Abstract
Background:
Distribution of mitochondrial oxidative energy metabolism is a characteristic of cardiomyocyte dysfunction and congenital familial and sporadic heart diseases. Several studies have indicated that mutations in mitochondrial DNA, particularly in tRNAs and MT-CYB coding for cytochrome B in complex III (CIII), were associated with cardiologic disorders. We hypothesized that mutations in these positions might play an important causal or modifying role in congenital heart defects. Congenital heart diseases (CHDs) are the most common anomaly in newborns and the leading non-infectious cause of mortality in the first year of life. These defects are multifactorial complex diseases resulting from both genetic predisposition and environmental risk factors. Despite advances in molecular medicine, the genetic mechanism underlying mitochondrial genetic determinants of development of CHD has remained poorly understood.Objectives:
The present study aimed to identify the association between mitochondrial mutations in tRNAGlu and Cytb genes and CHD in Iranian patients.Methods:
In this case-control study, tRNAGlu and MT-CYB genes were analyzed in DNA isolated from peripheral blood of 84 Iranian pediatric patients with non-familial CHD and 72 controls for alterations. The cases and healthy controls were matched regarding age and gender.Results:
A total of three nucleotide variations were detected by Polymerase chain reaction-single-strand conformation polymorphism (PCR-SSCP) and sequencing. One of them was located in tRNAGlu (A14696T) found in a child with tetralogy of fallot and ventricular septal defect, which was assessed as a pathogen mutation. The second mutation was a homoplasmic variant (m.14766C > T) in cytb gene and was not detected in the healthy controls.This variant altered an amino acid (T7I) with moderately interspecific amino acid conservation indicative of the functional importance of the residue. Finally, a homoplasmic synonymous variation (T14783C) was observed in 70.4% of the CHD patients.Conclusions:
The results suggested that mitochondrial mutations might be associated with CHD in Iranian pediatric patients. Thus, more patients with CHD should be examined for mutations in mtDNA in order to clarify the role of variants.Keywords
1. Background
Despite advances in preventive and medical care strategies, the etiology of congenital heart diseases (CHDs) is not completely understood yet (1). CHD affects the structure and function of the heart in approximately 1% of all live births and can occur through a heterogeneous and complex process. Besides, it can be caused by several genes or multifactorial conditions resulting from interactions between environmental and genetic risk factors, including infections, drugs, maternal exposures, and teratogens. In order to find genetic factors in CHD, early studies on familial CHD often showed different malformations, such as ventricular septal defects (VSDs), tetralogy of fallot (TOF), and atrial septal defects (ASDs) (2, 3). Now, efforts for identifying the genetic determinants of CHD have been focused on nuclear genomes, discovering that several genes were associated with the risk of CHD (4). However, the mitochondrial genetic effects on the progression of CHD have remained poorly explored. Therefore, it is obvious that any study dealing with involvement of mtDNA sequence alterations in CHDs may represent interest for further understanding of genetic basis and molecular mechanisms of CHD development. mitochondrial respiratory chain (MRC) defects are responsible for a large variety of clinical syndromes in adults and children (5). It has been determined that reduction in mitochondrial respiration was the leading cause of increase in oxidative stress, defective contractile and intracellular regulatory proteins, decrease in energy production, aberrant apoptotic induction, and selective cardiac dysfunctions (6). Recent studies have also suggested that alterations in metabolic levels of energy in mitochondria might be associated with defects in heart myofilament functions and cause cardiomyocytes death (7). Cardiac manifestations of mitochondrial disorders include arrhythmias, heart failure, stroke, and types of cardiomyopathy (8-10). Mitochondria contain their own DNA (mtDNA), which is a circular, 16,569 bp sequence with 37 genes encoding for 13 structural proteins all of which being subunits of various respiratory chain complexes, 22 transfer RNAs, and 2 ribosomal RNAs (11). Mitochondrial oxidative phosphorylation (OXPHOS) is performed by five large multisubunit complexes (I-V) (12). Point mutations that arise in the mtDNA can occur in both tRNA genes and genes encoding MRC subunits. They are thus associated with a large number of clinical presentations. One of the mtDNA located genes associated with cardiac disease is MT-CYB encoding for Cytochrome-b. The base substitutions in human MT-CYB gene are associated with a wide spectrum of diseases, such as cardiac and muscular defects (13, 14). MT-CYB, a component of complex III, is an extremely conserved protein, reflecting its fundamental role in energy production in mitochondria. It catalyzes the reversible electron transfer from ubiquinol to Cytochrome c coupled to proton translocation (Q-cycle) (15). The present study aims to assess the role of variations in tRNAGlu and MT-CYB genes in Iranian CHD patients and controls and to identify the potential pathogenic mutations associated with CHD.
