Abstract
Background:
Methamphetamine is a commonly abused drug, and its neurotoxic effects have increased in recent years. There is growing evidence that buprenorphine, a widely used drug for pain management, reduces the harmful effects of addiction and is effective in treating opioid dependence.Objectives:
This study aimed to investigate the interaction between methamphetamine and buprenorphine on analgesia and the expression of BDNF and CREB genes in the spinal cords of rats.Methods:
In this study, 56 male Wistar rats (200 ± 50 g) were randomly assigned to eight groups: Control, sham, methamphetamine, two buprenorphine groups, two methamphetamine + buprenorphine groups, and a deprivation group. A one-way ANOVA test was used to analyze the data.Results:
Intraperitoneal injection of methamphetamine (10 mg/kg) induced analgesia (P < 0.05) and increased CREB gene expression in the lumbar spinal cord (P < 0.01), whereas buprenorphine alone (6 and 10 mg/kg) did not induce significant analgesia or alter BDNF gene expression in the spinal cord. Pharmacological interactions revealed that buprenorphine (6 and 10 mg/kg, i.p.) enhanced the analgesic effect of methamphetamine. Additionally, while the lower dose of buprenorphine reduced methamphetamine's effect on CREB gene expression (P < 0.05), the higher dose potentiated the effect of methamphetamine on BDNF gene expression (P < 0.01) without significantly affecting CREB gene expression.Conclusions:
The findings suggest that buprenorphine may enhance the analgesic effects of acute methamphetamine administration and modulate gene expression in the lumbar spinal cord of male Wistar rats.Keywords
1. Background
Drug testing in pain management is essential for ensuring patient compliance with prescribed opioids. However, the destructive effects of addictive drugs on the spinal cord have been less studied (1). Research has shown that 25% of patients are already using opioids at the time of surgery (2). Methamphetamine (Meth), a highly addictive central nervous system stimulant, poses a significant threat to public health due to its acute side effects, including a strong propensity for dependence, long-term neurotoxicity, and hyperthermia (3). Methamphetamine abuse is a serious public health concern, as it is associated with adverse neuropsychiatric consequences such as addiction, psychosis, and cognitive impairments in humans (4).
Buprenorphine (BUP), a partial μ-opioid agonist, is considered less harmful than other opioid agonists, such as methadone, and is eliminated from the body more quickly (5). BUP is widely used for pain management, reduces the harmful effects of addiction, and is effective in treating opioid dependence (6). However, BUP can present challenges to effective acute pain management during perioperative and emergency situations (7). At different doses, BUP affects synaptic strength in spinal C-fibers (8).
The molecular mechanisms underlying the neurotoxicity of amphetamines, including Meth, are complex and ultimately lead to severe dysfunction in dopaminergic, and in some species, serotonergic neurotransmission (9, 10). The neurological effects of chronic methamphetamine abuse disrupt various neurotransmitter systems, including the dopamine system in the brain (11, 12). Meth appears to depress the transmission of monosynaptic reflexes in the spinal cord by influencing dopaminergic mechanisms (13).
The adverse effects of addictive drugs on the central nervous system (CNS) are due to their actions on specific genes and transcription factors, which are becoming increasingly well-known. Meth treatment has been shown to decrease the expression of genes such as myelin-associated glycoprotein (MAG), myelin basic protein (MBP), and cyclin-dependent kinase 5 (CDK5), and also induces demyelination in the spinal cord (2). Previous studies have emphasized that brain-derived neurotrophic factor (BDNF) is a neuronal growth factor associated with nerve cell survival, neuronal protection, and synaptic plasticity (14). The application of BDNF to the spinal cord promotes neuronal survival and sprouting in lesioned neurons in rats (15). Brain-derived neurotrophic factor also mediates the activity of the dopamine system and addictive drugs (16, 17), and acute and chronic use of opiates such as Meth may affect BDNF gene expression differently (18, 19). After opioid withdrawal, serum BDNF levels gradually increase, leading to nerve growth and pain associated with craving (20).
cAMP response element-binding (CREB) Protein is a transcription factor that regulates genes related to neuronal survival, neuroprotection, and neural plasticity, including BDNF (21). The role of CREB signaling in Meth addiction is supported by Meth-induced CREB phosphorylation in the rat striatum (22). cAMP response element-binding mediates both the positive and negative effects of opioid drugs (23), and BDNF/TrkB-mediated signaling is activated by CREB phosphorylation (23). Spinal nerve ligation increases CREB phosphorylation in the ipsilateral spinal cord, and hypersensitivity following spinal nerve ligation is reduced by intrathecal CREB antisense administration (24). Increased BDNF expression has been observed in lumbar dorsal root ganglia (DRGs) from rats with chronic heart failure compared to sham rats (25).
2. Objectives
Furthermore, tail flick tests are commonly used to assess opioid analgesia (26). Since treatment with BDNF has been shown to reduce nociceptive thresholds in rats (27), this study aimed to investigate the role of Meth and BUP interaction on analgesia and the expression of BDNF and CREB genes in the spinal cords of rats.
