family and society. The complex TBI pathological mechanism involves rapidly progressive injury. It is crucial in predicting the prognosis of patients to visualize the extent of lesions and determine the progress of the course early and accurately in traumatic brain injury. In craniocerebral trauma patients, mild traumatic brain injury accounts for 70% - 80% of the cases (6-8). Therefore, it is urgent to improve the diagnosis and treatment of mTBI.
CT examination is the first line modality for evaluating patients with TBI, because of its high density and time resolution, the skull fracture and brain hemorrhage can be detected easily. However, because of bony artifacts and poor soft tissue resolution, CT has obvious limitations; mTBI including diffuse axonal injury (DAI) is difficult to be diagnosed by CT. MRI is a very valuable modality in detecting brain diseases due to its high soft tissue resolution. However, it is difficult to diagnose mTBI by conventional MRI. Additionally, CT and conventional MRI performance is not strongly correlated with the severity and prognosis of patients constantly (9-11). Thus, it is essential to find suitable method or biomarkers to detect mTBI.
With the continuous development of functional magnetic resonance imaging (fMRI) in the recent years, more advanced technologies of fMRI are applied for the diagnosis and prognosis of central nervous system, including diffusion tensor imaging (DTI) and diffusion kurtosis imaging (DKI), diffusion weighted imaging (DWI), and susceptibility weighted imaging (SWI). Recently, many researchers use DTI and DKI to explore mTBI. Zhuo et al. (11) investigated DTI and DKI parameters of several white and grey matter regions in mild controlled cortical impact (CCI) injury in a rat model both at the acute (2 hours) and sub-acute periods (7 days) after injury and found increased mean kurtosis (MK) value in the contra-lateral cortex, hippocampus, external capsule and corpus callosum compared to the baseline in the sub-acute phase. Mean diffusion (MD) and fractional anisotropy (FA) values did not change. The increase in MK was consistent with the reactive astrogliosis observed from immunohistochemistry analysis. Grossman et al. (4) found that cognitive impairment was associated with MK values of the thalamus and internal capsule. Thus, MK could be an index to predict the existence of permanent brain damage and cognitive prognosis.
The above literatures show that DKI is a feasible method to detect mTBI. Meanwhile, the results of previous investigations are inconsistent.
2. Objectives
The purpose of this research is to detect whether there is change of regions of interest (ROIs) or not in mild traumatic brain injury by DKI.
3. Patients and Methods
3.1. Patients
Initially, a total of 27 healthy subjects (control) and 28 mTBI patients were recruited for this study. Due to excessive head movement during scanning, imaging data from two healthy controls and four mTBI patients were excluded and consequently a total of 49 participants were included in this study. Twenty-four mTBI patients and 25 healthy subjects were enrolled in this study. The mTBI patients were recruited from the First Affiliated Hospital of Jinan University. Gender and age matched healthy subjects were recruited from the local community through advertisements (December 2012 to January 2015). All subjects voluntarily signed the informed consent form. The study was approved by the human research ethics committee of Jinan University.
The clinical states of the patients were evaluated with the Glasgow Coma Scale (GCS). Inclusion criteria for mTBI patients were as follows: 26 - 55 years old, all of them had a history of craniocerebral trauma within 1 month, and a GCS score of 13 - 15, able to give voluntary informed consent, and qualified to undergo MRI scans. Exclusion criteria were as follows: craniocerebral operation histories, serious medical or neurological illness, pregnancy or breast-feeding, and having metallic implants or other contraindications to MRI scan.
Inclusion criteria for healthy subjects were as follows: 26 - 55 years old, able to give voluntary informed consent, no history of medical or neurological illness, no significant family history of neurological illness, not currently taking any prescriptions, and presented normally on T2 fluid attenuated inversion recovery (FLAIR) imaging. Exclusion criteria for healthy subjects were as follows: pregnancy, breast-feeding, and metallic implants or other contraindications to MRI scan.
3.2. MR Imaging
All MR images were obtained by using a 3.0 T MR scanner (GE DISCOVERY 750) equipped with an eight-channel head coil. Foam pads were used to limit head motion. All of conventional sequences included three- dimensional T1-BRAVO images, T2-weighted images, and T2-weighted FLAIR images. DKI has 30 diffusion gradient directions; other scanned parameters are shown in Table 1.
