Abstract
Background:
Recently, due to the numerous applications of silver nanoparticles (AgNPs) in industry, various routes to synthesize them have been developed.Objectives:
The current study was aimed at synthesizing silver nanoparticles by the leaf extract of Berberis vulgaris and evaluating the cytotoxic effects on human breast cancer MCF-7 cell line.Methods:
The leaf extract of Berberis vulgaris and silver nitrate solution were used to synthesize silver nanoparticles. Ultraviolet (UV)-visible, Fourier-transform infrared, and X-ray diffraction analysis spectroscopy and transmission electron microscopy methods were used to characterize and confirm the nanoparticles’ synthesis. The cytotoxic activity of synthesized nanoparticles (0, 5,10, 20, 40 µg/mL) was also studied by MTT assay.Results:
The results showed that Ag nanoparticles were polydisperse and spherical in shape and had a size of about 19.9 nm. Silver nanoparticles reduced the growth of cancerous cells based on time and concentration. The IC50 for MCF-7 cells at 48 hours was 20.27 µg/mL.Conclusions:
The findings showed that synthesized nanoparticles have an appropriate cytotoxic effect on cancer cells. This impact may be due to the production of free radicals through the release of Ag ions.Keywords
Berberis vulgaris Anticancer Activity Silver Nanoparticles Biosynthesis
1. Background
Today, the application of nanoparticles has generated a wide range of research due to their unique chemical, thermal, mechanical, and electrical properties rather than their massive materials. The high surface-to-volume ratio, the size of less than 100 nm and the ability to control the shape and size of nanoparticles have led to increased biological activity (1). Among metals, silver nanoparticle is one of the most important chemical elements due to its unique properties, including high electrical conductivity and antimicrobial activity. Currently, various physicochemical methods are used to synthesize nanoparticles for application in different fields, including medical, biological sensors, solar cells, textile and agricultural industries (2). Concerning the use of toxic chemicals, the complexity of the synthesis procedure, cost-intensiveness and the production of harmful wastes in these methods, their application in the biological and medicinal fields is limited (2, 3).
In the meantime, the use of plants due to biocompatibility and green and easy synthesis is one of the most reliable methods for producing nanoparticles. The presence of reducing and coating agents such as polysaccharides, amino acids, flavonoids, terpenes, enzymes and proteins in plant extracts can decrease metal ions and the production of nanoparticles with high stability and varied shapes and sizes (4, 5). Several reports have been published on the synthesis of silver nanoparticles using plant extracts such as Aloe vera, Mentha piperita, Ficus benghalensis and Catharanthus roseus (6-8).
One of the applications of silver nanoparticles is their use in the medical field as an antibacterial or as an anticancer agent. Due to the worldwide confirmation of the antibacterial effects of silver nanoparticles and the development of antibiotic resistance and the increase of infectious diseases, their application in industry and medicine is increasing today (9). Moreover, studies have shown that AgNPs exert their antibacterial activity through degradation of enzymes and breakage of DNA, enhancement of membrane permeability, and inactivation of cellular proteins (4, 10, 11). Research on the anticancer activity of silver nanoparticles is emerging and information limited and unclear. Therefore, studying the anticancer effects of AgNPs is critical.
Various lines of evidence have indicated the presence of phenolic compounds in Berberis vulgaris (12). These compounds such as berberine can act as reducing agents in the solution and cause the reduction of Ag+ ions to AgNPs. Accordingly, Berberis vulgaris is a great candidate for green synthesis of AgNPs.
2. Objectives
We used the extract of Berberis vulgaris in the synthesis of AgNPs. Cytotoxicity of the synthesized silver nanoparticles was evaluated on human breast cancer MCF-7 cell line.
3. Methods
3.1. Plant Material and Cell Line
Berberis vulgaris leaves were collected from Birjand in South Khorasan Province, Iran. The leaves were washed with distilled water and shade dried. Silver nitrate (AgNO3), nutrient broth (NB), nutrient agar (NA) medium, and gentamicin antibiotic were purchased from Merck. MCF-7 cell line was acquired from Pasteur Institute, Tehran, Iran. The cells were cultured and maintained according to the relevant protocols (13).
