Abstract
Background:
Atherosclerosis is the main cause of cardiovascular disease, which has no effective preventive treatments. Lectin-like Oxidized LDL Receptor-1 (LOX-1) is a major receptor of ox-LDL in endothelial cells and plays an important role in atherosclerosis.Objectives:
This research aimed to determine the role of LOX-1 protein vaccination in inhibiting atherosclerosis plaque progression through measurement of foam cells number and aorta wall thickness and reducing inflammation through Nuclear Factor–kappa B (NF-κB) activation and endothelial nitric oxide synthase (eNOS) expression.Methods:
This experimental study was conducted on seven groups of 28 male Wistar rats aged 6 to 8 weeks, including negative control group (normal diet rats), positive control group (atherogenic diet rats without vaccination), and 4 other experimental groups receiving atherogenic diet and LOX-1 vaccine containing 1, 10, 100, and 1000 ng LOX-1. Vaccination was performed on days 0, 21, and 35. The vaccines were also supplemented by aluminum hydroxide (alum) as an adjuvant. Finally, the 7th group only received atherogenic diet and alum. On the 57th day, the rats were euthanatized and their serum anti-LOX-1 IgG level, NF-κB activation, eNOS expressions, foam cells number, and aorta wall’s thickness were measured. After all, the data were analyzed using the SPSS statistical software, version 20 and P < 0.05 was considered to be statistically significant.Results:
The results indicated that the vaccine was unable to raise the anti-LOX-1 IgG level significantly (P = 0.010). However, it was able to inhibit foam cells formation and aorta wall’s thickness significantly (P = 0.001). Furthermore, LOX-1 vaccination could significantly inhibit the activation of NF-κB (P = 0.001) and increase eNOS expression (P = 0.001).Conclusions:
LOX-1 vaccine could potentially inhibit atherosclerosis by decreasing NF-κB activation, increasing eNOS expression, and inhibiting atherosclerosis plaques progression. Although the vaccine did not induce humoral immune response, it successfully inhibited the progression of atherosclerosis. Thus, it was predicted to work through other mechanisms, such as inducing cellular immune response.Keywords
1. Background
Cardiovascular disease, mainly caused by atherosclerosis, can inflict the greatest death in both developed and developing countries (1). Atherosclerosis is a chronic inflammation response towards cholesterol deposition on the arteries’ walls. Additionally, atherosclerosis plaque forming process is significantly affected by low-density lipoprotein (LDL) oxidation. Oxidized LDL (ox-LDL) uptake in macrophages leads to foam cell formation. The formed foam cells in turn result in secretion of pro-inflammation cytokines, such as tumor necrosis factor α (TNFα) and Interleukin-1 (IL-1) (2-4).
Lectin-like oxidized LDL receptor-1 (LOX-1) is one of the ox-LDL scavenger receptors in endothelial cells, which can contribute to atherogenesis through mediation of ox-LDL uptake. Moreover, ox-LDL causes endothelial cells dysfunction followed by an atherogenesis early phase inside the tunica intima (5-7). So far, atherosclerosis therapy has focused on anti-inflammation and anti-hyperlipidemia, which only inhibit atherosclerosis plaque progression, but not formation. Therefore, studies have to be conducted on atherosclerosis inhibition involving immune system as a promising preventive target. Peptide-based immunization is a choice to induce an immune response against atherosclerosis.
Recent studies have developed several vaccines against atherosclerosis, namely cholesteryl ester transfer protein (CETP) (8-11), IL-1 (12), and vascular endothelial growth factor receptor 2 (VEGFR2) (13), to induce protective immune response against atherosclerosis. Based on this approach, the present research aims to examine the potency of LOX-1 protein as an anti-atherosclerosis vaccine candidate. Although the role of LOX-1 in atherosclerosis is well known (2, 4-6), our study is the first to investigate the potency of LOX-1 as an anti-atherosclerosis vaccine candidate.