MRI Scanned Parameters for 27 Healthy Subjects and 28 mTBI Patients
Sequence | TR/TE, ms | Slice thickness/Interval, mm | Bandwidth | NEX | Matrix | FOV, cm | Imaging time, s |
---|---|---|---|---|---|---|---|
3DT1 BRAVO | 8.2/3.2 | 1/0 | 31.25 | 1 | 256 × 256 | 24 × 24 | 248 |
T2WI | 6739/103 | 5/1.5 | 83.333 | 1 | 512 × 512 | 24 × 24 | 48 |
DWI (b = 0, 1000 s/mm2) | 3000/min | 5/1.5 | 250 | 4 | 160 × 160 | 24 × 24 | 42 |
T2FLAIR | 8400/145 | 5/1.5 | 62.50 | 1 | 256 × 256 | 24 × 24 | 135 |
DKI (b = 0, 1000, 2000 s/mm2) | 4500/min | 4/0 | 250 | 2 | 128 × 128 | 24 × 24 | 725 |
3.3. DKI image Processing with ROI Analysis
DKI raw data analyses were performed on 3.0 T GE DISCOVERY post-processing workstation by using ADW-4.2 Function software. First, 3D-T1BRAVO image with DKI image were combined to get the superimposed image to highlight the anatomical sites. Next, ROIs were drawn and analyzed on fused FA (Figure 1A), MD maps (Figure 1B), and MK maps (Figure 1C). ROIs were selected on the area of bilateral centrum semiovale (CS), corona radiate (CR), caudate nucleus head (CNH), genu of corpus callosum (CCG), corpus callosum splenium (CCS), anterior limb of the internal capsule (ALIC), posterior limb of internal capsule (PLIC), external capsule (EC), lenticular nucleus (LN), and thalamus, which were normal in conventional MRI in both control and patient groups. The size range of ROIs is about 30 to 200 mm2. Last, twenty two lesions were selected from contusion lesions (Figure 2A) and FA, MD, and MK values were measured in contusion lesions (Figure 2B) and the pericontusional region in the maximum level of selected lesions and the contralateral mirror parts avoiding the effect of intracranial hematoma. Three appropriate sizes around the contusion lesions were selected as pericontusional regions. All ROIs were drawn manually on its largest axial slice.
A control subject. A, Regions of interest (ROIs) were drawn on several planes including white matter and deep gray matter nuclei on fused fractional anisotropy (FA); B, Mean diffusion (MD) maps; C, Mean kurtosis (MK) maps.
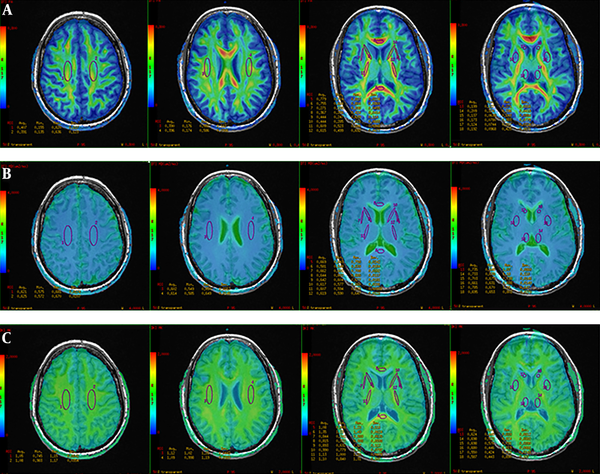
A typical right frontal contusion of a mild traumatic brain injury (mTBI) patient. A, Imaging features of contusion on conventional MRI. B, Fused fractional anisotropy (FA), mean diffusion (MD) and mean kurtosis (MK) images show regions of contusion, peri-contusion and contralateral mirror parts.
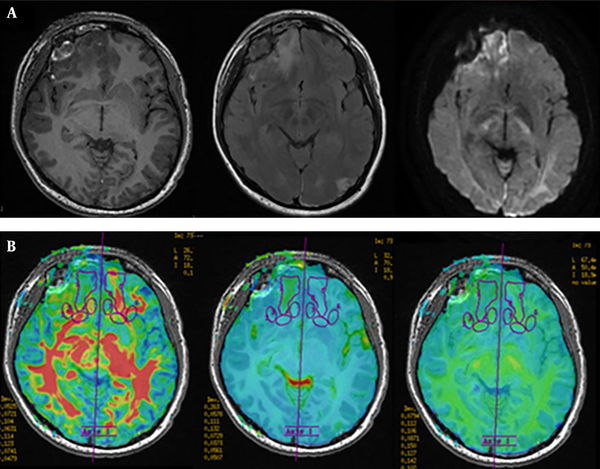
3.4. Statistical Analysis
All statistical analyses were performed with SPSS for Windows, version 16.0 software package (SPSS Inc., Ill., Chicago, USA). Statistical analysis of categorical data was conducted with χ2 test and Student’s t test for demographic and clinical data. The one-way analysis of variance (ANOVA) was analyzed to compare different anatomical ROIs of healthy controls. Student’s t test was used to compare the average values of FA, MD, and MK between mTBI patients and healthy controls. Paired t-test was also used to compare contusion lesions and mirror symmetrical areas. Statistical significance was set at P < 0.05 for all tests.
4. Results
4.1. Demographic and Clinical Features
The general clinical data of 24 mTBI patients are shown in Table 2.