3. Methods
3.1. Experimental Designs
Male Wistar rats, weighing 250 - 270 g at the time of surgery, were used in the study. The animals were housed in the animal facility of Tabriz University, with free access to food and water, maintained on a 12:12 h light/dark cycle (lights on at 07:00 h) and a controlled temperature (23 ± 2ºC). The study adhered to ethical guidelines for the investigation of experimental pain in animals (28). The drugs used in the study included Methamphetamine crystals (Sigma Chemical Co.), Buprenorphine (Faran Shimi Co.), triazole solution, chloroform, isopropanol, 75 - 70% ethanol, and 1% agarose gel. Methamphetamine and Buprenorphine were dissolved in sterile 0.9% saline and administered intraperitoneally (i.p.) for five consecutive days.
The injection volumes and dosing patterns were based on previous studies (18, 29, 30). The ethical approval for this descriptive-analytical investigation was granted by the Tabriz University of Medical Sciences Ethics Committee.
3.2. Experimental Protocol
Each group: The control group did not receive any drugs. The saline-treated group was administered 1 mL of saline (i.p.) once daily for five consecutive days. The Meth group received 10 mg/kg of Meth (i.p.) each day for five consecutive days. The BUP groups were administered 6 mg/kg and 10 mg/kg of buprenorphine (i.p.) daily for five consecutive days. In the Meth + BUP groups, Meth (10 mg/kg) was given daily for five days, followed by buprenorphine (6 mg/kg or 10 mg/kg, i.p.) for the same duration. Finally, the Meth-deprived group (Dep) was deprived of Meth for four days after the last injection.
3.3. Nociception Assay
Based on the D'Amour and Smith approach, the Tail Flick test (Burj Sanat Co.) was used to assess the nociceptive threshold (31). When the middle third of the tail is exposed to intense heat from a scorching light, the animal briefly retracts its tail (latency time). The tail flick (TF) apparatus (Sparco, Iran) was calibrated to produce a latency period of 4 to 5 seconds in an intact animal by adjusting the light intensity. A 15-second cutoff time was set to prevent any tissue damage. For each set of the TF test, latency time was measured three times at one-minute intervals. The average of these recordings was taken as the tail flick latency, or thermal pain threshold, and it was measured 30 minutes after drug administration. This time frame was considered sufficient for the drug to achieve complete systemic distribution in adult rats. The maximum possible effect percentage (MPE%) was calculated using the following formula (32).
3.4. Gene Expression Analyses
At the conclusion of each trial, the rats in all experimental groups were given deep chloroform anesthesia. The rats' heads were separated to expose the vertebral column. A precise incision was made 6 - 8 mm above and below the lumbar spinal cord (33), and the sections were carefully placed in 1.5 mL sterile DEPC water tubes. The samples were then frozen at -80°C. For RNA extraction, 100 mg of tissue was homogenized in a grinder device using 700 µL of triazole solution. After homogenization, 200 µL of chloroform was added, and the mixture was incubated on ice for 10 to 15 minutes before being transferred to a microtube.
The microtube was centrifuged at 13,000 rpm for 15 minutes at 4°C using a chilled centrifuge (Sigma Co.). After centrifugation, the RNA-containing silt was dried for 15 minutes at 60°C in a dry bath. The sample concentration was measured using a Nanodrop device (Thermo Co.). The quality and concentration of the extracted RNA were assessed by two methods: One percent agarose gel electrophoresis and spectrophotometry using a Nanodrop instrument.
The first strand of cDNA was synthesized from the total RNA isolated in the previous step, using a single-stranded kit (TAKARA) in a final volume of 20 µL (34). Table 1 lists the primers and their sequences. A real-time PCR technique was employed to examine the expression of the BDNF and CREB genes and their interactions. Roche and SYBR Green were used as internal controls to determine the expression levels of the CREB and BDNF genes. The SYBR Green quantitative real-time PCR method has been widely used in many investigations for identifying, measuring, and distinguishing species and is recognized as an effective approach for quantitative detection and species discrimination (35).
Sequence of Primers Used for Brain-Derived Neurotrophic Factor and Brain-Derived Neurotrophic Factor cAMP Response Element-Binding Gene
Gene Name and Primer Type | Primer Sequence | Proliferation Piece Length |
---|---|---|
BDNF | 85bp | |
Forward | AAACCATAACCCCGCACACTC | |
Reverse | AGCCTTCATGCAACCGAAGTA | |
CREB | 85bp | |
Forward | ATGGACTCTGGAGCAGACAAC | |
Reverse | ATCTGTGGCTGGGCTTGAACT |
3.5. Statistical Analysis
Using SPSS-22 software, a one-way ANOVA was conducted to analyze the differences in the mean expression of the genes under study across the various experimental groups. Statistical significance for group differences was established when P-values were less than 0.05. Additionally, Excel 2010 was used for plotting charts to visually represent the data and results.