2. Objectives
Our study aims at investigation of the effect of LOX-1 protein on inhibiting NF-κB activity, eNOS expression, foam cells formation, and aorta wall’s thickness in atherogenic-diet Wistar rats (Rattus norvegicus).
3. Methods
3.1. Research Objects and Samples
After obtaining ethical approval from the ethics committee of faculty of medicine, Brawijaya university (No. 169/EC/KEPK-S1-FARM/03/2014), 28 healthy male Wistar-strain rats (Rattus novergicus) aging 6 - 8 weeks and weighing 120 - 160 g were enrolled into this study. The rats were divided into 7 groups, including a negative control group (normal diet with no vaccination), a positive control group (atherogenic diet with no vaccination), P1, P2, P3, P4 (atherogenic diet with 1, 10, 100, 1000 ng LOX-1 protein vaccination combined with alum as the adjuvant), and P5 (atherogenic diet with alum vaccination). The samples were selected using simple random sampling.
3.2. Atherosclerosis Induction
Atherosclerosis was induced by giving atherogenic diet every day for 56 days (14, 15). The normal and atherogenic diets used in this research were a modification of the American Institute of Nutrition-93M (AIN-93M) diet (16, 17), as shown in Table 1.
Normal and Atherogenic Diet Compositions
Substrate | Weight (g/kg) | |
---|---|---|
Normal Diet | Atherogenic Diet | |
Corn starch | 620 | 210 |
Sucrose | 100 | 175 |
Soybean oil | 40 | 50 |
Gelatin | 65 | 50 |
Casein | 80 | 128 |
CMC | 50 | 51 |
Mineral and vitamin | 5 pieces | 10 pieces |
Fat | - | 105 |
Coconut oil | - | 105 |
Cholic acid | - | 3 |
Cholesterol | - | 10 |
Propylthiouracil | - | 2 |
3.3. LOX-1 Vaccination
The vaccines were prepared in a sterile condition by mixing 1, 10, 100, or 1000 ng of homodimer LOX-1 protein (Rat OLR1 Fc Tag) (Sino biological). Then, LOX-1 protein was dissolved in 100µL Phosphate Buffer Saline (PBS) and 100 μL alum as the adjuvant. Alum was provided by Professor Dr. Aulanni’am, DVM., DES, Faculty of Veterinary Medicine, Brawijaya University. The solution was prepared under sterile conditions. This mixture was subcutaneously injected on days 0, 21, and 35 (18-21).
3.4. Measurement of Anti-LOX-1
The rats were sacrificed using chloroform on the 57th day. Then, the aortas were isolated and blood samples were taken. The samples were centrifuged at 3000 rpm for 20 minutes to obtain the sera. After all, anti-LOX-1 IgG level was measured using ELISA method (Shanghai Crystal day Biotech Co, LTD).
3.5. Measurement of NF-kB and eNOS Expressions
Aorta slides were prepared using frozen section/fries coupe at -20°C and were cut into 3 - 5 µm thickness sections. The prepared slides were stained after methanol fixation for 5 minutes followed by three times washing with PBS and H2O2 addition. The blocking process was performed using Fetal Bovine Serum (FBS) containing 0.25% Triton X-100 for 1 hour. Then, anti-NF-kB (Sino Biological) and anti-eNOS (Source BioScience) antibodies were added and the slides were incubated overnight. On the next day, the slides were washed with PBS and were added to the secondary antibody for 1 hour. The streptavidin horse radish peroxidase (SA-HRP) was also added after slide washing followed by another incubation for 40 minutes. After washing, Diaminobenzidine (DAB) was added and the slides were incubated for 30 minutes. Counterstain was then performed with Mayer’s hematoxylin for 10 minutes. Thereafter, the slides were washed, dried, and covered using cover glasses. At each stage, the washing process was performed using PBS for three times. Finally, measurement of the cells with activation of NF-kB and eNOS was carried out using an optical microscope at 1000 × magnification.