General Clinical Data of 24 mTBI Patients
Number | Age, y | Time from injury to scanning, d | Causes | GCS score | Injury on imaging |
---|---|---|---|---|---|
1 | 37 | 10 | Tumble | 14 | Contusion, fracture, subarachnoid hemorrhage |
2 | 36 | 13 | Hit | 15 | Contusion |
3 | 52 | 8 | Traffic accident | 15 | Cortical contusion |
4 | 46 | 22 | Traffic accident | 15 | No intracranial lesions |
5 | 55 | 18 | Traffic accident | 15 | No intracranial lesions |
6 | 31 | 19 | Blunt hurt | 15 | No intracranial lesions |
7 | 55 | 12 | Traffic accident | 13 | Contusion, epidural hematoma, subarachnoid hemorrhage |
8 | 49 | 5 | Traffic accident | 14 | Contusion, subdural effusion, subarachnoid hemorrhage, fracture |
9 | 29 | 27 | Fall down | 15 | Contusion, fracture, subdural effusion |
10 | 26 | 7 | Tumble | 15 | Epidural hematoma |
11 | 34 | 3 | Blunt hurt | 15 | No intracranial lesions |
12 | 26 | 11 | Tumble | 15 | Cortical contusion |
13 | 51 | 7 | Tumble | 15 | Contusion, subdural hematoma, fracture |
14 | 47 | 8 | Tumble | 13 | Subdural hematoma, contusion, subarachnoid hemorrhage |
15 | 33 | 4 | Traffic accident | 15 | Epidural hematoma, fracture |
16 | 41 | 30 | Traffic accident | 13 | Contusion, subarachnoid hemorrhage, fracture |
17 | 30 | 9 | Tumble | 13 | Epidural hematoma, contusion, fracture |
18 | 45 | 12 | Tumble | 15 | Contusion, fracture, epidural hematoma |
19 | 28 | 10 | Traffic accident | 15 | Cortical contusion |
20 | 47 | 3 | Tumble | 15 | Subcortical hematoma, fracture |
21 | 50 | 14 | Blunt hurt | 15 | Subcortical hematoma |
22 | 31 | 1 | Hit | 15 | No intracranial lesions |
23 | 26 | 8 | Traffic accident | 15 | Cortical contusion, frature |
24 | 50 | 22 | Traffic accident | 15 | No intracranial lesions |
There were 24 patients with mTBI [(20 males and 4 females), 26 - 55 years old (mean 37.5 years)] and 25 subjects in the normal control group [(20 males and 5 females), 26 - 55 years old (mean 36.12 years)]. There was no significant difference between the two groups in age (z = 0.234, P = 0.82, Wilcoxon test). Chi-square test showed there were no significant differences between the two groups in terms of gender distribution (χ2 = 0.000, P = 1.000).
4.2. FA, MD, and MK Values of ROIs in the Healthy Brain and mTBI Patients
The results of bilateral FA, MD, and MK values in healthy controls showed that there was no statistically significant difference on both sides. Thus, the mean value of bilateral metrics of FA, MD and MK values of ROIs in 25 healthy subjects are demonstrated in Table 3.
Comparisons of Parameters Between mTBI Group (n = 24) and Healthy Group (n = 25)a
ROI | FA | MD, μm2/ms | MK | |||
---|---|---|---|---|---|---|
Healthy group | mTBI group | Healthy group | mTBI group | Healthy group | mTBI group | |
CS | 0.424 ± 0.002 | 0.422 ± 0.037 | 1.083 ± 0.061 | 1.110 ± 0.050 | 1.033 ± 0.057 | 1.001 ± 0.069b |
CR | 0.487 ± 0.015 | 0.469 ± 0.048 | 1.045 ± 0.085 | 1.068 ± 0.044 | 1.088 ± 0.025 | 1.048 ± 0.038b |
CNH | 0.134 ± 0.032 | 0.146 ± 0.041 | 1.055 ± 0.027 | 1.089 ± 0.061 | 0.518 ± 0.086 | 0.496 ± 0.087 |
CCG | 0.791 ± 0.021 | 0.733 ± 0.072b | 1.100 ± 0.970 | 1.136 ± 0.057b | 0.973 ± 0.081 | 0.864 ± 0.092b |
CCS | 0.820 ± 0.042 | 0.762 ± 0.060b | 1.082 ± 0.072 | 1.086 ± 0.112 | 1.159 ± 0.179 | 0.970 ± 0.203b |
ALIC | 0.504 ± 0.017 | 0.494 ± 0.062 | 1.067 ± 0.017 | 1.092 ± 0.088 | 0.934 ± 0.023 | 0.876 ± 0.019b |
PLIC | 0.670 ± 0.043 | 0.674 ± 0.041 | 1.045 ± 0.065 | 1.052 ± 0.106 | 1.145 ± 0.038 | 1.123 ± 0.076 |
EC | 0.392 ± 0.010 | 0.325 ± 0.054b | 1.038 ± 0.042 | 1.053 ± 0.077 | 0.771 ± 0.060 | 0.750 ± 0.087b |
LN | 0.177 ± 0.006 | 0.183 ± 0.031 | 1.044 ± 0.069 | 1.047 ± 0.119 | 0.667 ± 0.071 | 0.615 ± 0.085b |
Thalamus | 0.248 ± 0.009 | 0.253 ± 0.030 | 1.057 ± 0.037 | 1.074 ± 0.076 | 0.705 ± 0.071 | 0.664 ± 0.082b |
The results of FA, MD, and MK values of ROIs in 24 mTBI patients is also displayed in Table 3.
