Abstract
Keywords
1. Introduction
Heat stroke, defined by a body temperature higher than 40 ºC, is a medical emergency that can cause changed mental status and corruption of multiple organ systems (1, 2). Exposure to high temperature could be passive in high environmental temperatures or exertional while performing strenuous work or drug induced such as topiramate, alcohol, amphetamines, and ecstasy (3). The major incidence of heat stroke deaths happens in very young or elderly people during summer heat waves. Young, hearty people may experience exertional heat stroke while performing tiring physical activity in temperate or hot climates (2). Elements that predispose to heat stroke collapse include pre - existing illness, cardiovascular disease, drug use (anticholinergics, antidiuretics, ecstasy, and alcohol), and poor fitness level (2). Rapid cooling, support of multi-organ function and treatment of disseminated intravascular coagulation and sepsis are the most effective clinical treatments, but approximately 30% of heat stroke survivors prove continuous reductions in neurological and peripheral tissue function despite these efforts (1, 2).
Central nervous system abnormalities are common in the literature (Table 1) since the brain is very sensitive to hyperthermia, especially cerebellum, because cerebellar Purkinje cells have selective vulnerability to heat stroke (4-6). There is complicated interaction between heat cytotoxicity, coagulation disorder, cytotoxine - mediated systemic inflammatory response causing multiorgan faliure, metabolic derangement and circulatory insufficiency (3, 7). Brain lesions in heat stroke on MRI are bilateral and symmetrical in most cases (8). Diffuse cerebellar edema, cortical edema especially in the vascular boundary zone and lesions in the external capsules, hippocampus, thalamus, brain stem and white matter were reported on MRI (1, 3-18).
Brain MR and MR Spectroscopy Findings of Reported Cases with Heat Stroke
Report | Patient's Age, Sex | Body Temperature | Brain Imaging Findings | Author |
---|---|---|---|---|
1 | 17 years, M | 106.8 ºF | T1 and T2 hyperintensity with enhancement in external capsules, adjacent lateral putamen and thalami and T2 hyperintensity with enhancement in the cerebellum | Mc Laughlin CT (1) |
2 | 78 years, F | 42 ºC | High signal intensity in cerebellum, midbrain and bilateral thalami only on DWI | Ookura R (6) |
3 | 41 years, M | Not reported | Restricted diffusion in the bilateral dentate nuclei and splenium of corpus callosum | Lee JS (7) |
4 | 36 years, M | 41 ºC | T2 hyperintensity with restricted diffusion in dentate nuclei cerebellar cortices, frontal and occipital and parietal cortical vascular boundry zones and thalami and T2 hyperintensity with facilitated diffusion in caudate nuclei | Muccio CF (3) |
5 | 4 months, M | 106 ºF | Restricted diffusion in the splenium of corpus callosum, cerebral peduncles and cerebellar dentate nuclei | Ray S (10) |
6 | 54 years, M | 108 ºF | T2 hyperintensity with restricted diffusion in cerebellum, caudate nuclei and hippocampi on the 5th day of admission and additional small T2 hyperintense dots in the subcortical white matter of cerebrum and contrast enhancement of the previously affected areas on the 9th day of admission | Mahajan S (9) |
7 | 56 years, M | 39.4 ºC | T2 hyperintensity with restricted diffusion in the right temporoparietooccipital cortex with early decreased T2 of adjacent subcortical white matter on the 3rd day of heat stroke and resolution of cortical signal changes but increased T2 signal of adjacent subcortical white matter on the 6th day of heat stroke | Fuse A (8) |
8 | 53 years, F | 40 ºC | Hyperintensity on DWI with intermediate values on ADC maps (vasogenic and/or cytotoxic edema) in the dentate nuclei, bilateral superior cerebellar peduncles and central tegmentum of midbrain and vasogenic edema in cerebral cortices on the 9th day of heat stroke and diffuse cerebral cerebellar atrophy on the 2nd month of heat stroke | Fushimi Y (5) |
9 | 2 years and 3 months, F | 40 ºC | Asymmetrical T2 hyperintense foci in the cerebellar hemispheres, patchy hyperintensities in both the frontal, parietal and occipital cortices at the vascular boundary zones and symmetrical T2 hyperintensities of hippocampi on the 5th day of heat stroke | Sudhakar PJ (4) |