3.6. Measurement of Foam Cells and Aorta Wall’s Thickness
Histopathology slides for measurement of foam cells and aorta wall’s thickness were obtained using the paraffin method. Then, the slides were stained using Hematoxylin-Eosin. Afterwards, the slides were examined at 400 × magnification for measurement of aorta wall’s thickness and at 1000 × magnification for computation of foam cells number via olympus photo slide BX51 Microscope.
3.7. Data Analysis
The data were analyzed using the SPSS statistical software, version 20 and P < 0.05 was considered to be statistically significant. After assessing the normality and homogeneity of the data, they were analyzed using one-way ANOVA and Tukey’s post-hoc test.
4. Results
4.1. Analysis of Foam Cells Formation and Aorta Wall’s Thickness
Generally, foam cells formation and aorta wall’s thickness are the major characteristics of atherosclerosis. Therefore, this study aimed to determine the foam cells number and aorta wall’s thickness using specific staining for each group. The mean of foam cells number and aorta wall’s thickness in each group have been presented in Table 2. The representative examples of aorta walls have also been depicted in Figure 1. The results revealed a significant difference between the non-treated group and all the vaccinated groups with atherogenic diet regarding the number of foam cells. Nonetheless, the treatment group that received 1000 ng LOX-1+alum was similar to the normal diet group in this regard (P = 0.055). This indicated that LOX-1 vaccination inhibited the formation of foam cells. A similar trend was also detected with respect to aorta wall’s thickness. Accordingly, LOX-1 vaccination significantly inhibited aorta wall’s thickening. In other words, all the treated groups showed thinner aorta walls compared to the non-treated group.
Foam Cells Number and Aorta Wall’s Thickness [Mean (SD)]
Groups | Foam Cells Number | P Value (vs. Kp) | Aorta Wall’s Thickness (µm) | P Value (vs. Kp) |
---|---|---|---|---|