FA values of different ROIs including white matter and gray matter nuclei were significantly different in healthy controls. The FA values of these sites, from CNH, LN, thalamus, EC, CS, CR, ALIC, PLIC, CCG, to CCS, increased gradually. The MD values among different regions were not significantly different. MK values of ROIs were also significantly different between each other. The MK values of these sites, from CNH, LN, thalamus, EC, ALIC, CCG, CS, CR, PLIC, to CCS increased gradually. This order is not similar to FA when it comes to some white matter regions.
Compared with the normal control group, the FA values of CCG, CCS, and EC in the mTBI group was decreased (CCG: t = 4.734, P = 0.042; CCS: t = 5.743, P = 0.029; EC: t = 4.522, P = 0.046) (Table 3); the MD values of CCG in the mTBI group was increased (t = -6.174, P = 0.025) (Table 3). Except for CNH and PLIC, the MK values of the rest ROIs in the mTBI group were significantly lower than the healthy control group by two-sample t-test (CS: t = 5.093, P = 0.036; CR: t = 5.252, P = 0.034; CCG: t = 21.377, P = 0.002; CCS: t = 24.067, P = 0.002; ALIC: t = 11.600, P = 0.007; EC: t = 5.824, P = 0.028; LN: t = 8.554, P = 0.013; Thalamus: t = 11.371, P = 0.012) (Table 3).
4.3. FA, MD, and MK Values of Contusion Lesions and Pericontusional Regions
FA, MD, and MK values of contusion lesions and pericontusional regions in 24 mTBI patients is demonstrated in Table 4.
Comparisons of Parameters Between Contusion Lesions and Contralateral Mirror Sites in 24 mTBI Patientsa
FA | MD, μm2/ms | MK | |||||||||
---|---|---|---|---|---|---|---|---|---|---|---|
Con | Con-contr | Peri-con | Peri-contr | Con | Con-contr | Peri-con | Peri-contr | Con | Con-contr | Peri-con | Peri-contr |
0.124 | 0.359 | 0.220 | 0.169 | 1.701 | 0.978 | 1.610 | 1.030 | 0.614 | 0.781 | 0.583 | 0.666 |
0.193 | 0.537 | 0.255 | 0.389 | 1.232 | 0.894 | 1.320 | 1.110 | 0.788 | 0.981 | 0.744 | 0.707 |
0.237 | 0.318 | 0.323 | 0.307 | 0.882 | 0.899 | 0.918 | 0.961 | 0.665 | 0.699 | 0.548 | 0.460 |
0.163 | 0.238 | 0.246 | 0.286 | 1.022 | 1.023 | 0.905 | 1.000 | 0.578 | 0.767 | 0.664 | 0.827 |
0.121 | 0.356 | 0.169 | 0.409 | 1.320 | 0.970 | 1.000 | 0.996 | 0.653 | 0.802 | 0.439 | 0.609 |
0.087 | 0.245 | 0.249 | 0.118 | 2.150 | 1.010 | 1.270 | 0.989 | 0.456 | 0.667 | 0.672 | 0.653 |
0.101 | 0.191 | 0.121 | 0.145 | 1.320 | 1.340 | 1.510 | 2.070 | 0.597 | 0.675 | 0.404 | 0.592 |
0.101 | 0.174 | 0.288 | 0.128 | 1.100 | 1.870 | 1.500 | 0.987 | 0.454 | 0.477 | 0.480 | 0.785 |
0.068 | 0.365 | 0.088 | 0.207 | 2.310 | 0.942 | 1.250 | 1.060 | 0.441 | 0.767 | 0.623 | 0.558 |
0.116 | 0.349 | 0.122 | 0.230 | 2.170 | 0.906 | 0.879 | 1.990 | 0.472 | 0.715 | 0.418 | 0.625 |
0.125 | 0.232 | 0.446 | 0.334 | 1.290 | 0.998 | 1.800 | 0.952 | 0.708 | 0.753 | 0.649 | 0.663 |
0.119 | 0.239 | 0.134 | 0.155 | 1.820 | 1.240 | 1.740 | 1.120 | 0.464 | 0.581 | 0.542 | 0.631 |
0.132 | 0.253 | 0.196 | 0.198 | 2.060 | 1.180 | 1.410 | 0.967 | 0.417 | 0.646 | 0.517 | 0.588 |
0.113 | 0.161 | 0.240 | 0.117 | 2.080 | 1.060 | 1.100 | 1.189 | 0.437 | 0.631 | 0.454 | 0.526 |
0.172 | 0.228 | 0.178 | 0.364 | 1.570 | 1.060 | 1.460 | 1.050 | 0.516 | 0.666 | 0.688 | 0.745 |
0.087 | 0.271 | 0.010 | 0.330 | 3.690 | 2.200 | 4.410 | 2.340 | 0.622 | 0.556 | 0.598 | 0.594 |
0.107 | 0.127 | 0.242 | 0.190 | 1.230 | 0.923 | 0.797 | 0.933 | 0.665 | 0.740 | 0.509 | 0.616 |
0.179 | 0.247 | 0.317 | 0.327 | 2.480 | 2.320 | 2.140 | 2.177 | 0.546 | 0.627 | 0.685 | 0.769 |
0.103 | 0.134 | 0.142 | 0.218 | 1.200 | 1.580 | 5.130 | 2.380 | 0.573 | 0.727 | 0.487 | 0.493 |
0.166 | 0.295 | 0.176 | 0.263 | 2.150 | 1.140 | 1.600 | 1.090 | 0.460 | 0.686 | 0.599 | 0.612 |
0.068 | 0.110 | 0.238 | 0.118 | 2.410 | 1.790 | 1.050 | 2.507 | 0.488 | 0.579 | 0.451 | 0.486 |
0.183 | 0.239 | 0.294 | 0.310 | 0.649 | 0.857 | 0.811 | 0.875 | 0.866 | 0.625 | 0.792 | 0.779 |
FA values were significantly lower in the contusion lesions than in contralateral mirror sites (t = 6.602, P = 0.000). The MD values of the lesions were significantly different from that of the contralateral (t = 3.830, P = 0.001). Both FA and MD values of pericontusional regions were not significantly different with the contralateral (FA: t = -1.055, P = 0.304. MD: t = 1.404, P = 0.175), whereas the MK values of lesions and pericontusional regions were lower than that of the contralateral (lesions: t = -4.735, P = 0.000. pericontusional regions: t = -3.182, P = 0.004).