3.2. Biosynthesis of AgNPs
To make an aqueous extract, 20 g of dried leaves was powdered and mixed with 100 mL of distilled water at 60°C - 70°C for 1 hour. After cooling, the mixture was filtered and the final solution were kept at 4°C. To synthesize AgNPs, 5 ml of aqueous extract was added to an Erlenmeyer flask containing 45 mL of AgNO3 solution (1 mM) and placed at 25°C for 2 h in dark. After a few hours, the color of the solution was changed from yellow to brown. The resulting colloidal solution was centrifuged, and then the obtained deposit was washed frequently with absolute ethanol and deionized water. Finally, the samples were dried in an oven to get the powder (5).
3.3. Characterization of Silver Nanoparticles
The synthesized nanoparticles were characterized by ultraviolet-visible (UV-Vis) spectrum, X-ray diffraction (XRD), transition electron microscopy (TEM) and Fourier transform infrared (FT-IR). UV-Vis absorption spectrum of the synthesized NPs was recorded as a function of the reaction time on a UV-Vis spectrophotometer (T80, PG Instruments, UK) in the range of 300-600 nm at room temperature. The average crystalline size and nature of nanoparticles were obtained using an XRD pattern carried out on an STOE STADI MP diffractometer (Germany) with Cu Kα radiation (λ= 1.5406 Ǻ). The TEM measurements were performed by a transition electron microscope (Zeiss EM 10 C-100KV, Germany). Finally, FT-IR spectra were analyzed on a Bruker Tensor 27 spectrophotometer (Ettlingen, Germany) in the wavenumber range of 400 - 4000 cm-1.
3.4. Cytotoxicity Assay
MTT assay was used to determine the cytotoxic activity of the AgNPs. Cells were placed in 96 well plates and kept for 24 h at 37°C. Then, the cells were exposed to five concentrations of AgNPs (i.e., 0, 5,10, 20, and 40 µg/mL) and were incubated for 24, 48 and 72 h. After the addition of MTT solution to each well, the plate was incubated for 4 h. Then, 100 mL of dimethyl sulfoxide (DMSO) was added and optical density was determined at 540 nm using an ELISA microplate reader (Awareness, PalmCity, FL, USA).
3.5. Statistical Analysis
SAS 9.1 software was used to analyze the variances of the data related to the cytotoxicity effects, and GraphPad Prism 9 software was employed to depict the graphs.
4. Results and Discussion
4.1. Synthesis of AgNPs
In the present study, the leaf extract of Berberis vulgaris was used as a coating and reducing factor for AgNPs synthesis. As shown in Figure 1, by adding the AgNO3 solution (1 mM) to the Berberis vulgaris leaf extract, Ag ions reduced and led to a brownish color in solution within a few hours, which reveals the generation of AgNPs (14). The AgNO3 solution was used as a control sample. Leaf extract of Berberis vulgaris contains phenolics such as berberine can act as a reducing factor for nanoparticle synthesis (15). The alter in the color of the solution is because of the reduction of Ag+ ions by the extract of Berberis vulgaris and the excitement of surface plasmon resonance of silver nanoparticles (16).
Reduction of Ag ions to AgNPs in the presence of the leaf extract of Berberis vulgaris. Color changes from yellow to dark brown after 12 hours.
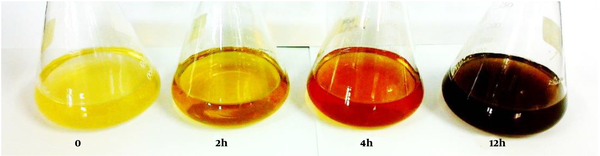
4.2. Characterizations of AgNPs
UV-vis spectra of AgNPs: The formation of AgNPs was monitored by UV-vis, XRD, TEM and FT-IR techniques. Metal nanoparticles such as silver formed the SPR absorption band because of the presence of free electrons. The Uv-vis spectra of the synthesized NPs revealed a broad peak at 439 nm, which is a characteristic of AgNPs (Figure 2) (14, 17). The broadening of the peak indicated that the nanoparticles were polydisperse (18). Generally, the synthesized AgNPs showed the absorbance peak from 400 to 480 nm (14). To prevent the agglomeration and formation of larger particles, an optimum time is required, as the color intensity did not change after 2 hours, we could be sure that the reaction was over.