Normal diet without vaccination (Kn) | 0.78 (0.30) | 0.001 | 1.83 (0.10) | 0.001 |
Atherogenic diet without vaccination (Kp) | 7.15 (0.13) | 3.55 (1.18) | ||
Atherogenic diet with 1 ng LOX-1 vaccination (P1) | 3.25 (0.72) | 0.001 | 1.81 (0.04) | 0.009 |
Atherogenic diet with 10 ng LOX-1 vaccination (P2) | 2.73 (0.56) | 0.001 | 1.77 (0.61) | 0.007 |
Atherogenic diet with 100 ng LOX-1 vaccination (P3) | 2.73 (0.87) | 0.001 | 1.63 (90.17) | 0.003 |
Atherogenic diet with 1000 ng LOX-1 vaccination (P4) | 2.25 (0.37) | 0.001 | 1.60 (0.19) | 0.002 |
Atherogenic diet with alum vaccination (P5) | 3.23 (0.42) | 0.001 | 1.84 (0.21) | 0.017 |
Aorta Wall’s Thickness in Various Groups
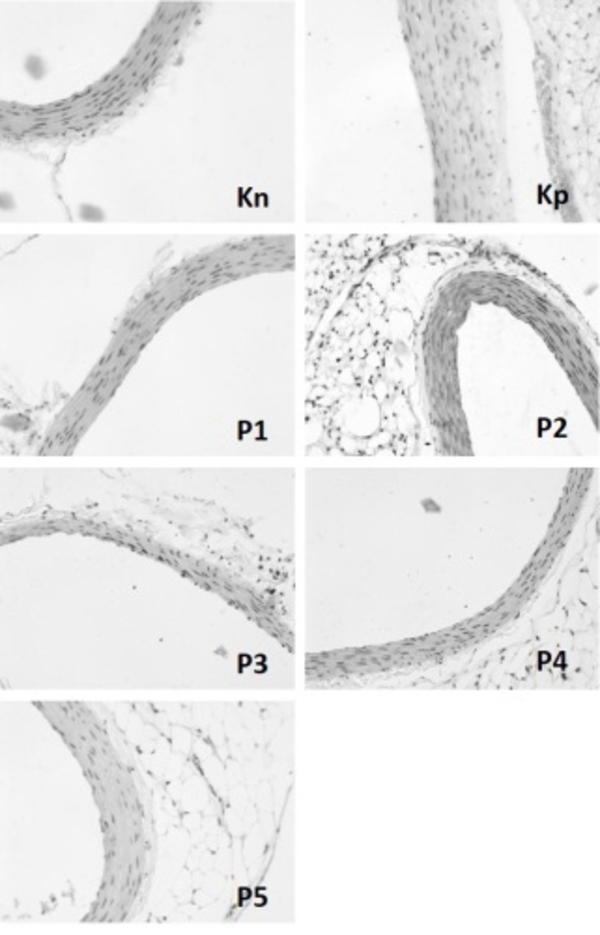
4.2. LOX-1 Administration Inhibited NF-κB Activation and Stabilized eNOS Expression in Endothelial Cells
In general, NF-κB activation and reduction of eNOS expression show endothelial cells dysfunction (22). The current study also assessed NF-κB activation and eNOS expression in aortic sections from different groups of rats. The results have been presented in Figure 2. Accordingly, the NF-κB activation significantly reduced in the vaccinated groups compared to the non-vaccinated group. On the other hand, the LOX-1 vaccination normalized the expression of eNOS. Interestingly, the group only treated by alum showed a reduction in eNOS expression compared to the LOX-1 vaccination groups.
Nf-kB Activation Percentage (A) and eNOS Expression Percentage (B)
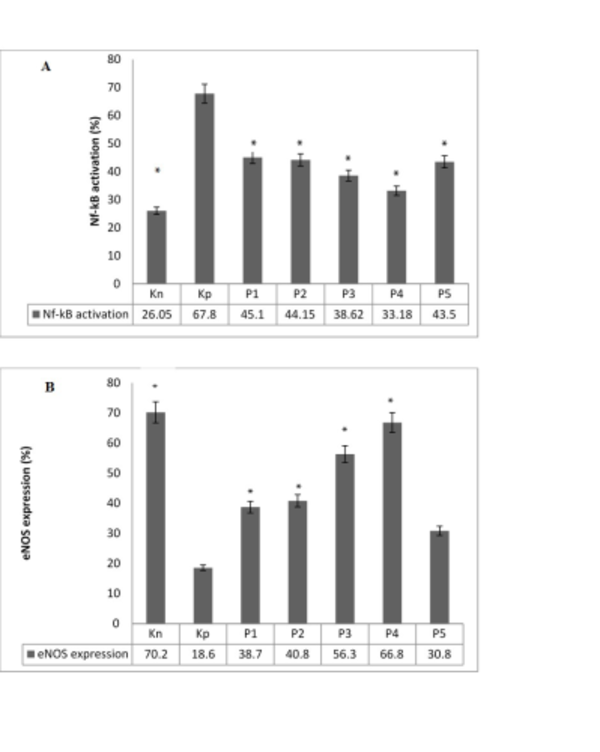
4.3. The Effect of LOX-1 Administration on Anti-LOX-1 Ig-G Level
LOX-1 administration was expected to raise IgG anti-LOX-1 production that can inhibit the interaction between ox-LDL and LOX-1. Although LOX-1 is an endogenous protein, LOX-1 administration combined with the alum as the adjuvant was presumed to increase the anti-LOX-1 level. However, administration of LOX-1 in various concentrations (1, 10, 100, and 1000 ng) in combination with alum failed to raise the serum level of IgG anti-LOX-1 (Figure 3).