5. Discussion
DTI, DKI and DWI techniques not only reflect the morphological changes, but also can show the pathophysiologic changes. DTI in mTBI supplies quantitative measures of micro-structural integrity and organization in the living system and is thus more suitable for detecting subtle injury which is not evident with conventional MRI. Parameters of DTI can be used as a prediction index of the prognosis of patients with brain injury, especially the fractional anisotropy (FA) value (4, 12-14). Previous DTI researches of TBI have discovered decreased FA values in several white matter regions, both within contused lesions and in tissue appearing normal on conventional MRI (15-17). Others have shown that DTI can detect the micro injury of white matter, particularly in the brainstem, corpus callosum, and subcortical white matter, which are connected to the basal ganglia, thalamus, and the function of the cerebellum (6, 18). Diffusion kurtosis imaging (DKI) is a newly developed imaging technology based on DTI and describes the non-Gaussian distribution of water molecules diffusion properties of biological tissues. Conventional wisdom holds that the movement of water molecules through biological tissues follow the Gaussian distribution. In fact, as the result of the existence of diffusion barriers such as in nerve fibers and cell membranes or organelles, the probability distribution of water molecular diffusion deviates from Gaussian FUNC. The degree of deviation from Gaussian distribution is the diffusion kurtosis. The main parameters of DKI include mean kurtosis (MK), kurtosis anisotropy (KA), and radial kurtosis (RK). MK as the most frequently used parameter is a dimensionless parameter that reflects thelimited degree of diffusion. MK value depends on the complexity or integrity of the tissue structure of region-of-interest (ROI), such that the more complex the structure, the greater the MK value (19). Compared with DTI, MK not only reflects the anisotropic environment, but also offers quantification of the microstructural integrity of the white matter. Additionally, in contrast to the second-order tensor of DTI technology, DKI is a fourth-order tensor imaging technology that can detect the complex of crossing fibers which is the second-order tensor’s limitation. Therefore, DKI is more sensitive in detecting the microstructural integrity of the white matter (20-22).
From this study, we can confirm three findings. First, we demonstrated that FA and MK values were significantly different between the white matter (CS, CR, CCG, CCS, ALIC, PLIC, EC) and the deep gray matter nuclei (CNH, LN, and Thalamus) in normal controls. Second, compared with normal controls, reduced MK values were detected in a more extensive area than FA reduction; apart from in CCG, CCS, and EC, reductions in MK values were seen in the CS, CR, ALIC, LN, and thalamus. Increased MD values only occurred at CCG significantly. Third, FA, MD, and MK values were significantly different between contusion lesions and the mirror areas. Only MK values were reduced significantly in pericontusional regions compared with the mirror area. This study compared DKI and DTI when it comes to analyzing white matter and deep gray nuclei injury to understand which technology is more effective with mTBI.
This study demonstrates that FA and MD values were not different between the two sides of the same ROI in a healthy person. The result is consistent with previous studies (23-25). Our result shows that FA and MK values of CCS are maximum while that for CNH is minimum. The FA of CCS is consistent with previous studies (24), which might be due to the high density and close arrangement of the neural axon in CCS (26, 27). FA value was statistically different between gray matter nuclei. The FA value of the thalamus is the largest, which might be due to the spinothalamic tract (STT) causing the increased FA value through the thalamus. Because the lentiform nucleus includes putamen and globus pallidus, which contains some myelinated fiber bundles, the FA value of LN is higher than that of the head of the caudate nucleus. In addition to the high density of the neural axon, the largest MK values detected on CCS are related to the complexity of crossing fibers. The sites ordered by increasing MK value do not follow the same trend as that for FA when it comes to some white matter regions. This may indicate that the diversity and complexity of different crossing fibers of the white matter could lead to different deviation of non-Gaussian water diffusion in contrast to conventional DTI which needs further confirmed pathological and histological studies. Our study shows that there was no statistically significant difference between MD values of ROIs; this result is consistent with the theory that the MD value has nothing to do with the direction factor.