4. Results
4.1. The Role of Intraperitoneal BUP, Meth, and Intervention Injection of the BUP and Meth on Tail Flick Latency in the Lumbar Spinal Cord of Rats
Methamphetamine injection at 10 mg/kg for five consecutive days significantly increased tail flick latency (P < 0.05; Figure 1, left panel) compared to the control group, as revealed by one-way ANOVA and post hoc Tukey analysis. However, tail flick latency was not affected by buprenorphine doses (6 and 10 mg/kg) administered for five consecutive days (P > 0.05; Figure 1, middle panel). Additionally, the findings demonstrated that methamphetamine (10 mg/kg i.p.) for five days had a more pronounced effect on Tail Flick latency when combined with intraperitoneal buprenorphine at doses of 6 and 10 mg/kg (P < 0.01; Figure 1, right panel). The results indicate that methamphetamine administered acutely has an analgesic effect, and drug deprivation did not significantly impact the rats. Furthermore, the findings suggest that buprenorphine, when applied alone, does not provide effective pain relief but may enhance methamphetamine's analgesic effects when combined.
Male rat tail flick latency as a function of methamphetamine deprivation syndrome and intraperitoneal injection of methamphetamine (10 mg/kg i.p.) alone or in combination with buprenorphine (6 and 10 mg/kg). * P < 0.05, ** P < 0.01 with relation to the baseline cohort. For each data set, the mean ± SEM (n = 7) is used.
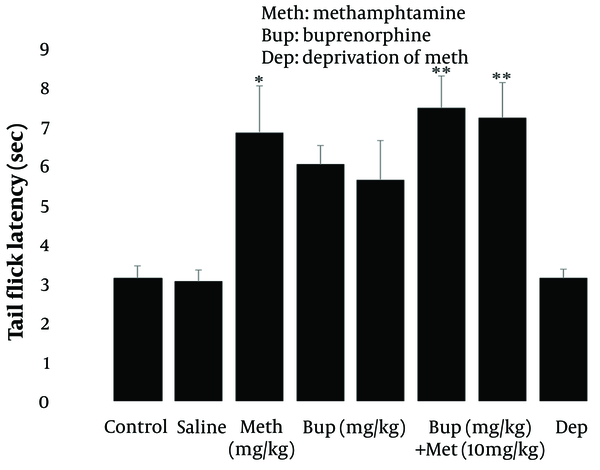
4.2. The Role of Intraperitoneal BUP, Meth, and Intervention Injection of the BUP and Meth on the Expression of BDNF and CREB Genes in the Lumbar Spinal Cord of Rats
One-way ANOVA and post hoc Tukey analysis revealed that the injection of 10 mg/kg Meth (i.p.) for five consecutive days did not significantly alter the expression of BDNF (P > 0.05; Figure 2, left panel), whereas it significantly increased the level of CREB (P < 0.01; Figure 3, left panel) in the lumbar spinal cord of rats compared to the control group. These results indicate that Meth administered over this period does not modulate BDNF gene expression but does influence CREB expression. Furthermore, Meth deprivation for four days after the last injection did not significantly affect the expression of BDNF (P > 0.05; Figure 2, right panel] or CREB genes (P > 0.05; Figure 3, right panel] compared to the Meth group.
Effect of intraperitoneal injection of methamphetamine (10 mg/kg i.p) alone or in combination with buprenorphine (6 and 10 mg/kg) and also methamphetamine deprivation syndrome on the expression of BDNF gene in the lumbar spinal cord of male rats. ** P < 0.01 compared to the control group. All data are expressed as mean ± SEM (n = 7).
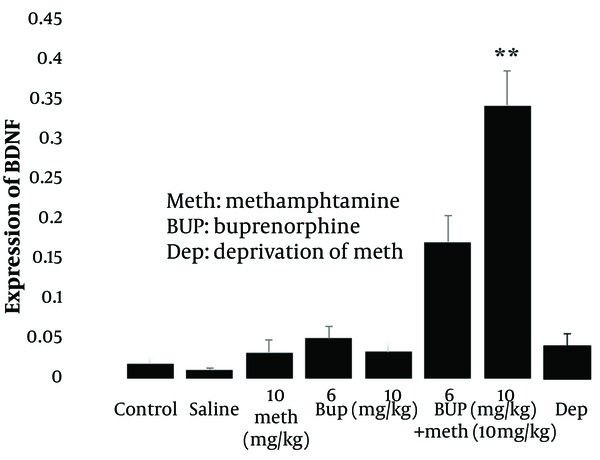
Effect of intraperitoneal injection of methamphetamine (10 mg/kg i.p) alone or in combination with buprenorphine (6 and 10 mg/kg) and also methamphetamine deprivation syndrome on the expression of CREB gene in the lumbar spinal cord of male rats. ** P < 0.01 compared to the control group and + P < 0.5 compared to the methamphetamine group. All data are expressed as mean ± SEM (n = 7).