Anti-LOX-1 Ig G Concentration in Various Groups
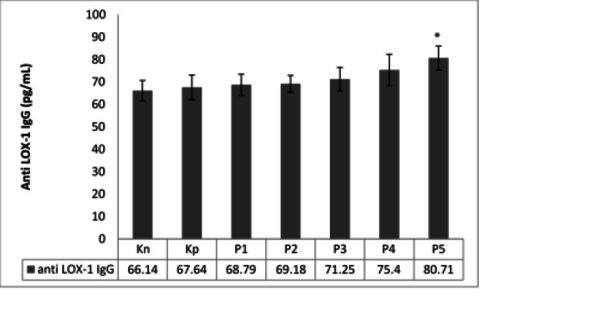
5. Discussion
Atherogenic diet promotes atherosclerosis by increasing LDL, changing it into its oxidized form, ox-LDL. ox-LDL uptake, in turn, leads to activation of NF-κB (6, 23). A previous study showed that NF-κB activation increased as an inflammatory response that stimulated endothelial dysfunction in an atherosclerotic rat model (23). In the present study also, vaccination using LOX-1 protein significantly prevented endothelial dysfunction, proven by the inhibition of NF-κB activation and stabilization of eNOS expression.
Similar results were also obtained in the previous researches. For instance, in vivo given anti-LOX-1 antibody restored. NO-mediated coronary arteriolar dilatation in ApoE-/- mice (24). Similar to the in vivo model, an in vitro experiment showed that LOX-1 and Membrane Type 1 Matrix Metalloproteinase (MT-1-MMP) complex contributed to downregulation of reactive oxygen species (ROS) formation and eNOS expression (25). Moreover, the studies conducted in patients with stable coronary artery disease (CAD) or acute coronary syndrome (ACS) indicated that high-density lipoprotein (HDL) could trigger LOX-1 expression and consequently increased endothelial Protein Kinase C β2 (PKCβ2) activation, which led to eNOS activation (26). In short, LOX-1 played a central role in regulating. NO-mediated vascular reactivity.Activation of NF-κB induced increase of vascular endothelial adhesion molecule (VCAM-1), intracellular endothelial adhesion molecule (ICAM-1), and monocyte chemoattractant protein (MCP-1) expressions (25, 26). Furthermore, increase of the adhesion molecule led to attraction of monocytes followed by lipid accumulation that resulted in foam cells formation. Indeed, inhibition of NF-κB activation repressed the formation of foam cells (27, 28). Our findings demonstrated that inhibition of NF-κB activation successfully blocked the formation of foam cells. Decrease in the number of foam cells, in turn, affected the reduction of aorta wall’s thickness in the vaccinated rats. Hence, vaccination of LOX-1 protein protected atherosclerosis by inhibiting foam cells formation and aorta wall’s thickening.
Although the importance of LOX-1 protein in development of atherosclerosis has been studied extensively (3, 6, 26), our study was the first to demonstrate the potential inhibition of atherosclerosis through LOX-1 vaccination. In this study, LOX-1 protein combined with alum was used to induce anti-LOX-1 IgG production. Besides, the LOX-1 vaccination was given in 1 - 1000 ng dosages, and alum was used as the adjuvant. Generally, alum acts as a delivery system to promote antigen uptake into immune cells. It is one of the most useful adjuvants with multiple pathways to stimulate the immune system. Moreover, alum plays a role as a depot system by allowing retention of antigen in the injection site. In this way, the antigen is released slowly and the recruited antigen presenting cells can interact extensively (29-31). Alum also has anti-atherogenic properties. Therefore, this adjuvant may be suitable for developing anti-atherosclerosis vaccines. However, the hypercholesterolemia condition may shift the immune response induced by alum towards activation of Tregs. Moreover, individuals with hypercholesterolemia may develop tolerance against the antigen given through vaccination (20). Although the antibody against LOX-1 was not produced, the results indicated that our vaccination inhibited atherosclerosis progression. In the same line, apo-B peptide vaccine inhibited atherosclerosis without activating apo-B specific antibody, suggesting that the atheroprotection effect was modulated through cellular immune response (18). The CD8+T cell has been shown to play a role in atheroprotection, as well (32-34). Nevertheless, the role of IgG in atherosclerosis is still unclear. IgG had an anti-atherosclerosis function through its neutralizing action. However, it induced activation of macrophages, thereby promoting atherogenesis (35). Another possibility is that LOX-1 acts as a soluble receptor for ox-LDL. Therefore, ox-LDL uptake is inhibited that leads to inhibition of atherosclerosis progression. Yet, the pathophysiological role of soluble LOX-1 remains unclear. Neither signaling nor scavenging activity of this soluble receptor has been identified (36).