The increased MD values in CCG and the reduced FA and MK values in some ROIs of mTBI patients are consistent with the results from previous studies (13, 14, 17). These values may be associated with trauma sequelae such as headache and cognitive impairment (3, 4, 28). Grossman et al. (4) demonstrated that reductions in FA and MK values occurred across the thalamus, internal capsule, external capsule, corpus callosum, cingulate, corona radiata, CS and deep gray matter nuclei at an early stage of mTBI, accompanied with the increased MD value. Interestingly, only the MK value of the thalamus was significantly reduced in the group that exhibited cognitive impairment at a later stage. As the previous studies emphasized, the diffusion rate of water molecules follows the Gaussian distribution in an unrestricted environment, but in the brain tissue, this is restricted by organelles or cell membranes in vivo. Therefore, the diffusion of water molecules in biological tissue displays non-Gaussian distribution. DKI is more sensitive in detecting white microstructure alternation or integrity than DTI. We detected reduced MK values across a more extensive area than FA reduction; whereas, increased MD was observed only in the CCG. In addition to white matter, MK value decreased significantly in some of the deep grey nuclei including the thalamus and LN. Therefore, MK appears to be also more sensitive to subtle alternations in deep gray matter nuclei than FA and MD. Immunohistochemistry confirmed that the MK value could reflect reactive astrocytes (11). MK value decline may have bearing on heterogeneous diffusion caused by the decreased number of neurons and degeneration of the axon myelin sheath (19, 21, 29).
In contusions, it is suggested that acute lesions mainly composed of cytotoxic edema would lead to decreased diffusivity and increased FA values (30). However, most of the patients in our study were at sub-acute or chronic stage when examined, which means that a larger contribution of vasogenic edema in lesions would cause the opposite outcome. Destruction of cellular structures would cause water molecules to diffuse faster, which could result in the decline in FA value, the mean diffusion kurtosis and increase in the mean diffusivity (31). The reduced FA and MK values in contusions are consistent with pathological changes that occur in pathology. Among these metrics, only the reduction in MK values can be detected significantly in pericontusions by ROI analysis. The fact that DKI can detect subtle changes in pericontusions may be the reason why MK is more sensitive to changes in microstructure integrity.
Our present study had limitations and needs future work. First, we did not analyze the correlation between the age and ROIs for small sample size of healthy controls. In the process of development of the human brain, brain structure and morphology changes due to cerebral white matter density and capacity increase in the early teens and maturity while it gradually reduces after old age (32-35). We will enlarge the sample size as our next step for further research. Second, because the ROIs were drawn manually, the reproducibility of measurements may vary; all parameters of ROIs were measured blindly by two experimenters. Third, in this paper, we only analyzed the mTBI for a short time period (injury within one month), and the follow-up information on therapy effects, and prognosis should be collected through interview, telephone call, and questionnaire. Besides, the relationship between behavioral disorders, cognitive impairment and the abnormal connectivity caused by damaged brain white matter fiber tracts of mTBI patients need to be further studied in the future.
In conclusion, DKI can detect micro white matter injury, and MK is more sensitive than FA and MD. In addition, MK can find these changes not only in the white matter but also in the deep gray matter nucleus, making it a promising imaging parameter to assist the detection of mild traumatic brain injury. It may also be a latent indicator for predicting whether patients with mild traumatic brain injury will have cognitive impairment or not.
References
-
1.
Holm L, Cassidy JD, Carroll LJ, Borg J, Neurotrauma Task Force on Mild Traumatic Brain Injury of the WC. Summary of the WHO Collaborating Centre for Neurotrauma Task Force on Mild Traumatic Brain Injury. J Rehabil Med. 2005;37(3):137-41. [PubMed ID: 16040469]. https://doi.org/10.1080/16501970510027321.
-
2.
Ruff RM, Iverson GL, Barth JT, Bush SS, Broshek DK, N. A. N. Policy, et al. Recommendations for diagnosing a mild traumatic brain injury: a National Academy of Neuropsychology education paper. Arch Clin Neuropsychol. 2009;24(1):3-10. [PubMed ID: 19395352]. https://doi.org/10.1093/arclin/acp006.
-
3.
Grossman EJ, Inglese M, Bammer R. Mild traumatic brain injury: is diffusion imaging ready for primetime in forensic medicine? Top Magn Reson Imaging. 2010;21(6):379-86. [PubMed ID: 22158131]. [PubMed Central ID: PMC3985857]. https://doi.org/10.1097/RMR.0b013e31823e65b8.
-
4.
Grossman EJ, Ge Y, Jensen JH, Babb JS, Miles L, Reaume J, et al. Thalamus and cognitive impairment in mild traumatic brain injury: a diffusional kurtosis imaging study. J Neurotrauma. 2012;29(13):2318-27. [PubMed ID: 21639753]. [PubMed Central ID: PMC3430483]. https://doi.org/10.1089/neu.2011.1763.
-
5.
Gardner RC, Yaffe K. Epidemiology of mild traumatic brain injury and neurodegenerative disease. Mol Cell Neurosci. 2015;66(Pt B):75-80. [PubMed ID: 25748121]. [PubMed Central ID: PMC4461453]. https://doi.org/10.1016/j.mcn.2015.03.001.
-
6.