2. Objectives
The present study also aims to identify the association between rs4977574 polymorphism in ANIRL gene and CAD incidence in a population of Iranian patients.
3. Methods
3.1. Patients
This case-control study was performed on 84 unrelated pediatric patients with congenital heart malformations selected from 112 cases referred to the department of cardiac surgery in Afshar hospital (Yazd, Iran) from 2013 to 2015. All the samples were obtained from the CHD patients undergoing cardiac surgery at Afshar cardiac hospital and were assessed by clinical history, physical examination, 12-lead electrocardiogram, and echocardiography. Also, 72 unrelated healthy individuals (24 males and 38 females) who were matched with the patients regarding age, sex, and ethnicity and had no family history of CHD were enrolled into the control group. In the patients group, 52 subjects (61.90%) had VSD, 20 (23.8%) suffered from ASD, and 12 (14.28%) had TOF. Besides, 63 participants were female (75%) and 21 were male (25%). Additionally, their median age at the time of surgery was 2.8 years (range 0 - 3.5 years). Recruitment of the patients and laboratory protocols in this study were approved by the ethics committee of Yazd university. After obtaining written informed consents, a 5 mL peripheral blood sample was collected from each patient. Then, genomic DNA was extracted from blood leukocytes using DNA isolation kit (Qiagene Co, Tehran, Iran).
3.2. Mutation Screening of MT-CYB
By using two pairs of specific primers designed from the genomic sequence of the mitochondrial MT-CYB gene (14747 - 15887), the two coding segments of the MT-CYB gene (initial and terminal segments) were amplified by polymerase chain reaction (PCR) (Table 1). The primers were designed by primer design software (Primer Premier 5.0; Premier Biosoft Inc., Canada) and their secondary structure was examined using Gene Runner, version 3.05 (Hastings Software Inc. Hastings, NY, USA, http://www.generunner.com).PCR was performed in a 25 µL mixture containing 1 × PCR buffer, 25 ng genomic DNA, 10 pmol/each forward and reverse primers, 1.5 mmol/L MgCl2, 0.2 mmol/L in each dNTPs, and 1 U Taq DNA polymerase. The amplification program included one cycle of an initial denaturation step at 94°C for 5 mintes followed by 35 cycles at 94°C for 35 seconds, 57°C for 45 seconds, 72°C for 50 seconds, and a final extension at 72°C for 10 minutes. The amplified products were then analyzed for size on 1.5% agarose gel. For mutation screening, Single-Strand conformation polymorphism (SSCP) analysis was performed in all the patients and controls. For SSCP assay, the PCR products were heat-denatured at 93°C for 3 minutes, chilled on ice for 3 minutes, and loaded onto a polyacrylamide/TBE 0.5x gel. SSCP was carried out using Payapajoohesh vertical gel. The gel concentrations and running conditions were as follows: 8% polyacrylamide gel, 16 hours, room temperature, 200 V. After the run, the gel was removed from the apparatus and the DNA bands were visualized through silver staining. The typical gene variants got sequenced from a commercial agency (Macrogene Seoul, South Korea). In addition, the online multiple sequence alignment software, ClustalW2 (http://www.ebi.ac.uk/tools/msa/clustalw2/) and Blast analysis were used to determine the homology of the sequences obtained in the study and all other sequences of other species.