UV/Vis absorbance spectra of synthesized AgNPs using Berberis vulgaris extract and AgNO3 solution (1 mM).
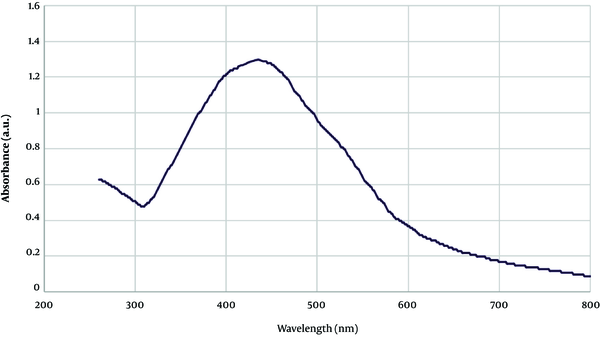
TEM Analysis of AgNPs: TEM images confirmed that the synthesized nanoparticles were polydisperse and spherical in shape with no aggregation. The particle size distribution histogram is shown in Figure 3, with an average size of 16 nm, a minimum of 8 nm, and a maximum of 27 nm. Many reports have been published on AgNPs synthesis with various sizes (19). Song and Kim (20) synthesized silver nanoparticles using the leaf extract of various plants through Ag+ reduction to Ag0 in the range of sizes of 15 nm - 500 nm.
Transition electron microscopy (TEM) images of synthesized AgNPs by the extract of Berberis vulgaris.
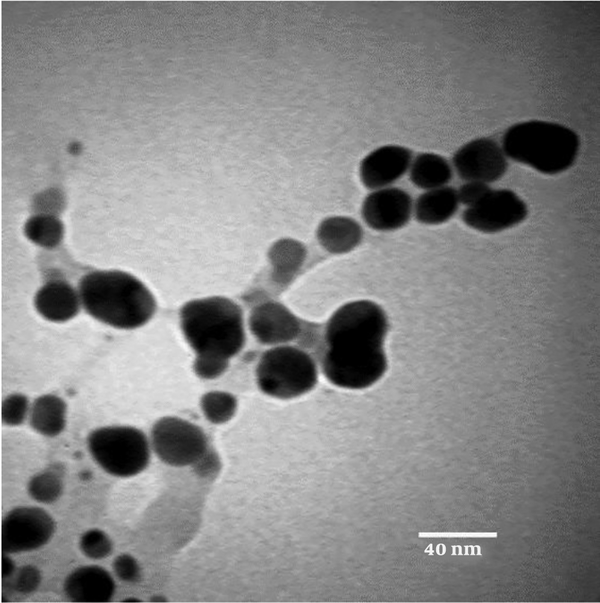
XRD Spectra of AgNPs: The crystallinity of synthesized nanoparticles was analyzed by XRD. The presence of diffraction peaks at 27.4°, 38.04°, 44.08°, 46.24°, 55,12°, 57.36°, 64.88°, and 78.16° in the 2θ range, which corresponded to the (2 1 0), (1 1 1), (2 0 0), (2 3 1), (1 4 2), (2 4 1), (2 2 0) and (3 1 1) planes of silver, respectively (Figure 4), proves that ANPs have a face-centered cubic crystal structure (13, 21). The presence of the unknown peaks can be related to the Berberis vulgaris extract, action as a reducing agent, and stabilizers for AgNPs (22).