10 | 47 years, M | Not reported | Restricted diffusion of cerebellar cortices at admission and cerebellar atrophy 2 months later | Fujioka Y (11) |
11 | 45 years, F | Unknown | T2 hyperintensity in the left striatum and patchy T2 hyperintensity in the white matter of cerebral hemispheres on the 2nd week and cerebellar atrophy on the 6th month of heat stroke | Biary N (12) |
12 | 79 years, F | 42.9 ºC | T2 hyperintensity in the pons suggesting central pontine myelinolysis | McNamee T (13) |
13 | 39 years, M | 42 ºC | Normal brain MRI findings | Deleu D (14) |
14 | N/A, N/A | Unknown | Cerebellar atrophy on the 10 weeks of heat stroke | Albukrek D (15) |
16 | 74 years, F | 42.9 ºC | Normal brain MRI findings | Bazille C (16) |
17 | 80 years, M | 42 °C | Mild cerebellar atrophy and increased signal in the central tegmentum of the midbrain at the decussation of superior cerebellar peduncles on the 12th day of heat stroke | Bazille C |
18 | 10 months, M | Unknown | Diffuse cerebral edema at first and cortical laminar necrosis in the vascular boundary zones on the 40th day of heat stroke | Akaboshi S (17) |
19 | 22 years, M | 39 ºC | Normal brain MRI findings on 6th day of heat stroke | Van Stavern GP (19) |
20 | 43 years, M | > 40°C | Dentate nuclei, Low NAA/Cr, Lac peak | Li J (18) |
21 | 64 years, M | > 40°C | Low NAA/Cr | Li J |
22 | 69 years, F | > 40°C | Low NAA/Cr | Li J |
23 | 60 years, M | > 40°C | Low NAA/Cr | Li J |
24 | 90 years, M | > 40°C | Low NAA/Cr | Li J |
25 | 75 years, F | > 40°C | Cerebellar peduncle,Low NAA/Cr | Li J |
26 | 67 years, F | > 40°C | Dentate nuclei and cerebellar cortical gray matter, Low NAA/Cr, Lac peak | Li J |
27 | 78 years, M | > 40°C | Low NAA/Cr | Li J |
We reviewed the literature and discussed brain MRI and MR spectroscopy findings of heat stroke, detailing the pathophysiology underlying the brain involvement and proposing excitotoxic injury as an alternative mechanism of brain damage in heat stroke.
2. Case Presentation
A four - year - old boy was admitted to our emergency clinic suffering from unconsciousness, vomiting, and fever. His highest fever was 39 ºC on his records. On physical examination, he was unconscious, having no verbal response and his Glasgow coma scale was 8. On admission, contrast - enhanced brain MRI and MR spectroscopy were performed using a 1.5 T whole - body superconducting MRI machine (Siemens, Avanto, Erlangen, Germany) with a head and neck coil. Multivoxel MR spectroscopy was performed by using the point - resolved two - dimensional chemical shift imaging - point - resolved spectroscopy (CSI - PRESS) [repetition time (TR): 1500; echo time (TE): 135/35 ms, field of view: 160 × 160 mm2, section thickness:15 mm, average: 4]. Axial FLAIR (TR: 8000; TE: 126 ms) and T2 (TR: 4580; TE: 91 ms), T1 (TR: 400; TE: 73 ms), diffusion weighted imaging (DWI) (TR: 2539; TE: 82 ms, b - factor: 1000 s/mm2) with corresponding apparent diffusion coefficient (ADC) maps, coronal FLAIR and postcontrast axial and coronal T1 weighted images were obtained on brain MRI. On MRI, in addition to brain lesions, acute pansinusitis and bilateral granulomatous otitis with enhancing effusion within tympanomastoid cavities were noted. Brain parenchymal lesions of T2 hyperintensity with diffusion restriction in cerebellar cortices, along the corticofugal fibers, lemniscal pathways and tegmental tracts of the brain stem, in the external capsules, hipothalami, thalami and hippocampi were noted. Signal changes in the uvulae and nodule of cerebellar vermis were more prominent than the rest of the cerebellum. Mean ADC values of the external capsule (478 × 10-6 mm2/s), thalami (638 × 10-6 mm2/s), affected brain - stem tract (555 × 10-6 mm2/s), cerebellum (358 × 10-6 mm2/s) were measured. Signal changes were bilateral and symmetric. There was no meningeal or parenchymal enhancement. On short echo (TE 35 ms) MR spectroscopy, glutamine/glutamate peaks were present at 2.4 ppm as well as 3.8 ppm (Figure 1). Glutamine/glutamate peaks resonated at 2.4 ppm nearby the N - acetyl - aspartate (NAA) peaks at 2 ppm as well as at 3.8 ppm between the inositol peak at 3.56 ppm and secondary creatinine peak at 3.9 ppm. There were peaks between 0.9 to 1.4 ppm on TE 35 ms suggesting lactate/lipid elevations. There was a negative doublet (reversal) at 1.3 ppm on TE 135 ms that was separating Lac peaks from lipid peaks. Lac/lipid elevation shows anaerobic glycolysis and cell necrosis. NAA peaks were normal, but choline (Cho) elevation is noted at 3.2 ppm with increased Cho/NAA and Cho/Cr ratios. Increased Cho suggests membrane turnover or cell proliferation. The patient has no hepatic or renal disorders biochemically on blood panel. Cerebrospinal fluid analysis was only positive for protein elevation. Metabolic panel was normal. There were no blood biochemical changes suggesting urea-cycle defects. Blood ammoniac level was measured high only once (174 µg/dL) (normal values range from 0 to 86 µg/dL). It was accepted as insignificant. On brain MRI, FLAIR and T2 signal increases with diffusion restriction on DWI and corresponding ADC maps suggested cytotoxic edema. Symmetric cytotoxic edema in the brain were suggestive of mitochondrial disease but external capsule involvement was atypical for mitochondrial disease. Lesion locations were typical of osmotic myelinolysis, but cytotoxic edema was against it. In osmotic myelinolysis, vasogenic edema was present and there was no associated diffusion restriction. Because of high fever and specific involvements such as external capsule and involvements of hippocampi, brainstem tracts and cerebellar cortices prompted us to think of heat stroke. The possibility of encephalitis was very low by virtue of bilateral symmetrical involvement. Mitochondrial disease and heat stroke were suspected. Mitochondrial panels were found to be normal on the following days. Brain MRI performed 16 days after admission revealed volume loss in the external capsule, thalamus, brainstem and cerebellar cortices with resolution of cytotoxic edema and regression of sinusitis and otomastoiditis at a great extent. Cytotoxic edema with hyperintensity on DWI and hypointensity on the corresponding ADC maps were all resolved. Mean ADC measurements from same places in the external capsule (1633 × 10-6 mm2/s), thalamus (801 × 10-6 mm2/s), brain stem tract (857 × 10-6 mm2/s) and cerebellum (773 × 10-6 mm2/s) on control MRI showed increased diffusion compared to the previous measurements. Edema and hyperintense signal changes were greatly decreased on FLAIR and T2 weighted images. On follow-up spectroscopy there were no glutamate/glutamine peaks but increased Cho peaks and Lac peak were still visible (Figure 2). For treatment of the patient, at first, fever was lowered by antipyretics. Antibiotics (ceftriaxon and clindamycin) were given for pansinusitis and otomastoiditis. The left ear was also drained via myringotomy. Acyclovir was also given for suspicion of viral encephalitis at the beginning. Two weeks later, the patient was discharged with clinical well being.
A 4 - year - old boy was admitted to our emergency clinic suffering from unconsciousness, vomiting and fever. On admission MRI, axial T2 (A, B, C, D) and FLAIR (E, F) images show bilateral symmetric hyperintense edematous changes in thalami and external capsules on A, Superior cerebellar peduncles (thin long arrows) and decussation of superior cerebellar peduncles (short arrows) on B and E, Hippocampi on B, C and E, Hypothalami (thick arrow on E), along tegmental tracts (long thin arrows on C, D and F), corticofugal fibers (black arrow on D) and lemniscal pathways (short arrows on C, D, F), and in the cerebellum on D, and F. The nodule (long thick arrow on F) of cerebellar vermis are seen more hyperintense compared to the rest of the cerebellum. There is associated diffusion restriction on diffusion weighted imaging (DWI) (G, H, and I) and corresponding apparent diffusion coefficient (ADC) maps (J, K, and L) in the external capsule, brain stem tracts and cerebellum. Mean ADC measurements from the external capsule (478 × 10 -6 mm2/s), thalamus (638 × 10-6 mm2/s), affected brain stem tract (555 × 10-6 mm2/s) and cerebellum (358 × 10-6 mm2/s) are shown on ADC maps (J, K, L). M - N, On long echo time (TE 135 ms), MR spectroscopy (M) shows elevated choline peak while short TE (TE 35 ms) (N) shows glutamine/glutamate peaks at 2.4 ppm and 3.8 ppm. Lipid - lactate peaks are seen on short echo (N) with doublet reversal on long echo (M). Cho: Choline, Glu/Gln: glutamate/glutamine, Lac: Lactate, Lac - Lip: Lactate - lipid, MI: myo - inositol.