The Primers Used for Amplification by PCR
Primer pairs 1 | Nucleotides | Primer sequence |
---|---|---|
Forward 1 | 14561 - 14580 | 5’ - ACCACACCGCTAACAATCAA – 3’ |
Reverse 1 | 14840 - 14821 | 5’ - TTCATCATGCGGAGATGTTG – 3’ |
Primer pairs 2 | ||
Forward 2 | 15791 - 15810 | 5’ - ATCATTGGACAAGTAGCATA – 3’ |
Reverse 2 | 16150 - 16131 | 5’ - GTGGTCAAGTATTTATGGTA – 3’ |
3.3. Software and Databases
In this study, PolyPhen-2 (http://genetics.bwh.harvard.edu/pph2/) was utilized for prediction of the functional consequences of mutations and damaging effect of missense mutations on protein sequence. Besides, sequence alignment was performed using the standard protein blast (blastp) program available at the national center for biotechnology information (NCBI) website (http://www.ncbi.nlm.nih.gov/Blastp) and the ClustalW program (http://bioinfo.hku.hk/services/analyseq/cgi-bin/clustalw_in.pl). Mamit-tRNA (http://mamit-trna.u-strasbg.fr) was also used for detection of the structural features of mammalian mitochondrial tRNAs and human diseases linked to point mutations in mitochondrial tRNA genes. Finally, in order to identify the hydrophobicity or hydrophilicity scales in proteins, degree of protein hydrophobicity was analyzed using a plot created by the ExPASy Protscale tool (http://web.expasy.org/protscale).
3.4. Statistical Analysis
Fisher’s exact test was used to determine the associated between mitochondrial polymorphisms and the genetic risk of CHD. The statistical analyses were performed using the GraphPad Prism software (GraphPad Software, Inc. USA) and P < 0.05 was considered to bestatistically significant.
4. Results
Mutation analysis in tRNAGlu and MT-CYB genes was performed using PCR-SSCP in a total of 84 unrelated Iranian pediatric CHD patients and 72 healthy subjects. According to the results, one mutation was located in tRNAGlu (A14696T) (Figure 1). This homoplasmic mutation was found in three children with TOF and VSD and occurred at the position that was highly evolutionarily conserved nucleotide of the corresponding tRNA (Figure 2). The results of Mamit tRNA site also showed that the position of A14696T mutation was T-stem and was previously reported as a pathogenic variation in progressive encephalomyopathy (16). This mutation has not been previously reported in various CHDs and was absent among the 72 unrelated Iranian controls. The second mutation was a homoplasmic non-synonymous one (m.14766C > T) within MT-CYB gene in 12 CHD patients (6 female children with TOF, 5 with VSD, and 1 male child with ASD), but not in the healthy controls (Figure 3). C to T transition at nucleotide 66 resulted in substitution of polar threonine with non-polar isoleucine at amino acid position 7. This alteration in amino acid might impair the enzymatic function of the Cytochrome b protein because of changes in the hydropathy determinants of the protein (hydropathy score was - 0.7 for polar threonine but 4.5 for isoleucine) (Figure 4). The results of SSCP analysis also showed that this mutation was homoplasmic and, consequently, it was considered for pathogenicity. Accordingly, three of them (T14783C) were homoplasmic synonymous mutations observed in 70.4% of our CHD patients (60 pediatric patients) and were considered to be neutral polymorphisms based on the previous reports (17, 18).
Sequence Analysis of the mtDNA of the Normal Control (a) and Patient (b) Revealed the Homoplasmic Mutation of an A to G at Position 14696 in tRNAGlu Gene in a Child with Tetralogy of Fallot and Ventricular Septal Defect SSCP Results (c)
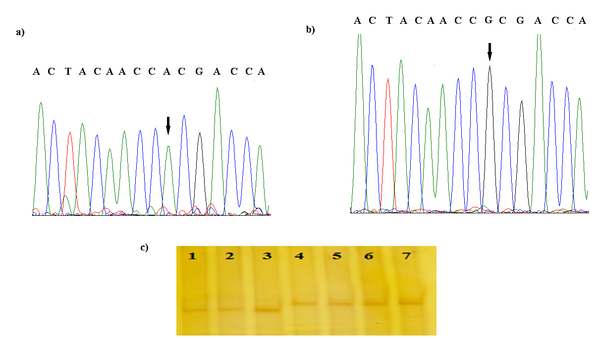
A, schematic representation of the tRNAGlu cloverleaf structure, showing the mutation in the T-domain and the affected base pair; B, comparison of this region of tRNAGlu gene sequences across different species showing that the mutated base pair Is conserved.