X-ray diffraction (XRD) pattern of synthesized AgNPs by Berberis vulgaris extract
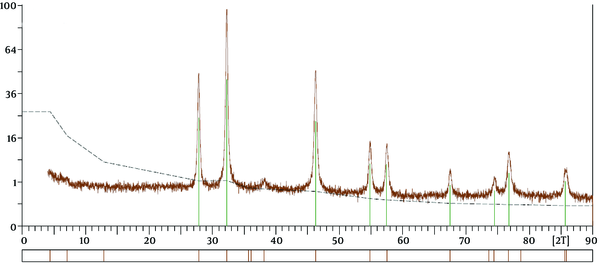
FTIR Analysis of AgNPs: FTIR spectroscopy was performed to identify the functional groups responsible for capping and coating agents for the synthesized AgNPs. As shown in Figure 5, the absorption bands at 825, 1059, 1451 and, 1549 cm-1 are associated with CH2-CH-CH3 aliphatic groups, the C-O groups of the polysaccharides, bending vibrations of O-H group and C-H (23). The band at 2921 cm-1 can be associated with the C-H stretching vibrations of methoxy, methylene, and methyl groups (14). The band at 1640 cm-1 corresponding to amide I led to bending vibration of the carbonyl group in the proteins and plays a central role in the coating of AgNPs (24). The broad peak at 3271 cm-1 can be associated with the stretching vibration of O-H group in alcohols and phenolics that result in the reduction of Ag ions to AgNPs.
Fourier transform infrared (FT-IR) spectrum of synthesized AgNPs by Berberis vulgaris extract
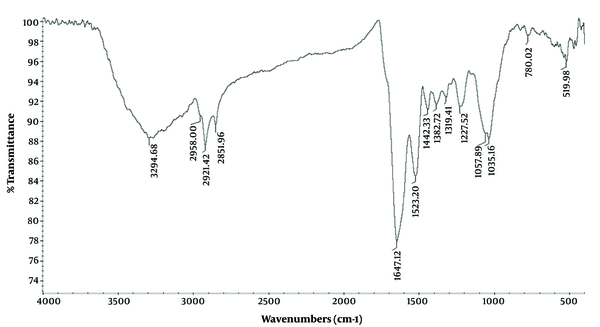
Cytotoxicity of AgNPS: MTT assay was conducted to evaluate the potential cytotoxic effect of AgNPS on breast MCF-7 cancer cell line. These cell lines were exposed to various doses of AgNPs for 24, 48, and 72 h. The results revealed that AgNPs significantly decreased the viability of malignant cells based on time and dose (P < 0.05). This cytotoxic effect was detected within a 24-h duration, which continued to extend up to the next 72 h. The survival rates of MCF-7 cells was approximately 76, 48 and 26% after exposure to 10, 20 and 40 µg/mL of AgNPs for 48 h, respectively (Figure 6). AgNPs IC50 values of MCF-7 cells were 20.27 µg/mL and 12 µg/mL for 48 and 72 h, respectively.
Cytotoxic effects of AgNPs on MCF-7 human breast cell lines. Cells were treated with different concentrations of AgNPs. Data are the mean ± SEM of three independent experiments. (*, P < 0.05).
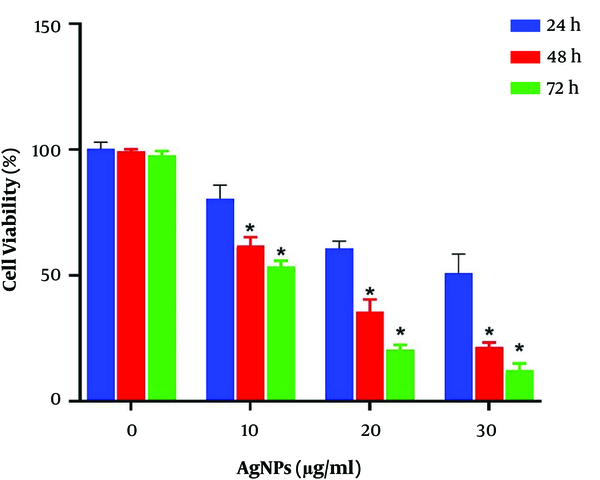
According to the results of this study, silver nanoparticles have a toxic effect on cancer cells, which can be due to the relatively acidic environment of tumor cells, which causes the fast release of silver ions from silver nanoparticles (25). Besides, the toxic effects of silver ions on cancer cells can be due to the reaction of silver atoms with domains of proteins, as well as nitrogenous bases and phosphate groups of DNA molecules (26). Several studies and evidence suggest the induction of apoptosis due to the use of silver nanoparticles in cancer cells (27-29). Silver nanoparticles seem to produce free radicals through releasing Ag ions. Free radicals with DNA damage and mitochondrial membrane destruction cause cytochrome c release and finally induce apoptosis from the mitochondrial pathway (30).