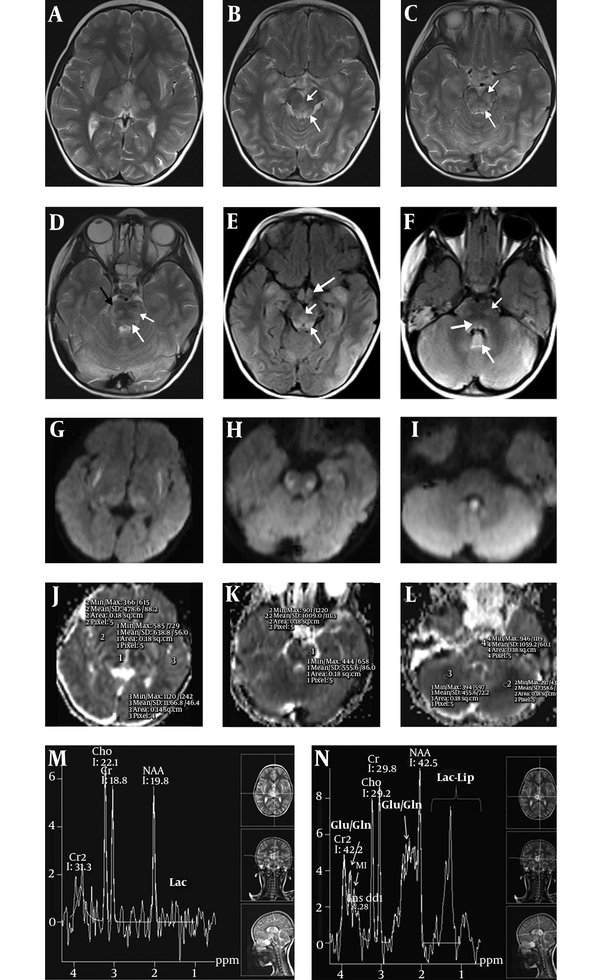
Control MRI performed 16 days later (A - E) shows that resolution of diffusion restriction on axial diffusion weighted imaging (DWI) (A) and decrease in the edematous hyperintense signal changes on axial T2W (B, C) images in the external capsules, thalami and brain stem. On long echo time (TE 135 ms) (D) and short TE (TE 35 ms) (E), MR spectroscopy views show elevations of choline, lactate and lipid peaks and resolution of glutamine/glutamate peaks. Lac: Lactate, Lac - Lip: Lactate - lipid, MI: myo - inositol.