5.1. Conclusion
LOX-1 vaccination significantly decreased the formation of foam cells and thickening of the aorta’s wall compared to the non-vaccinated atherogenic-diet Wistar rats. LOX-1 vaccination also inhibited the increase of NF-κB activity and stabilized the expression of eNOS in endothelial cells of atherogenic-diet Wistar rats. Thus, LOX-1 protein vaccination might have atheroprotection effects through modulation of cellular immune response. In addition, it might act as a soluble receptor inhibiting ox-LDL uptake in endothelial cells.
Acknowledgements
References
-
1.
Mozaffarian. Heart disease and stroke statistics-2015 update: A report from the american heart association (vol 131, pg e29, 2015). Circulation. 2015;131(24):535.
-
2.
Ding Z, Liu S, Wang X, Dai Y, Khaidakov M, Romeo F, et al. LOX-1, oxidant stress, mtDNA damage, autophagy, and immune response in atherosclerosis. Can J Physiol Pharmacol. 2014;92(7):524-30. [PubMed ID: 24959993]. https://doi.org/10.1139/cjpp-2013-0420.
-
3.
Mehta JL, Chen J, Hermonat PL, Romeo F, Novelli G. Lectin-like, oxidized low-density lipoprotein receptor-1 (LOX-1): a critical player in the development of atherosclerosis and related disorders. Cardiovasc Res. 2006;69(1):36-45. [PubMed ID: 16324688]. https://doi.org/10.1016/j.cardiores.2005.09.006.
-
4.
Yoshimoto R, Fujita Y, Kakino A, Iwamoto S, Takaya T, Sawamura T. The discovery of LOX-1, its ligands and clinical significance. Cardiovasc Drugs Ther. 2011;25(5):379-91. [PubMed ID: 21805404]. https://doi.org/10.1007/s10557-011-6324-6.
-
5.
Mango R, Predazzi IM, Romeo F, Novelli G. LOX-1/LOXIN: the yin/yang of atheroscleorosis. Cardiovasc Drugs Ther. 2011;25(5):489-94. [PubMed ID: 21904818]. https://doi.org/10.1007/s10557-011-6333-5.
-
6.
Pirillo A, Norata GD, Catapano AL. LOX-1, OxLDL, and atherosclerosis. Mediators Inflamm. 2013;2013:152786. [PubMed ID: 23935243]. https://doi.org/10.1155/2013/152786.
-
7.
Sawamura T, editor. LOX-1, a lectin-like oxidized LDL receptor identified form endothelial cells, in endothelial dysfunction. International Congress Series. 2004. Elsevier; p. 531-4.
-
8.
Gaofu Q, Jun L, Xin Y, Wentao L, Jie W, Xiuyun Z, et al. Vaccinating rabbits with a cholesteryl ester transfer protein (CETP) B-Cell epitope carried by heat shock protein-65 (HSP65) for inducing anti-CETP antibodies and reducing aortic lesions in vivo. J Cardiovasc Pharmacol. 2005;45(6):591-8. [PubMed ID: 15897787].
-
9.
Liaw YW, Lin CY, Lai YS, Yang TC, Wang CJ, Whang-Peng J, et al. A vaccine targeted at CETP alleviates high fat and high cholesterol diet-induced atherosclerosis and non-alcoholic steatohepatitis in rabbit. PLoS One. 2014;9(12). e111529. [PubMed ID: 25486007]. https://doi.org/10.1371/journal.pone.0111529.
-
10.
Mao D, Kai G, Gaofu Q, Zheng Z, Li Z, Jie W, et al. Intramuscular immunization with a DNA vaccine encoding a 26-amino acid CETP epitope displayed by HBc protein and containing CpG DNA inhibits atherosclerosis in a rabbit model of atherosclerosis. Vaccine. 2006;24(23):4942-50. [PubMed ID: 16697088]. https://doi.org/10.1016/j.vaccine.2006.03.082.
-
11.