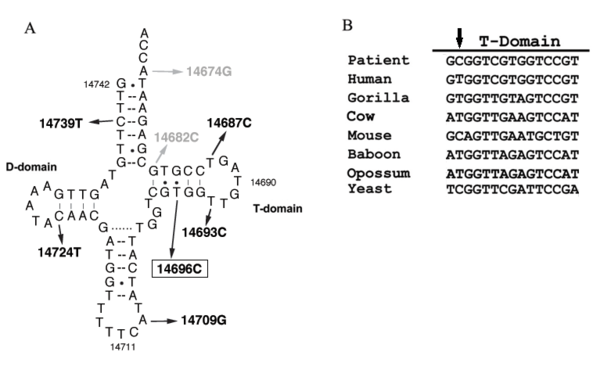
(a) DNA Sequencing Chromatogram Showing (Arrows) Homoplasmic Variant m.14766C > T in Cytb Gene in a Pediatric Patient with Atrial Septal Defect and TOF. (b) SSCP Gel Electrophoresis
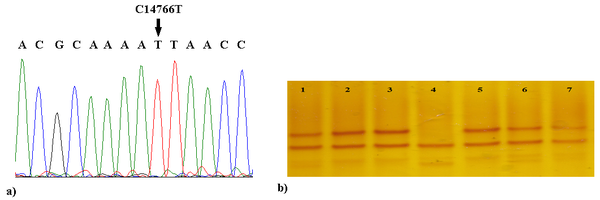
The Hydropathy Plot of the Amino Acids Sequence of MTCYB Protein
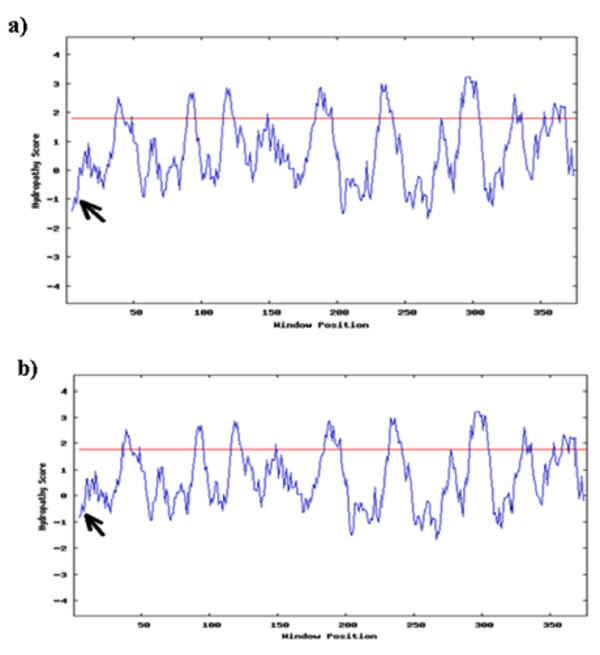
5. Discussion
MT-CYB plays a key role in the structure of mitochondria and is one of the 11 components of complex III in oxidative phosphorylation. Cytochrome b is the only mitochondrial DNA (mtDNA) encoding the subunit of respiratory complex III. It catalyzes the transfer of electrons from ubiquinol to Cytochrome c and utilizes the energy to translocate protons from inside to outside the mitochondrial inner membrane (15, 19). Mitochondrial Cytb is a hydrophobic and highly conserved protein containing several transmembrane domains and heme groups and has a fundamental role in ATP production. Most MT-CYB gene mutations that cause mitochondrial complex III deficiency change single protein building blocks (amino acids) in the Cytochrome b protein or lead to an abnormally short protein. These Cytochrome b alterations impair the formation of complex III, severely reducing the complex’s activity and oxidative phosphorylation. It is thought that the tissues that require a lot of energy, such as those in the muscles, brain, heart, liver, and kidneys, are most affected by reduction in oxidative phosphorylation. Damage to these tissues and organs leads to various features of mitochondrial complex III deficiency (20). Little information is presently available concerning mitochondrial respiratory and oxidative phosphorylation function in the congenital heart defects. The present study was the first molecular genetic analysis in tRNAGlu and MT-CYB genes mutations identified in the patients with CHD. Although these heart defects might be due to diverse etiologic factors, the presence of abnormal mitochondria in our present findings suggests that defects in mtDNA should be considered in patients with these disorders. Because heart tissue was not available for our molecular genetic analysis, the mutations of this region were investigated in the peripheral blood. According to the results, the C14766T mutation was considered to be pathogenic because it was never reported as a neutral polymorphism despite sequencing of the cyt b gene in normal individuals. Besides, C14766T mutation resulted in replacement of polar threonine with non-polar isoleucine at amino acid position 7 (T7I). Moreover, because this position has to be functional for proton transfer to occur, the C14766T mutation is likely to inhibit proton transfer and to result in a partial block of the respiratory chain at complex III. We suggested that the cytb mutations were responsible for decreased complex III activity and might play a role in the pathophysiology of CHD. The findings of the current study revealed a mtSNP (T14783C), which was not previously reported in any cardiac disorders. The rate of this synonymous substitution was significantly