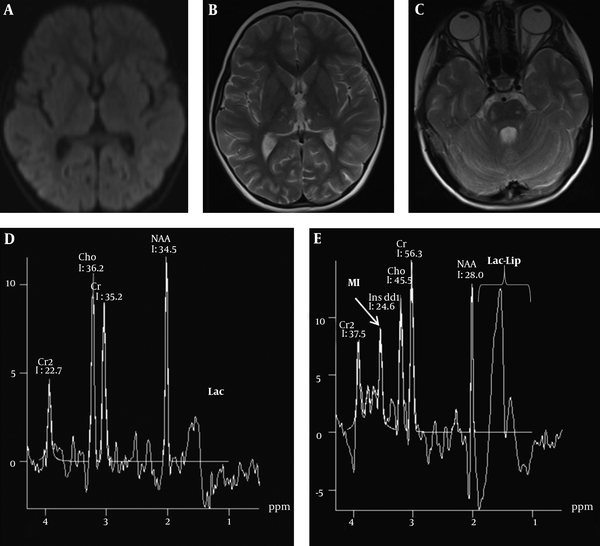
3. Discussion
Heat stroke is a medical emergency. It is described as a body temperature of > 40 °C that concludes changed mental situation and multiorgan dysfunction. Although the body temperature passes over a critical temperature at the time of collapse, in many cases lower temperatures are listed because the first reading may be delayed, applied by untrained personnel or evaluated incorrectly. The diagnosis of heat stroke should be thought of unless another cause is obvious (4). Heat - stroke can induce various different responses within the body that lead to neurologic dysfunction, such as decreased cerebral perfusion and aberrations in coagulations (1). At first, during hyperthermia, peripheral vasodilatation prevails to simplify heat loss through the skin. A compensatory vasoconstriction of the splanchnic and renal vasculature happens to avoid functional hypovolemia and causes symptoms of nausea, vomiting, and diarrhea. Combined with a failure of splanchnic vasoconstriction and decreased mean arterial pressure, cerebral blood flow decreases. This results in cerebral ischemia. During splanchnic vasoconstriction, ischemia increases bowel permeability. Gut bacteria produce endotoxin that infiltrate the bloodstream due to the increased gut permeability began by bowel ischemia. It is suggested that this endotoxin release results in an extreme inflammatory acute - phase response similar to sepsis. This extreme inflammatory response leads to a sepsis - like syndrome with multiorgan failure and change in hemostasis. If heat stress continues, however, the compensatory vasoconstriction will finally fail, further increasing the body temperature. Excessive production of cytokines can disrupt the blood brain barrier that results in vasogenic edema. Cerebrovascular congestion and cerebral edema happen with the hyperthermia, causing an increase in intracranial pressure. An aberration in coagulation is caused by the reduction in protein C, protein S, and antithrombin III, as well as changes in vascular endothelium, creating a pattern like sepsis and disseminated intravascular coagulation. This can cause small infarctions and hemorrhages within the brain, resulting in neurologic dysfunction. All these physiologic processes happen concomitantly, causing the common result of neuronal dysfunction. The central nervous system dysfunction results in delirium, convulsion or coma (1, 2, 4, 8, 9, 16, 20). Rhabdomyolysis and multiorgan failure are other serious complications of heat stroke (6).
Damage to the brain caused by heat stroke is multifactorial. Heat itself is directly cytotoxic to the cells. Circulatory insufficiency can cause hypoxic ischemic findings. Excessive production of cytokines can disrupt the blood brain barrier that results in vasogenic edema. Microthrombosis due to disseminated intravascular coagulation can result in small vessel ischemic damage. Metabolic derangement can result in osmotic myelinolysis (2, 6, 8).
Although the pathophysiologic mechanism of neuronal harm is clearly understood and known to occur in heat stroke, MR imaging of this situation is rare (1). In the literature, there are various reports on brain involvement in patients with heat stroke. The mostly reported findings were T2/FLAIR and DWI hyperintensity, representing either vasogenic or cytotoxic edema depending on the their appearance on ADC maps, in the cerebellum, thalamus, external capsule, cerebellar dentate nucleus, hippocampus, splenium of corpus callosum, gray matter of frontal, parietal and occipital cortex at vascular boundary zones and brainstem (Table 1) (1, 3-18). Involvement of basal ganglia was reported very rarely (1, 3, 12). Small millimetric foci were reported in the supratentorial white matter suggesting small vessel disease secondary to microthrombosis. Involvement of vascular boundary zones suggest circulatory disturbance in heat stroke. Brain MRI findings are usually bilateral and symmetric, except for a few cases (4, 8, 12). Cerebellar, mesencephalon and thalamic involvement are common and theorized to occur secondary to deafferentation of tracts exiting from the cerebellum. Dentarubral and dentathalamic tract are affected following cerebellar injury. This is why signal abnormalities are common in mesencephalon especially in superior cerebellar peduncles and decussation of superior cerebellar peduncles (central tegmental region of midbrain) and intralaminar and ventrolateral thalamic nuclei where tracts from the cerebellum project into (5, 16). Paraventricular thalamic involvement was also reported (1). Pontine involvement was reported and proposed to occur secondary to osmotic myelinolysis that is seen in heat stroke by electrolyte imbalance (1). Hippocampal involvement is probably the result of circulatory imbalance and hypoxic ischemic event. Midline cerebellum, especially the vestibulocerebellar region was proposed to be more affected by heat stroke upon the downbeat nystagmus, which was the sole clinical finding of cases with heat stroke (14, 19).