Rittershaus CW, Miller DP, Thomas LJ, Picard MD, Honan CM, Emmett CD, et al. Vaccine-induced antibodies inhibit CETP activity in vivo and reduce aortic lesions in a rabbit model of atherosclerosis. Arterioscler Thromb Vasc Biol. 2000;20(9):2106-12. [PubMed ID: 10978256].
-
12.
Tissot AC, Spohn G, Jennings GT, Shamshiev A, Kurrer MO, Windak R, et al. A VLP-based vaccine against interleukin-1alpha protects mice from atherosclerosis. Eur J Immunol. 2013;43(3):716-22. [PubMed ID: 23254454]. https://doi.org/10.1002/eji.201242687.
-
13.
Hauer AD, van Puijvelde GH, Peterse N, de Vos P, van Weel V, van Wanrooij EJ, et al. Vaccination against VEGFR2 attenuates initiation and progression of atherosclerosis. Arterioscler Thromb Vasc Biol. 2007;27(9):2050-7. [PubMed ID: 17600223]. https://doi.org/10.1161/ATVBAHA.107.143743.
-
14.
El-Shatanovi G, Ashoush I, Ahmed EK, Ali SA. Antiatherogenic properties of vegetable juice rich in antioxidants in cholesterol-fed rats. Annal Agricultural Sci. 2012;57(2):167-73.
-
15.
Shirai K, Ishikawa Y, Nishide T, Sasaki N, Murano S, Sato A, et al. Effect of niceritrol on lipid metabolism of aorta in atherosclerotic rats. Tohoku J Exp Med. 1984;143(2):231-8.
-
16.
Handayani D, Chen J, Meyer BJ, Huang XF. Dietary Shiitake Mushroom (Lentinus edodes) Prevents Fat Deposition and Lowers Triglyceride in Rats Fed a High-Fat Diet. J Obes. 2011;2011:258051. [PubMed ID: 22028957]. https://doi.org/10.1155/2011/258051.
-
17.
Handayani D, Meyer BJ, Chen J, Tang P, Kwok PCL, Chan HK, et al. The comparison of the effect of oat and Shiitake mushroom powder to prevent body weight gain in rats fed high fat diet. Food Nutr Sci. 2012.
-
18.
Fredrikson GN, Bjorkbacka H, Soderberg I, Ljungcrantz I, Nilsson J. Treatment with apo B peptide vaccines inhibits atherosclerosis in human apo B-100 transgenic mice without inducing an increase in peptide-specific antibodies. J Intern Med. 2008;264(6):563-70. [PubMed ID: 18783480]. https://doi.org/10.1111/j.1365-2796.2008.01995.x.
-
19.
Pauwels R, Bazin H, Platteau B, Van der Straeten M. The influence of antigen dose on IgE production in different rat strains. Immunol. 1979;36(1):151.
-
20.
Wigren M, Bengtsson D, Duner P, Olofsson K, Bjorkbacka H, Bengtsson E, et al. Atheroprotective effects of Alum are associated with capture of oxidized LDL antigens and activation of regulatory T cells. Circ Res. 2009;104(12):62-70. [PubMed ID: 19478203]. https://doi.org/10.1161/CIRCRESAHA.109.196667.
-
21.
Wigren M, Kolbus D, Duner P, Ljungcrantz I, Soderberg I, Bjorkbacka H, et al. Evidence for a role of regulatory T cells in mediating the atheroprotective effect of apolipoprotein B peptide vaccine. J Intern Med. 2011;269(5):546-56. [PubMed ID: 21114693]. https://doi.org/10.1111/j.1365-2796.2010.02311.x.
-
22.
Sawamura T, Wakabayashi I, Okamura T. LOX-1 in atherosclerotic disease. Clin Chim Acta. 2015;440:157-63. [PubMed ID: 25463747]. https://doi.org/10.1016/j.cca.2014.11.016.
-
23.