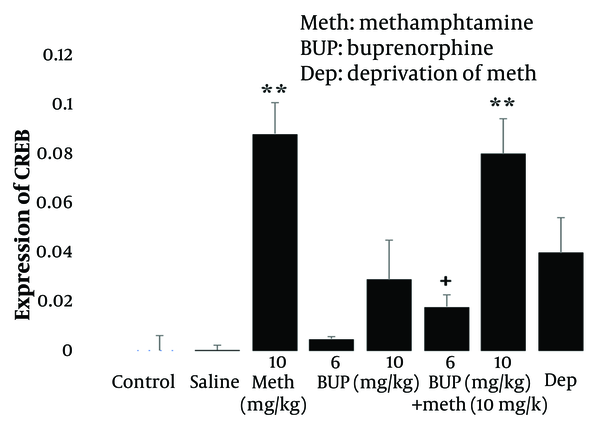
Additionally, administration of buprenorphine (BUP) at doses of 6 and 10 mg/kg for five consecutive days did not significantly alter the expression of BDNF (P > 0.05; Figure 2, middle panel] or CREB (P > 0.05; Figure 3, middle panel]. This suggests that the doses of BUP applied did not significantly affect the expression of these genes in the lumbar spinal cord of rats.
Finally, the interaction of the drugs revealed that the injection of 6 mg/kg BUP for five consecutive days reduced Meth’s effect on CREB expression (P < 0.05; Figure 3, right panel], but did not significantly affect BDNF gene expression compared to the Meth group (P > 0.05; Figure 2, right panel]. Conversely, the 10 mg/kg dose of BUP potentiated Meth’s effect on BDNF expression (P < 0.01; Figure 2, right panel], but their interaction did not significantly alter CREB expression compared to the Meth group, although their effect remained significant compared to the control group (P < 0.01; Figure 3, right panel]. These findings suggest that the differential effects of BUP and Meth on BDNF and CREB gene expression may be dose-dependent.
5. Discussion
The present data showed that the injection of 10 mg/kg Meth (i.p.) for five consecutive days did not alter the expression of the BDNF gene, whereas it increased the expression of the CREB gene in the lumbar spinal cord and prolonged the Tail Flick latency in rats. Our findings are consistent with some previous studies but differ from others. Chronic Meth injections have been reported to induce antinociceptive effects in rats (36). Meth has been shown to increase BDNF expression in the nucleus accumbens (NAc), improve memory, and reduce pain (33). Additionally, BDNF expression in mesocorticolimbic areas has been found to increase following exposure to dopamine agonists, such as amphetamine (37). However, other reports suggest that chronic use of opioid substances not only causes addiction and drug tolerance but also reduces BDNF expression and disrupts central and peripheral nervous system function (38). For instance, heroin use has been associated with a decrease in BDNF serum levels (21), while BDNF levels increase in the ventral tegmental area (VTA), accumbens, and amygdala after withdrawal from cocaine self-administration (39).
It has been proposed that chronic Meth administration decreases BDNF, CREB (total and phosphorylated), Akt-1 protein expression, and GSK3 levels in the hippocampus, leading to nerve damage, cell apoptosis, inflammation, and oxidative stress (40). The differences in BDNF gene expression observed in this study compared to others may be attributed to factors such as whether the drug was administered acutely or chronically, the dosage, the methods used, and the type of drug administered (13, 41). Furthermore, repeated Meth administration has been shown to attenuate the inhibition of dopamine release from the NAc via presynaptic dynorphin-sensitive receptors (42). Meth appears to depress the transmission of the monosynaptic reflex in the spinal cord through its effects on dopaminergic mechanisms (13).
In this study, Meth administration for five days demonstrated only an antinociceptive effect, which aligns with previous research (36, 43). Despite its effect on increasing CREB gene expression, Meth did not significantly alter BDNF gene expression, suggesting it may not have addictive or destructive effects on the nervous system at this dose. Consequently, Meth deprivation did not alter the expression of the CREB or BDNF genes, supporting our hypothesis that the dose of Meth used was not sufficient to cause addiction.
The present results also showed that the intraperitoneal injection of the applied doses of BUP did not affect Tail Flick latency or the expression of BDNF and CREB genes. However, previous research has indicated that BUP exhibits an analgesic effect (7, 36). Prenatal exposure to BUP has been shown to increase the expression of BDNF, enhance BDNF/TrkB/PKA/CREB signaling, and promote neurogenesis (44). It is possible that the doses of buprenorphine used in this study were insufficient to induce an analgesic effect, which aligns with the findings of previous studies. At an analgesic dose, buprenorphine has been reported to depress synaptic strength at spinal C-fibers through the activation of spinal opioid receptors. In contrast, at an ultra-low dose, buprenorphine has been shown to facilitate synaptic strength at C-fibers in the dorsal horn (8).