In our case, cytotoxic edema was noted in cerebellar cortices, along the corticofugal fibers, lemniscal pathways and tegmental tracts of the brain stem, in the external capsules, thalami, hippothalami, and hippocampi with acute pansinusitis and bilataral granulomatous otitis. Cytotoxic edema occurs when the cells are injured and swollen. Swollen cells hinder movements of protons in extracellular spaces, resulting in diffusion restriction. Cytotoxic edema due to diffusion restriction appears hyperintense on DWI and hypointensite on the corresponding ADC maps. Brain signal changes were bilateral, symmetric and nonenhancing. On MR spectroscopy, glutamine/glutamate peaks were present at 2.4 ppm and at 3.8 ppm. We evaluated peak signals visually. This is our limitation, as we did not perform any analysis such as curve - fitting method for quantification of metabolite peaks. In differential diagnosis, symmetrical involvement of brain stem, thalami and external capsule lead us to think of mitochondrial diseases and osmotic myelinosis at first. There is no history of hyponatremia nor recent fluid overload to suggest osmotic myelinolysis. Moreover, cytotoxic edema is atypical for osmotic myelinolysis. On the other hand, McLaughlin et al. and Sudhakar et al. suggest that heat stroke itself can cause serum electrolyte imbalance and osmotic myelinolysis (1, 4). Therefore, in our case, we cannot exclude the contribution of osmotic myelinolysis into brain MRI findings. We could not exclude the possibility of mitochondrial disease until the negative test results for mitochondrial disease arrived although external capsule involvement is unusual for mitochondrial diseases. Because of external capsules and cerebellar involvement, lead toxicity was questioned (21). Blood lead level was normal and there were no radiodense lines (lead lines) at the metaphysis of the long bones to suggest any current and previous lead exposure respectively. Meanwhile, biochemical panel for metabolic diseases were all negative. Cerebellar, deep gray matter, midbrain involvements were also suggestive of hepatic encephalopathy and Wilson disease. Moreover, glutamine/glutamate peaks can be seen in hepatic encephalopathy. Neither hepatic encephalopathy nor Wilson disease was present in our case (1). Uvulae and nodule of cerebellar vermis were more severely affected compared to the rest of the cerebellum in our case. Together with hypothalamic involvement, thiamine deficiency could be suspected, but there was no thiamine deficiency in our case. B12 deficiency, hypomagnesemia or drug abuse, which may affect vestibulocerebellum, were not present (14, 19). Involvement of external capsules and cerebellum as well as acute pansinusitis and bilateral granulamatous otomastoiditis guide us to think heat stroke as the cause of the brain MRI findings. Hippocampus and cerebellar cortical involvement could be part of hypoxic ischemic encephalopathy since heat stroke itself can cause hypoxic ischemic injury as well as the fact that cerebellum and hippocampus are vulnerable to that. However, white matter tract is resistant to hypoxic ischemic injury. We cannot exclude the contribution of hypoxic ischemic injury due to heat stroke, but all lesions in the brain cannot be explained by hypoxic ischemic injury alone.
On MR spectroscopy, glutamine/glutamate peaks both at 2, 4 and 3.8 ppm levels suggest excitotoxic brain injury in heat stroke. It could be the result of high body temperature or heat related hypoxic ischemic injury (1). Glutamate excitotoxicity during cerebral ischemia happens when a large amount of glutamate is released from the brain tissue into the extracellular space (20). There are a few studies in the literature proposing the possible role of excitotoxic injury in heat stroke (3, 18). In an animal study, Campos et al. showed that during the acute phase of stroke, pharmacological modulation of glutamate levels could neutralize the deleterious effects of hyperthermia as well as beneficial effects of hypothermia. They stated that glutamate excitotoxity is more critical than the effects of inflammatory response and metabolic rate on ischemic damage (22). MR spectroscopy findings in heat stroke have been reported in eight cases with low NAA/Cr value in all and elevated Lac peak in two of them after heat stroke compared to that of age matched controls (18). They found significant positive correlation between NAA/Cr ratios and Glasgow coma scale scores of patients. Excitotoxic injury could be another mechanism underlying heat stroke. This possibility may change the treatment approach since pharmacological modulation of glutamate levels in the brain through systemic injections may neutralize the deleterious effects of hyperthermia to the brain.