References
-
1.
Gupta R. Fundamentals of Drug Nanoparticles. Nanoparticle Technology for Drug Delivery. CRC Press; 2006. p. 25-44. https://doi.org/10.1201/9780849374555.ch1.
-
2.
Zhang XF, Liu ZG, Shen W, Gurunathan S. Silver Nanoparticles: Synthesis, Characterization, Properties, Applications, and Therapeutic Approaches. Int J Mol Sci. 2016;17(9). [PubMed ID: 27649147]. [PubMed Central ID: PMC5037809]. https://doi.org/10.3390/ijms17091534.
-
3.
Iravani S, Korbekandi H, Mirmohammadi SV, Zolfaghari B. Synthesis of silver nanoparticles: chemical, physical and biological methods. Res Pharm Sci. 2014;9(6):385-406. [PubMed ID: 26339255]. [PubMed Central ID: PMC4326978].
-
4.
Mittal AK, Chisti Y, Banerjee UC. Synthesis of metallic nanoparticles using plant extracts. Biotechnol Adv. 2013;31(2):346-56. [PubMed ID: 23318667]. https://doi.org/10.1016/j.biotechadv.2013.01.003.
-
5.
Kuppusamy P, Yusoff MM, Maniam GP, Govindan N. Biosynthesis of metallic nanoparticles using plant derivatives and their new avenues in pharmacological applications - An updated report. Saudi Pharm J. 2016;24(4):473-84. [PubMed ID: 27330378]. [PubMed Central ID: PMC4908060]. https://doi.org/10.1016/j.jsps.2014.11.013.
-
6.
MubarakAli D, Thajuddin N, Jeganathan K, Gunasekaran M. Plant extract mediated synthesis of silver and gold nanoparticles and its antibacterial activity against clinically isolated pathogens. Colloids Surf B Biointerfaces. 2011;85(2):360-5. [PubMed ID: 21466948]. https://doi.org/10.1016/j.colsurfb.2011.03.009.
-
7.
Saxena A, Tripathi RM, Zafar F, Singh P. Green synthesis of silver nanoparticles using aqueous solution of Ficus benghalensis leaf extract and characterization of their antibacterial activity. Mater Lett. 2012;67(1):91-4. https://doi.org/10.1016/j.matlet.2011.09.038.
-
8.
Rajagopal T, Jemimah IA, Ponmanickam P, Ayyanar M. Synthesis of silver nanoparticles using Catharanthus roseus root extract and its larvicidal effects. J Environ Biol. 2015;36(6):1283-9. [PubMed ID: 26688962].
-
9.
Byarugaba DK. Mechanisms of Antimicrobial Resistance. Antimicrobial Resistance in Developing Countries. Springer; 2010. p. 15-26. https://doi.org/10.1007/978-0-387-89370-9_2.
-
10.
Prabhu S, Poulose EK. Silver nanoparticles: mechanism of antimicrobial action, synthesis, medical applications, and toxicity effects. Int Nano Lett. 2012;2(1). https://doi.org/10.1186/2228-5326-2-32.
-
11.
Guzman M, Dille J, Godet S. Synthesis and antibacterial activity of silver nanoparticles against gram-positive and gram-negative bacteria. Nanomedicine. 2012;8(1):37-45. [PubMed ID: 21703988]. https://doi.org/10.1016/j.nano.2011.05.007.
-
12.
Imenshahidi M, Hosseinzadeh H. Berberis Vulgaris and Berberine: An Update Review. Phytother Res. 2016;30(11):1745-64. [PubMed ID: 27528198]. https://doi.org/10.1002/ptr.5693.
-
13.
Li J, Tian B, Li T, Dai S, Weng Y, Lu J, et al. Biosynthesis of Au, Ag and Au-Ag bimetallic nanoparticles using protein extracts of Deinococcus radiodurans and evaluation of their cytotoxicity. Int J Nanomedicine. 2018;13:1411-24. [PubMed ID: 29563796]. [PubMed Central ID: PMC5849937]. https://doi.org/10.2147/IJN.S149079.
-
14.