Our results revealed that the applied doses of BUP, by modulating the expression of BDNF and CREB genes, could potentiate the analgesia induced by Meth administration. Specifically, treatment with 6 mg/kg of BUP reduced Meth’s influence on CREB gene expression and enhanced analgesia without affecting BDNF expression. In contrast, both the antinociceptive effect and the expression of BDNF and CREB genes were significantly increased in the Meth + high-dose BUP group compared to the control group. BUP not only alleviates withdrawal symptoms and acute pain in opioid addicts but also mitigates the behavioral damage caused by chronic Meth use (45). Consistent with our findings, the administration of 10 mg/kg Meth with bupropion has been shown to increase CREB gene expression in the hippocampus of male rats (46). It has been suggested that lithium may protect hippocampal neurons against Meth-induced oxidative stress, apoptosis, and inflammation by enhancing the expression of CREB (total and phosphorylated), BDNF, and Akt-1, although the mechanism is not yet fully understood (40).
Increased levels of BDNF may enhance the neuroprotective potential against Meth-induced toxicity or play a crucial role in Meth uptake in the brain (47). Meth-induced reductions in the size and dendritic structure of dopaminergic neurons in the VTA and NAc regions of the brain can be improved by BDNF administration (48). Additionally, alterations in the dopaminergic response to Meth under BUP treatment suggest that BUP may be effective in treating Meth addiction (49). Thioredoxin-1 (Trx-1) has been shown to protect the spinal cord from demyelination caused by Meth administration by suppressing ER stress and inflammation (2). Based on our results and previous studies, the interactions between Meth and BUP on gene expression differ from the effects of each drug administered alone. While both Meth and BUP alone have been shown to have anxiolytic effects in rats, their co-administration resulted in anxiogenic effects (43).
Both heroin and cocaine use have been associated with reduced serum levels of NGF, with heroin also linked to decreased BDNF levels (21). Rats treated with both BUP and Meth exhibited significantly faster reaction times, suggesting antinociceptive properties. Furthermore, Meth amplified the antinociceptive effects of BUP. These synergistic effects may involve the adrenergic, serotonergic, and/or dopaminergic systems (36). In summary, our results suggest that BUP may attenuate Meth's adverse effects in the spinal cord by influencing dopaminergic mechanisms (13) and modulating the BDNF/CREB signaling pathway (37, 39). Additionally, BUP enhances Meth's antinociceptive effects.
5.1. Conclusions
Taken together, BUP likely modulates Meth’s effects on CREB and BDNF gene expression and analgesia, providing protection against neuronal impairment in the spinal cord of rats. However, the effects of these drugs are dose-dependent.
References
-
1.
Yang L, Guo Y, Huang M, Wu X, Li X, Chen G, et al. Thioredoxin-1 Protects Spinal Cord from Demyelination Induced by Methamphetamine through Suppressing Endoplasmic Reticulum Stress and Inflammation. Front Neurol. 2018;9:49. [PubMed ID: 29467717]. [PubMed Central ID: PMC5808126]. https://doi.org/10.3389/fneur.2018.00049.
-
2.
Sandhu S, Calcaterra SL. How Do I Manage Acute Pain for Patients Prescribed Buprenorphine for Opioid Use Disorder? NEJM Evid. 2024;3(5):EVIDccon2300275. [PubMed ID: 38815158]. [PubMed Central ID: PMC11282871]. https://doi.org/10.1056/EVIDccon2300275.
-
3.
Chmiel J, Malinowska A, Rybakowski F, Leszek J. The Effectiveness of Mindfulness in the Treatment of Methamphetamine Addiction Symptoms: Does Neuroplasticity Play a Role? Brain Sci. 2024;14(4). [PubMed ID: 38671972]. [PubMed Central ID: PMC11047954]. https://doi.org/10.3390/brainsci14040320.
-
4.
Jayanthi S, Daiwile AP, Cadet JL. Neurotoxicity of methamphetamine: Main effects and mechanisms. Exp Neurol. 2021;344:113795. [PubMed ID: 34186102]. [PubMed Central ID: PMC8338805]. https://doi.org/10.1016/j.expneurol.2021.113795.
-
5.
Laffont CM, Ngaimisi E, Gopalakrishnan M, Ivaturi V, Young M, Greenwald MK, et al. Buprenorphine exposure levels to optimize treatment outcomes in opioid use disorder. Front Pharmacol. 2022;13:1052113. [PubMed ID: 36467036]. [PubMed Central ID: PMC9715596]. https://doi.org/10.3389/fphar.2022.1052113.
-
6.
Urits I, Pham C, Swanson D, Berardino K, Bandi P, Amgalan A, et al. The utilization of buprenorphine in chronic pain. Best Pract Res Clin Anaesthesiol. 2020;34(3):355-68. [PubMed ID: 33004153]. https://doi.org/10.1016/j.bpa.2020.06.005.
-
7.
Anderson TA, Quaye ANA, Ward EN, Wilens TE, Hilliard PE, Brummett CM. To Stop or Not, That Is the Question: Acute Pain Management for the Patient on Chronic Buprenorphine. Anesthesiol. 2017;126(6):1180-6. [PubMed ID: 28511196]. [PubMed Central ID: PMC7041233]. https://doi.org/10.1097/ALN.0000000000001633.