higher in the CHD patients compared to the controls (P = 0.0017). Here, we reported three children with TOF, VSD, and a previously described A-to-G mutation at nucleotide position 96 in the gene encoding tRNA glutamic acid (tRNAGlu). This base pair is in the T-stem of the tRNA predicted cloverleaf and was conserved among species. It is often difficult to distinguish pathogenic mtDNA mutations from neutral polymorphisms, especially when they occur within tRNA genes, because they do not produce alterations in polypeptide sequences. The alteration of secondary structure of this tRNA by this mtDNA mutation may lead to a failure in tRNA metabolism, thereby causing impairment of mitochondrial protein synthesis. Therefore, this mtDNA mutation may have a potential modifier role in increasing the severity of symptoms in Iranian pediatric patients with CHD. In conclusion, the current study revealed three homoplasmic mutations in mitochondrial tRNAGlu and cyt b genes in pediatric patients with congenital heart defects. These mutations were associated with the severity of heart defects in our patients, but not in the controls. This suggests the importance of mitochondrial modifier genes associated with environmental factors and responsible nuclear genes in development and evolution of the embryonic heart. Overall, the study findings demonstrated that defects in mtDNA should be considered in patients with CHDs. However, the small sample size of this study does not allow establishment of such a correlation. Thus, additional studies are required to identify the mechanisms by which these mutations affect cardiac development.
Acknowledgements
References
-
1.
Huang JB, Liu YL, Sun PW, Lv XD, Du M, Fan XM. Molecular mechanisms of congenital heart disease. Cardiovasc Pathol. 2010;19(5):183-93. [PubMed ID: 19747853]. https://doi.org/10.1016/j.carpath.2009.06.008.
-
2.
Garg V. Insights into the genetic basis of congenital heart disease. Cell Mol Life Sci. 2006;63(10):1141-8. [PubMed ID: 16568242]. https://doi.org/10.1007/s00018-005-5532-2.
-
3.
Mitchell ME, Sander TL, Klinkner DB, Tomita-Mitchell A. The molecular basis of congenital heart disease. Semin Thorac Cardiovasc Surg. 2007;19(3):228-37. [PubMed ID: 17983950]. https://doi.org/10.1053/j.semtcvs.2007.07.013.
-
4.
Clark KL, Yutzey KE, Benson DW. Transcription factors and congenital heart defects. Annu Rev Physiol. 2006;68:97-121. [PubMed ID: 16460268]. https://doi.org/10.1146/annurev.physiol.68.040104.113828.
-
5.
Ren J, Pulakat L, Whaley-Connell A, Sowers JR. Mitochondrial biogenesis in the metabolic syndrome and cardiovascular disease. J Mol Med (Berl). 2010;88(10):993-1001. [PubMed ID: 20725711]. https://doi.org/10.1007/s00109-010-0663-9.
-
6.
Sue CM, Schon EA. Mitochondrial respiratory chain diseases and mutations in nuclear DNA: a promising start? Brain Pathol. 2000;10(3):442-50. [PubMed ID: 10885663].
-
7.
Brega A, Narula J, Arbustini E. Functional, structural, and genetic mitochondrial abnormalities in myocardial diseases. J Nucl Cardiol. 2001;8(1):89-97. [PubMed ID: 11182713]. https://doi.org/10.1067/mnc.2001.112755.