Gareus R, Kotsaki E, Xanthoulea S, van der Made I, Gijbels MJ, Kardakaris R, et al. Endothelial cell-specific NF-kappaB inhibition protects mice from atherosclerosis. Cell Metab. 2008;8(5):372-83. [PubMed ID: 19046569]. https://doi.org/10.1016/j.cmet.2008.08.016.
-
24.
Xu X, Gao X, Potter BJ, Cao JM, Zhang C. Anti-LOX-1 rescues endothelial function in coronary arterioles in atherosclerotic ApoE knockout mice. Arterioscler Thromb Vasc Biol. 2007;27(4):871-7. [PubMed ID: 17272755]. https://doi.org/10.1161/01.ATV.0000259358.31234.37.
-
25.
Sugimoto K, Ishibashi T, Sawamura T, Inoue N, Kamioka M, Uekita H, et al. LOX-1-MT1-MMP axis is crucial for RhoA and Rac1 activation induced by oxidized low-density lipoprotein in endothelial cells. Cardiovasc Res. 2009;84(1):127-36. [PubMed ID: 19487339]. https://doi.org/10.1093/cvr/cvp177.
-
26.
Turcan S, Rohle D, Goenka A, Walsh LA, Fang F, Yilmaz E, et al. IDH1 mutation is sufficient to establish the glioma hypermethylator phenotype. Nature. 2012;483(7390):479-83.
-
27.
Libby P, Ridker PM, Hansson GK. Progress and challenges in translating the biology of atherosclerosis. Nature. 2011;473(7347):317-25. [PubMed ID: 21593864]. https://doi.org/10.1038/nature10146.
-
28.
Weber C, Noels H. Atherosclerosis: current pathogenesis and therapeutic options. Nat Med. 2011;17(11):1410-22. [PubMed ID: 22064431]. https://doi.org/10.1038/nm.2538.
-
29.
Brito LA, O'Hagan DT. Designing and building the next generation of improved vaccine adjuvants. J Control Release. 2014;190:563-79. [PubMed ID: 24998942]. https://doi.org/10.1016/j.jconrel.2014.06.027.
-
30.
De Gregorio E, Caproni E, Ulmer JB. Vaccine adjuvants: mode of action. Front Immunol. 2013;4:214. [PubMed ID: 23914187]. https://doi.org/10.3389/fimmu.2013.00214.
-
31.
Kool M, Fierens K, Lambrecht BN. Alum adjuvant: some of the tricks of the oldest adjuvant. J Med Microbiol. 2012;61(Pt 7):927-34. [PubMed ID: 22174375]. https://doi.org/10.1099/jmm.0.038943-0.
-
32.
Hauer AD, Habets KL, van Wanrooij EJ, de Vos P, Krueger J, Reisfeld RA, et al. Vaccination against TIE2 reduces atherosclerosis. Atherosclerosis. 2009;204(2):365-71. [PubMed ID: 19022447]. https://doi.org/10.1016/j.atherosclerosis.2008.09.039.
-
33.
O'Brien C. Vaccine for atherosclerosis. Mol Med Today. 1997;3(6):231. [PubMed ID: 9211407].
-
34.
Petrovan RJ, Kaplan CD, Reisfeld RA, Curtiss LK. DNA vaccination against VEGF receptor 2 reduces atherosclerosis in LDL receptor-deficient mice. Arterioscler Thromb Vasc Biol. 2007;27(5):1095-100. [PubMed ID: 17303776]. https://doi.org/10.1161/ATVBAHA.106.139246.
-
35.
Tsiantoulas D, Diehl CJ, Witztum JL, Binder CJ. B cells and humoral immunity in atherosclerosis. Circ Res. 2014;114(11):1743-56. [PubMed ID: 24855199]. https://doi.org/10.1161/CIRCRESAHA.113.301145.
-
36.
Shaw DJ, Seese R, Ponnambalam S, Ajjan R. The role of lectin-like oxidised low-density lipoprotein receptor-1 in vascular pathology. Diab Vasc Dis Res. 2014;11(6):410-8. [PubMed ID: 25216847]. https://doi.org/10.1177/1479164114547704.