-
8.
Gerhold KJ, Drdla-Schutting R, Honsek SD, Forsthuber L, Sandkuhler J. Pronociceptive and Antinociceptive Effects of Buprenorphine in the Spinal Cord Dorsal Horn Cover a Dose Range of Four Orders of Magnitude. J Neurosci. 2015;35(26):9580-94. [PubMed ID: 26134641]. [PubMed Central ID: PMC4571500]. https://doi.org/10.1523/JNEUROSCI.0731-14.2015.
-
9.
Moszczynska A, Callan SP. Molecular, Behavioral, and Physiological Consequences of Methamphetamine Neurotoxicity: Implications for Treatment. J Pharmacol Exp Ther. 2017;362(3):474-88. [PubMed ID: 28630283]. [PubMed Central ID: PMC11047030]. https://doi.org/10.1124/jpet.116.238501.
-
10.
Sabrini S, Russell B, Wang G, Lin J, Kirk I, Curley L. Methamphetamine induces neuronal death: Evidence from rodent studies. NeuroToxicol. 2020;77:20-8. https://doi.org/10.1016/j.neuro.2019.12.006.
-
11.
Siefried KJ, Acheson LS, Lintzeris N, Ezard N. Pharmacological Treatment of Methamphetamine/Amphetamine Dependence: A Systematic Review. CNS Drugs. 2020;34(4):337-65. [PubMed ID: 32185696]. [PubMed Central ID: PMC7125061]. https://doi.org/10.1007/s40263-020-00711-x.
-
12.
Fornai F, Lenzi P, Gesi M, Soldani P, Ferrucci M, Lazzeri G, et al. Methamphetamine produces neuronal inclusions in the nigrostriatal system and in PC12 cells. J Neurochem. 2003;88(1):114-23. https://doi.org/10.1046/j.1471-4159.2003.02137.x.
-
13.
Ono H, Fukuda H. Effect of methamphetamine on rat spinal cord. Dopamine receptor-mediated depression of monosynaptic reflex. Neuropharmacol. 1984;23(6):637-42. [PubMed ID: 6462372]. https://doi.org/10.1016/0028-3908(84)90144-8.
-
14.
Di Carlo P, Punzi G, Ursini G. Brain-derived neurotrophic factor and schizophrenia. Psychiatr Genet. 2019;29(5):200-10. [PubMed ID: 31465000]. [PubMed Central ID: PMC7386257]. https://doi.org/10.1097/YPG.0000000000000237.
-
15.
Hiebert GW, Khodarahmi K, McGraw J, Steeves JD, Tetzlaff W. Brain-derived neurotrophic factor applied to the motor cortex promotes sprouting of corticospinal fibers but not regeneration into a peripheral nerve transplant. J Neurosci Res. 2002;69(2):160-8. [PubMed ID: 12111797]. https://doi.org/10.1002/jnr.10275.
-
16.
Karila L, Petit A, Cottencin O, Reynaud M. Dépendance à la méthamphétamine : de nombreuses conséquences et complications. La Presse Médicale. 2010;39(12):1246-53. https://doi.org/10.1016/j.lpm.2010.09.003.
-
17.
Crist RC, Reiner BC, Berrettini WH. A review of opioid addiction genetics. Curr Op Psycholo. 2019;27:31-5. https://doi.org/10.1016/j.copsyc.2018.07.014.
-
18.
Roque Bravo R, Faria AC, Brito-da-Costa AM, Carmo H, Mladenka P, Dias da Silva D, et al. Cocaine: An Updated Overview on Chemistry, Detection, Biokinetics, and Pharmacotoxicological Aspects including Abuse Pattern. Toxins (Basel). 2022;14(4). [PubMed ID: 35448887]. [PubMed Central ID: PMC9032145]. https://doi.org/10.3390/toxins14040278.
-
19.
Robinson TE, Kolb B. Structural plasticity associated with exposure to drugs of abuse. Neuropharmacolo. 2004;47:33-46. https://doi.org/10.1016/j.neuropharm.2004.06.025.
-
20.
Angelucci F, Ricci V, Pomponi M, Conte G, Mathe AA, Attilio Tonali P, et al. Chronic heroin and cocaine abuse is associated with decreased serum concentrations of the nerve growth factor and brain-derived neurotrophic factor. J Psychopharmacol. 2007;21(8):820-5. [PubMed ID: 17715210]. https://doi.org/10.1177/0269881107078491.
-
21.
Dibner C, Schibler U, Albrecht U. The Mammalian Circadian Timing System: Organization and Coordination of Central and Peripheral Clocks. Ann Rev Physiolo. 2010;72(1):517-49. https://doi.org/10.1146/annurev-physiol-021909-135821.
-
22.