Zappala G, Thiebaut de Schotten M, Eslinger PJ. Traumatic brain injury and the frontal lobes: what can we gain with diffusion tensor imaging? Cortex. 2012;48(2):156-65. [PubMed ID: 21813118]. https://doi.org/10.1016/j.cortex.2011.06.020.
-
7.
Management of Concussion/m TG. VA/DoD Clinical Practice Guideline for Management of Concussion/Mild Traumatic Brain Injury. J Rehabil Res Dev. 2009;46(6):CP1-68. [PubMed ID: 20108447].
-
8.
Wang JY, Bakhadirov K, Devous MS, Abdi H, McColl R, Moore C, et al. Diffusion tensor tractography of traumatic diffuse axonal injury. Arch Neurol. 2008;65(5):619-26. [PubMed ID: 18474737]. https://doi.org/10.1001/archneur.65.5.619.
-
9.
Niogi SN, Mukherjee P, Ghajar J, Johnson C, Kolster RA, Sarkar R, et al. Extent of microstructural white matter injury in postconcussive syndrome correlates with impaired cognitive reaction time: a 3T diffusion tensor imaging study of mild traumatic brain injury. AJNR Am J Neuroradiol. 2008;29(5):967-73. [PubMed ID: 18272556]. https://doi.org/10.3174/ajnr.A0970.
-
10.
Heldt SA, Elberger AJ, Deng Y, Guley NH, Del Mar N, Rogers J, et al. A novel closed-head model of mild traumatic brain injury caused by primary overpressure blast to the cranium produces sustained emotional deficits in mice. Front Neurol. 2014;5:2. [PubMed ID: 24478749]. [PubMed Central ID: PMC3898331]. https://doi.org/10.3389/fneur.2014.00002.
-
11.
Zhuo J, Xu S, Proctor JL, Mullins RJ, Simon JZ, Fiskum G, et al. Diffusion kurtosis as an in vivo imaging marker for reactive astrogliosis in traumatic brain injury. Neuroimage. 2012;59(1):467-77. [PubMed ID: 21835250]. [PubMed Central ID: PMC3614502]. https://doi.org/10.1016/j.neuroimage.2011.07.050.
-
12.
Little DM, Kraus MF, Joseph J, Geary EK, Susmaras T, Zhou XJ, et al. Thalamic integrity underlies executive dysfunction in traumatic brain injury. Neurology. 2010;74(7):558-64. [PubMed ID: 20089945]. [PubMed Central ID: PMC2830915]. https://doi.org/10.1212/WNL.0b013e3181cff5d5.
-
13.
Messe A, Caplain S, Paradot G, Garrigue D, Mineo JF, Soto Ares G, et al. Diffusion tensor imaging and white matter lesions at the subacute stage in mild traumatic brain injury with persistent neurobehavioral impairment. Hum Brain Mapp. 2011;32(6):999-1011. [PubMed ID: 20669166]. https://doi.org/10.1002/hbm.21092.
-
14.
Inglese M, Makani S, Johnson G, Cohen BA, Silver JA, Gonen O, et al. Diffuse axonal injury in mild traumatic brain injury: a diffusion tensor imaging study. J Neurosurg. 2005;103(2):298-303. [PubMed ID: 16175860]. https://doi.org/10.3171/jns.2005.103.2.0298.
-
15.
Arfanakis K, Haughton VM, Carew JD, Rogers BP, Dempsey RJ, Meyerand ME. Diffusion tensor MR imaging in diffuse axonal injury. AJNR Am J Neuroradiol. 2002;23(5):794-802. [PubMed ID: 12006280].
-
16.
Salmond CH, Menon DK, Chatfield DA, Williams GB, Pena A, Sahakian BJ, et al. Diffusion tensor imaging in chronic head injury survivors: correlations with learning and memory indices. Neuroimage. 2006;29(1):117-24. [PubMed ID: 16084738]. https://doi.org/10.1016/j.neuroimage.2005.07.012.
-
17.
Rutgers DR, Fillard P, Paradot G, Tadie M, Lasjaunias P, Ducreux D. Diffusion tensor imaging characteristics of the corpus callosum in mild, moderate, and severe traumatic brain injury. AJNR Am J Neuroradiol. 2008;29(9):1730-5. [PubMed ID: 18617586]. https://doi.org/10.3174/ajnr.A1213.
-
18.
Hulkower MB, Poliak DB, Rosenbaum SB, Zimmerman ME, Lipton ML. A decade of DTI in traumatic brain injury: 10 years and 100 articles later. AJNR Am J Neuroradiol. 2013;34(11):2064-74. [PubMed ID: 23306011]. https://doi.org/10.3174/ajnr.A3395.
-
19.
Jensen JH, Helpern JA. MRI quantification of non-Gaussian water diffusion by kurtosis analysis. NMR Biomed. 2010;23(7):698-710. [PubMed ID: 20632416]. [PubMed Central ID: PMC2997680]. https://doi.org/10.1002/nbm.1518.
-
20.
Hui ES, Cheung MM, Qi L, Wu EX. Towards better MR characterization of neural tissues using directional diffusion kurtosis analysis. Neuroimage. 2008;42(1):122-34. [PubMed ID: 18524628]. https://doi.org/10.1016/j.neuroimage.2008.04.237.