-
8.
Hagen CM, Aidt FH, Havndrup O, Hedley PL, Jespersgaard C, Jensen M, et al. MT-CYB mutations in hypertrophic cardiomyopathy. Mol Genet Genomic Med. 2013;1(1):54-65. [PubMed ID: 24498601]. https://doi.org/10.1002/mgg3.5.
-
9.
Obayashi T, Hattori K, Sugiyama S, Tanaka M, Tanaka T, Itoyama S, et al. Point mutations in mitochondrial DNA in patients with hypertrophic cardiomyopathy. Am Heart J. 1992;124(5):1263-9. [PubMed ID: 1442494].
-
10.
Papadimitriou A, Neustein HB, Dimauro S, Stanton R, Bresolin N. Histiocytoid cardiomyopathy of infancy: deficiency of reducible cytochrome b in heart mitochondria. Pediatr Res. 1984;18(10):1023-8. [PubMed ID: 6093033].
-
11.
Anderson S, Bankier AT, Barrell BG, de Bruijn MH, Coulson AR, Drouin J, et al. Sequence and organization of the human mitochondrial genome. Nature. 1981;290(5806):457-65. [PubMed ID: 7219534].
-
12.
DiMauro S, Schon EA. Mitochondrial respiratory-chain diseases. N Engl J Med. 2003;348(26):2656-68. [PubMed ID: 12826641]. https://doi.org/10.1056/NEJMra022567.
-
13.
Wibrand F, Ravn K, Schwartz M, Rosenberg T, Horn N, Vissing J. Multisystem disorder associated with a missense mutation in the mitochondrial cytochrome b gene. Ann Neurol. 2001;50(4):540-3. [PubMed ID: 11601507].
-
14.
Zarrouk Mahjoub S, Mehri S, Ourda F, Finsterer J, Ben Arab S. Novel m.15434C>A (p.230L>I) Mitochondrial Cytb Gene Missense Mutation Associated with Dilated Cardiomyopathy. ISRN Cardiol. 2012;2012:251723. [PubMed ID: 22811935]. https://doi.org/10.5402/2012/251723.
-
15.
Blakely EL, Mitchell AL, Fisher N, Meunier B, Nijtmans LG, Schaefer AM, et al. A mitochondrial cytochrome b mutation causing severe respiratory chain enzyme deficiency in humans and yeast. FEBS J. 2005;272(14):3583-92. [PubMed ID: 16008558]. https://doi.org/10.1111/j.1742-4658.2005.04779.x.
-
16.
Uusimaa J, Finnila S, Remes AM, Rantala H, Vainionpaa L, Hassinen IE, et al. Molecular epidemiology of childhood mitochondrial encephalomyopathies in a Finnish population: sequence analysis of entire mtDNA of 17 children reveals heteroplasmic mutations in tRNAArg, tRNAGlu, and tRNALeu(UUR) genes. Pediatrics. 2004;114(2):443-50. [PubMed ID: 15286228].
-
17.
Ranaweera L, Kaewsutthi S, Win Tun A, Boonyarit H, Poolsuwan S, Lertrit P. Mitochondrial DNA history of Sri Lankan ethnic people: their relations within the island and with the Indian subcontinental populations. J Hum Genet. 2014;59(1):28-36. [PubMed ID: 24196378]. https://doi.org/10.1038/jhg.2013.112.
-
18.
Rollins B, Martin MV, Sequeira PA, Moon EA, Morgan LZ, Watson SJ, et al. Mitochondrial variants in schizophrenia, bipolar disorder, and major depressive disorder. PLoS One. 2009;4(3):4913. [PubMed ID: 19290059]. https://doi.org/10.1371/journal.pone.0004913.
-
19.
Berry EA, Guergova-Kuras M, Huang LS, Crofts AR. Structure and function of cytochrome bc complexes. Annu Rev Biochem. 2000;69:1005-75. [PubMed ID: 10966481]. https://doi.org/10.1146/annurev.biochem.69.1.1005.
-
20.
Casademont J, Miro O. Electron transport chain defects in heart failure. Heart Fail Rev. 2002;7(2):131-9. [PubMed ID: 11988637].