Krasnova IN, Chiflikyan M, Justinova Z, McCoy MT, Ladenheim B, Jayanthi S, et al. CREB phosphorylation regulates striatal transcriptional responses in the self-administration model of methamphetamine addiction in the rat. Neurobiolo Dis. 2013;58:132-43. https://doi.org/10.1016/j.nbd.2013.05.009.
-
23.
Reus GZ, Stringari RB, Ribeiro KF, Ferraro AK, Vitto MF, Cesconetto P, et al. Ketamine plus imipramine treatment induces antidepressant-like behavior and increases CREB and BDNF protein levels and PKA and PKC phosphorylation in rat brain. Behav Brain Res. 2011;221(1):166-71. [PubMed ID: 21397634]. https://doi.org/10.1016/j.bbr.2011.02.024.
-
24.
Martin TJ, Ewan E. Chronic pain alters drug self-administration: implications for addiction and pain mechanisms. Exp Clin Psychopharmacol. 2008;16(5):357-66. [PubMed ID: 18837632]. [PubMed Central ID: PMC3788584]. https://doi.org/10.1037/a0013597.
-
25.
Schiller AM, Hong J, Xia Z, Wang HJ. Increased Brain-Derived Neurotrophic Factor in Lumbar Dorsal Root Ganglia Contributes to the Enhanced Exercise Pressor Reflex in Heart Failure. Int J Mol Sci. 2019;20(6). [PubMed ID: 30909643]. [PubMed Central ID: PMC6471760]. https://doi.org/10.3390/ijms20061480.
-
26.
Gades NM, Danneman PJ, Wixson SK, Tolley EA. The magnitude and duration of the analgesic effect of morphine, butorphanol, and buprenorphine in rats and mice. Contemp Top Lab Anim Sci. 2000;39(2):8-13. [PubMed ID: 11487232].
-
27.
Pezet S, Malcangio M, McMahon SB. BDNF: a neuromodulator in nociceptive pathways? Brain Res Brain Res Rev. 2002;40(1-3):240-9. [PubMed ID: 12589922]. https://doi.org/10.1016/s0165-0173(02)00206-0.
-
28.
Zimmermann M. Ethical guidelines for investigations of experimental pain in conscious animals. Pain. 1983;16(2):109-10. https://doi.org/10.1016/0304-3959(83)90201-4.
-
29.
Qu D, Zhang K, Chen L, Wang Q, Wang H. RNA-sequencing analysis of the effect of luteolin on methamphetamine-induced hepatotoxicity in rats: a preliminary study. PeerJ. 2020;8. e8529. [PubMed ID: 32071822]. [PubMed Central ID: PMC7007981]. https://doi.org/10.7717/peerj.8529.
-
30.
Gibson AE, Doran CM, Bell JR, Ryan A, Lintzeris N. A comparison of buprenorphine treatment in clinic and primary care settings: a randomised trial. Med J Aust. 2003;179(1):38-42. [PubMed ID: 12831383]. https://doi.org/10.5694/j.1326-5377.2003.tb05417.x.
-
31.
Shahbazi R, Hatami Nemati H, Ahmadi H, Zogoulipour F. Nociceptive threshold response and alterations of special genes expression during methamphetamine administration and treatment with buprenorphine. J Basic Res Med Sci. 2022;9(2):25-34.
-
32.
Fereidoni M, Javan M, Semnanian S, Ahmadiani A. [Hypothalamus Pituitary Adrenal axis and stimulatory G proteins signaling role in nociceptive changes induced by forced swim stress]. Physiol Pharmacol. 2007;10(4):291-302. FA.
-
33.
Ferrucci M, Pasquali L, Paparelli A, Ruggieri S, Fornai F. Pathways of methamphetamine toxicity. Ann N Y Acad Sci. 2008;1139:177-85. [PubMed ID: 18991862]. https://doi.org/10.1196/annals.1432.013.
-
34.
Kuba Y, Kyan H, Arakaki E, Takara T, Kato T, Okano S, et al. Molecular Epidemiological Study of Mumps Epidemics of 2015 in Okinawa, Japan. Jpn J Infect Dis. 2017;70(3):329-32. [PubMed ID: 28003601]. https://doi.org/10.7883/yoken.JJID.2016.390.
-
35.
Safdar M, Junejo Y. Development and validation of fast duplex real-time PCR assays based on SYBER Green florescence for detection of bovine and poultry origins in feedstuffs. Food Chem. 2015;173:660-4. [PubMed ID: 25466073]. https://doi.org/10.1016/j.foodchem.2014.10.088.
-
36.
Etaee F, Rezvani-Kamran A, Taheri M, Omidi G, Hasanein P, Komaki A. Comparing the Antinociceptive Effects of Methamphetamine, Buprenorphine, or Both After Chronic Treatment and Withdrawal in Male Rats. Basic Clin Neurosci. 2019;10(4):313-22. [PubMed ID: 32231768]. [PubMed Central ID: PMC7101515]. https://doi.org/10.32598/bcn.9.10.160.