Excitotoxicity is extreme glutamatergic activation of postsynaptic receptors which causes cell injury and cell death. Glutamate is a main excitatory amino acid neurotransmitter in the central nervous system that mediates fast synaptic transmission and rules synaptic plasticity, learning and memory and other cognitive functions. However, large amounts of it can make severe damage if it accidentally leaks out of the brain tissue. In physiological situations, the presence of glutamate in the synapse is regulated by ATP - dependent glutamate transporters in neurons and glial cells. In pathologic terms, the role of transporters is impaired, which results in glutamate accumulation in the synaptic cleft and in turn excessive activation of postsynaptic glutamate receptors with next massive Ca2+ influx. Impaired calcium homeostasis makes nitric oxide synthesis, mitochondrial dysfunction, free radical production and programmed cell death which result in progressive neurodegeneration (20, 22, 23).
In conclusion, heat stroke is critical illness caused by hyperthermia, and symmetric involvement of the cerebellum, midbrain, external capsule, thalamus and hippocampus are typical. Low NAA/Cr ratios and lactate elevation was reported after heat stroke. There are many theories behind the pathophysiology of brain imaging findings of heat stroke: heat itself, circulatory insufficiency, impairment of the blood brain barrier by excessive production of cytokines, microthrombosis and osmotic myelinolysis. MR spectroscopy findings of our case showed us glutamine/glutamate peaks at 2.4 ppm and 3.8 ppm, suggesting excitotoxic injury. Excitotoxic injury could be another mechanism underlying heat stroke.
References
-
1.
McLaughlin CT, Kane AG, Auber AE. MR imaging of heat stroke: external capsule and thalamic T1 shortening and cerebellar injury. AJNR Am J Neuroradiol. 2003;24(7):1372-5. [PubMed ID: 12917130].
-
2.
Leon LR, Helwig BG. Heat stroke: role of the systemic inflammatory response. J Appl Physiol (1985). 2010;109(6):1980-8. [PubMed ID: 20522730]. https://doi.org/10.1152/japplphysiol.00301.2010.
-
3.
Muccio CF, De Blasio E, Venditto M, Esposito G, Tassi R, Cerase A. Heat-stroke in an epileptic patient treated by topiramate: follow-up by magnetic resonance imaging including diffusion-weighted imaging with apparent diffusion coefficient measure. Clin Neurol Neurosurg. 2013;115(8):1558-60. [PubMed ID: 23411048]. https://doi.org/10.1016/j.clineuro.2013.01.005.
-
4.
Sudhakar PJ, Al-Hashimi H. Bilateral hippocampal hyperintensities: a new finding in MR imaging of heat stroke. Pediatr Radiol. 2007;37(12):1289-91. [PubMed ID: 17899057]. https://doi.org/10.1007/s00247-007-0612-0.
-
5.
Fushimi Y, Taki H, Kawai H, Togashi K. Abnormal hyperintensity in cerebellar efferent pathways on diffusion-weighted imaging in a patient with heat stroke. Clin Radiol. 2012;67(4):389-92. [PubMed ID: 22119294]. https://doi.org/10.1016/j.crad.2011.09.009.
-
6.
Ookura R, Shiro Y, Takai T, Okamoto M, Ogata M. Diffusion-weighted magnetic resonance imaging of a severe heat stroke patient complicated with severe cerebellar ataxia. Intern Med. 2009;48(12):1105-8. [PubMed ID: 19525609].
-
7.
Lee JS, Choi JC, Kang SY, Kang JH, Park JK. Heat stroke: increased signal intensity in the bilateral cerebellar dentate nuclei and splenium on diffusion-weighted MR imaging. AJNR Am J Neuroradiol. 2009;30(4). E58. [PubMed ID: 19179428]. https://doi.org/10.3174/ajnr.A1432.
-
8.
Fuse A, Yamashiro K, Oji Y, Furuya T, Noda K, Hattori N, et al. Reversible focal cerebral cortical lesions in a patient with heat stroke. Intern Med. 2013;52(3):377-80. [PubMed ID: 23370749].
-
9.