-
21.
Wu EX, Cheung MM. MR diffusion kurtosis imaging for neural tissue characterization. NMR Biomed. 2010;23(7):836-48. [PubMed ID: 20623793]. https://doi.org/10.1002/nbm.1506.
-
22.
Steven AJ, Zhuo J, Melhem ER. Diffusion kurtosis imaging: an emerging technique for evaluating the microstructural environment of the brain. AJR Am J Roentgenol. 2014;202(1):W26-33. [PubMed ID: 24370162]. https://doi.org/10.2214/AJR.13.11365.
-
23.
Bammer R, Acar B, Moseley ME. In vivo MR tractography using diffusion imaging. Eur J Radiol. 2003;45(3):223-34. [PubMed ID: 12595107].
-
24.
Chepuri NB, Yen YF, Burdette JH, Li H, Moody DM, Maldjian JA. Diffusion anisotropy in the corpus callosum. AJNR Am J Neuroradiol. 2002;23(5):803-8. [PubMed ID: 12006281].
-
25.
Lipton ML, Kim N, Park YK, Hulkower MB, Gardin TM, Shifteh K, et al. Robust detection of traumatic axonal injury in individual mild traumatic brain injury patients: intersubject variation, change over time and bidirectional changes in anisotropy. Brain Imaging Behav. 2012;6(2):329-42. [PubMed ID: 22684769]. https://doi.org/10.1007/s11682-012-9175-2.
-
26.
Miao X, Qi M, Cui S, Guan Y, Jia Z, Hong X, et al. Assessing sequence and relationship of regional maturation in corpus callosum and internal capsule in preterm and term newborns by diffusion-tensor imaging. Int J Dev Neurosci. 2014;34:42-7. [PubMed ID: 24480665]. https://doi.org/10.1016/j.ijdevneu.2014.01.004.
-
27.
Keshavan MS, Diwadkar VA, DeBellis M, Dick E, Kotwal R, Rosenberg DR, et al. Development of the corpus callosum in childhood, adolescence and early adulthood. Life Sci. 2002;70(16):1909-22. [PubMed ID: 12005176].
-
28.
Grossman EJ, Jensen JH, Babb JS, Chen Q, Tabesh A, Fieremans E, et al. Cognitive impairment in mild traumatic brain injury: a longitudinal diffusional kurtosis and perfusion imaging study. AJNR Am J Neuroradiol. 2013;34(5):951-7. S1-3. [PubMed ID: 23179649]. [PubMed Central ID: PMC3908903]. https://doi.org/10.3174/ajnr.A3358.
-
29.
Fieremans E, Jensen JH, Helpern JA. White matter characterization with diffusional kurtosis imaging. Neuroimage. 2011;58(1):177-88. [PubMed ID: 21699989]. [PubMed Central ID: PMC3136876]. https://doi.org/10.1016/j.neuroimage.2011.06.006.
-
30.
Wilde EA, McCauley SR, Hunter JV, Bigler ED, Chu Z, Wang ZJ, et al. Diffusion tensor imaging of acute mild traumatic brain injury in adolescents. Neurology. 2008;70(12):948-55. [PubMed ID: 18347317]. https://doi.org/10.1212/01.wnl.0000305961.68029.54.
-
31.
Kou Z, Gattu R, Kobeissy F, Welch RD, O'Neil BJ, Woodard JL, et al. Combining biochemical and imaging markers to improve diagnosis and characterization of mild traumatic brain injury in the acute setting: results from a pilot study. PLoS One. 2013;8(11). e80296. [PubMed ID: 24260364]. [PubMed Central ID: PMC3833898]. https://doi.org/10.1371/journal.pone.0080296.
-
32.
Lebel C, Walker L, Leemans A, Phillips L, Beaulieu C. Microstructural maturation of the human brain from childhood to adulthood. Neuroimage. 2008;40(3):1044-55. [PubMed ID: 18295509]. https://doi.org/10.1016/j.neuroimage.2007.12.053.
-
33.
Sowell ER, Thompson PM, Toga AW. Mapping changes in the human cortex throughout the span of life. Neuroscientist. 2004;10(4):372-92. [PubMed ID: 15271264]. https://doi.org/10.1177/1073858404263960.
-
34.
Helpern JA, Adisetiyo V, Falangola MF, Hu C, Di Martino A, Williams K, et al. Preliminary evidence of altered gray and white matter microstructural development in the frontal lobe of adolescents with attention-deficit hyperactivity disorder: a diffusional kurtosis imaging study. J Magn Reson Imaging. 2011;33(1):17-23. [PubMed ID: 21182116]. [PubMed Central ID: PMC3492944]. https://doi.org/10.1002/jmri.22397.
-
35.
Latt J, Nilsson M, Wirestam R, Stahlberg F, Karlsson N, Johansson M, et al. Regional values of diffusional kurtosis estimates in the healthy brain. J Magn Reson Imaging. 2013;37(3):610-8. [PubMed ID: 23055442]. [PubMed Central ID: PMC3596978]. https://doi.org/10.1002/jmri.23857.