-
37.
Meredith GE, Callen S, Scheuer DA. Brain-derived neurotrophic factor expression is increased in the rat amygdala, piriform cortex and hypothalamus following repeated amphetamine administration. Brain Res. 2002;949(1-2):218-27. [PubMed ID: 12213320]. https://doi.org/10.1016/s0006-8993(02)03160-8.
-
38.
Russo SJ, Mazei-Robison MS, Ables JL, Nestler EJ. Neurotrophic factors and structural plasticity in addiction. Neuropharmacolo. 2009;56:73-82. https://doi.org/10.1016/j.neuropharm.2008.06.059.
-
39.
Grimm JW, Lu L, Hayashi T, Hope BT, Su TP, Shaham Y. Time-dependent increases in brain-derived neurotrophic factor protein levels within the mesolimbic dopamine system after withdrawal from cocaine: implications for incubation of cocaine craving. J Neurosci. 2003;23(3):742-7. [PubMed ID: 12574402]. [PubMed Central ID: PMC6741929]. https://doi.org/10.1523/JNEUROSCI.23-03-00742.2003.
-
40.
Mehrafza S, Kermanshahi S, Mostafidi S, Motaghinejad M, Motevalian M, Fatima S. Pharmacological evidence for lithium-induced neuroprotection against methamphetamine-induced neurodegeneration via Akt-1/GSK3 and CREB-BDNF signaling pathways. Iran J Basic Med Sci. 2019;22(8):856-65. [PubMed ID: 31579440]. [PubMed Central ID: PMC6760490]. https://doi.org/10.22038/ijbms.2019.30855.7442.
-
41.
Carp JS, Anderson RJ. Dopamine receptormediated depression of spinal monosynaptic transmission. Brain Res. 1982;242:247-54. https://doi.org/10.1016/0006-8993(82)90307-9.
-
42.
Yokoo H, Yamada S, Yoshida M, Tanaka M, Nishi S. Attenuation of the inhibitory effect of dynorphin on dopamine release in the rat nucleus accumbens by repeated treatment with methamphetamine. Eur J Pharmacol. 1992;222(1):43-7. [PubMed ID: 1361440]. https://doi.org/10.1016/0014-2999(92)90461-c.
-
43.
Etaee F, Asadbegi M, Taslimi Z, Shahidi S, Sarihi A, Soleimani Asl S, et al. The effects of methamphetamine and buprenorphine, and their interaction on anxiety-like behavior and locomotion in male rats. Neurosci Lett. 2017;655:172-8. [PubMed ID: 28698151]. https://doi.org/10.1016/j.neulet.2017.04.043.
-
44.
Wu CC, Hung CJ, Shen CH, Chen WY, Chang CY, Pan HC, et al. Prenatal buprenorphine exposure decreases neurogenesis in rats. Toxicol Lett. 2014;225(1):92-101. [PubMed ID: 24321744]. https://doi.org/10.1016/j.toxlet.2013.12.001.
-
45.
Chiang YC, Hung TW, Ho IK. Development of sensitization to methamphetamine in offspring prenatally exposed to morphine, methadone and buprenorphine. Addict Biol. 2014;19(4):676-86. [PubMed ID: 23551991]. https://doi.org/10.1111/adb.12055.
-
46.
Motaghinejad M, Taheri P, Keshavarzi S, Ebadi M, Motevalian M. Neuroprotective Effects of Forced Exercise and Bupropion on Chronic Methamphetamine-induced Cognitive Impairment via Modulation of cAMP Response Element-binding Protein/Brain-derived Neurotrophic Factor Signaling Pathway, Oxidative Stress, and Inflammatory Biomarkers in Rats. Adv Biomed Res. 2018;7(1). https://doi.org/10.4103/abr.abr_11_18.
-
47.
Ren W, Tao J, Wei Y, Su H, Zhang J, Xie Y, et al. Time-Dependent Serum Brain-Derived Neurotrophic Factor Decline During Methamphetamine Withdrawal. Med. 2016;95(5). e2604. [PubMed ID: 26844469]. [PubMed Central ID: PMC4748886]. https://doi.org/10.1097/MD.0000000000002604.
-
48.
Li Y, Xia B, Li R, Yin D, Liang W. Changes in Expression of Dopamine, Its Receptor, and Transporter in Nucleus Accumbens of Heroin-Addicted Rats with Brain-Derived Neurotrophic Factor (BDNF) Overexpression. Med Sci Monit. 2017;23:2805-15. [PubMed ID: 28598964]. [PubMed Central ID: PMC5473376]. https://doi.org/10.12659/msm.904670.
-
49.
Pereira FC, Gough B, Macedo TR, Ribeiro CF, Ali SF, Binienda ZK. Buprenorphine modulates methamphetamine-induced dopamine dynamics in the rat caudate nucleus. Neurotox Res. 2011;19(1):94-101. [PubMed ID: 20033362]. https://doi.org/10.1007/s12640-009-9143-9.