Mahajan S, Schucany WG. Symmetric bilateral caudate, hippocampal, cerebellar, and subcortical white matter MRI abnormalities in an adult patient with heat stroke. Proc (Bayl Univ Med Cent). 2008;21(4):433-6. [PubMed ID: 18982090].
-
10.
Ray S, Sharma S, Maheshwari A, Aneja S, Kumar A. Heat stroke in an infant with hypohidrotic ectodermal dysplasia: brain magnetic resonance imaging findings. J Child Neurol. 2013;28(4):538-40. [PubMed ID: 23358631]. https://doi.org/10.1177/0883073812474097.
-
11.
Fujioka Y, Yasui K, Hasegawa Y, Takahashi A, Sobue G. [An acute severe heat stroke patient showing abnormal diffuse high intensity of the cerebellar cortex in diffusion weighted image: a case report]. Rinsho Shinkeigaku. 2009;49(10):634-40. [PubMed ID: 19999144].
-
12.
Biary N, Madkour MM, Sharif H. Post-heatstroke parkinsonism and cerebellar dysfunction. Clin Neurol Neurosurg. 1995;97(1):55-7. [PubMed ID: 7788975].
-
13.
McNamee T, Forsythe S, Wollmann R, Ndukwu IM. Central pontine myelinolysis in a patient with classic heat stroke. Arch Neurol. 1997;54(8):935-6. [PubMed ID: 9267966].
-
14.
Deleu D, El Siddig A, Kamran S, Kamha AA, Al Omary IY, Zalabany HA. Downbeat nystagmus following classical heat stroke. Clin Neurol Neurosurg. 2005;108(1):102-4. [PubMed ID: 16311159]. https://doi.org/10.1016/j.clineuro.2004.12.009.
-
15.
Albukrek D, Bakon M, Moran DS, Faibel M, Epstein Y. Heat-stroke-induced cerebellar atrophy: clinical course, CT and MRI findings. Neuroradiology. 1997;39(3):195-7. [PubMed ID: 9106293].
-
16.
Bazille C, Megarbane B, Bensimhon D, Lavergne-Slove A, Baglin AC, Loirat P, et al. Brain damage after heat stroke. J Neuropathol Exp Neurol. 2005;64(11):970-5. [PubMed ID: 16254491].
-
17.
Akaboshi S, Miyashita A. [A case of heat stroke with cortical laminar necrosis on vascular boundary zones]. No To Hattatsu. 1996;28(5):434-7. [PubMed ID: 8831247].
-
18.
Li J, Zhang XY, Wang B, Zou ZM, Li HF, Wang PY, et al. Multivoxel proton magnetic resonance spectroscopy in heat stroke. Clin Radiol. 2015;70(1):37-41. [PubMed ID: 25443777]. https://doi.org/10.1016/j.crad.2014.09.004.
-
19.
Van Stavern GP, Biousse V, Newman NJ, Leingang JC. Downbeat nystagmus from heat stroke. J Neurol Neurosurg Psychiatry. 2000;69(3):403-4. [PubMed ID: 10991650].
-
20.
Campos F, Perez-Mato M, Agulla J, Blanco M, Barral D, Almeida A, et al. Glutamate excitoxicity is the key molecular mechanism which is influenced by body temperature during the acute phase of brain stroke. PLoS One. 2012;7(8). e44191. [PubMed ID: 22952923]. https://doi.org/10.1371/journal.pone.0044191.
-
21.
Barkovich AJ, Patay Z. Metabolic, toxic, and inflammatory brain disorders. In: Barkovich AJ, Raybaud C, editors. Pediatric Neuroimaging. 5th ed. Philadelphia, PA: Lippincott Williams and Wilkins; 2012. 141 p.
-
22.
Mehta A, Prabhakar M, Kumar P, Deshmukh R, Sharma PL. Excitotoxicity: bridge to various triggers in neurodegenerative disorders. Eur J Pharmacol. 2013;698(1-3):6-18. [PubMed ID: 23123057]. https://doi.org/10.1016/j.ejphar.2012.10.032.
-
23.
Wang Y, Qin ZH. Molecular and cellular mechanisms of excitotoxic neuronal death. Apoptosis. 2010;15(11):1382-402. [PubMed ID: 20213199]. https://doi.org/10.1007/s10495-010-0481-0.