Patra JK, Baek KH. Antibacterial Activity and Synergistic Antibacterial Potential of Biosynthesized Silver Nanoparticles against Foodborne Pathogenic Bacteria along with its Anticandidal and Antioxidant Effects. Front Microbiol. 2017;8:167. [PubMed ID: 28261161]. [PubMed Central ID: PMC5309230]. https://doi.org/10.3389/fmicb.2017.00167.
-
15.
Vaya J, Mahmood S. Flavonoid content in leaf extracts of the fig (Ficus carica L.), carob (Ceratonia siliqua L.) and pistachio (Pistacia lentiscus L.). Biofactors. 2006;28(3-4):169-75. [PubMed ID: 17473377]. https://doi.org/10.1002/biof.5520280303.
-
16.
Mulvaney P. Surface Plasmon Spectroscopy of Nanosized Metal Particles. Langmuir. 1996;12(3):788-800. https://doi.org/10.1021/la9502711.
-
17.
Nazeruddin GM, Prasad NR, Prasad SR, Shaikh YI, Waghmare SR, Adhyapak P. Coriandrum sativum seed extract assisted in situ green synthesis of silver nanoparticle and its anti-microbial activity. Ind Crops Prod. 2014;60:212-6. https://doi.org/10.1016/j.indcrop.2014.05.040.
-
18.
Aziz N, Fatma T, Varma A, Prasad R. Biogenic Synthesis of Silver Nanoparticles Using Scenedesmus abundans and Evaluation of Their Antibacterial Activity. J Nanopart. 2014;2014:1-6. https://doi.org/10.1155/2014/689419.
-
19.
Jain S, Mehata MS. Medicinal Plant Leaf Extract and Pure Flavonoid Mediated Green Synthesis of Silver Nanoparticles and their Enhanced Antibacterial Property. Sci Rep. 2017;7(1):15867. [PubMed ID: 29158537]. [PubMed Central ID: PMC5696514]. https://doi.org/10.1038/s41598-017-15724-8.
-
20.
Song JY, Kim BS. Rapid biological synthesis of silver nanoparticles using plant leaf extracts. Bioprocess Biosyst Eng. 2009;32(1):79-84. [PubMed ID: 18438688]. https://doi.org/10.1007/s00449-008-0224-6.
-
21.
Jyoti K, Baunthiyal M, Singh A. Characterization of silver nanoparticles synthesized using Urtica dioica Linn. leaves and their synergistic effects with antibiotics. J Radiat Res Appl Sci. 2019;9(3):217-27. https://doi.org/10.1016/j.jrras.2015.10.002.
-
22.
Sathiya CK, Akilandeswari S. Fabrication and characterization of silver nanoparticles using Delonix elata leaf broth. Spectrochim Acta A Mol Biomol Spectrosc. 2014;128:337-41. [PubMed ID: 24681317]. https://doi.org/10.1016/j.saa.2014.02.172.
-
23.
Erjaee H, Rajaian H, Nazifi S. Synthesis and characterization of novel silver nanoparticles using Chamaemelum nobile extract for antibacterial application. Adv Nat Sci. 2017;8(2). https://doi.org/10.1088/2043-6254/aa690b.
-
24.
Rao B, Tang R. Green synthesis of silver nanoparticles with antibacterial activities using aqueous Eriobotrya japonica leaf extract. Adv Nat Sci. 2017;8(1). https://doi.org/10.1088/2043-6254/aa5983.
-
25.
Sweeney SK, Luo Y, O'Donnell MA, Assouline J. Nanotechnology and cancer: improving real-time monitoring and staging of bladder cancer with multimodal mesoporous silica nanoparticles. Cancer Nanotechnol. 2016;7:3. [PubMed ID: 27217840]. [PubMed Central ID: PMC4846680]. https://doi.org/10.1186/s12645-016-0015-8.
-
26.
Zhang T, Wang L, Chen Q, Chen C. Cytotoxic potential of silver nanoparticles. Yonsei Med J. 2014;55(2):283-91. [PubMed ID: 24532494]. [PubMed Central ID: PMC3936614]. https://doi.org/10.3349/ymj.2014.55.2.283.
-
27.
Poulose S, Panda T, Nair PP, Theodore T. Biosynthesis of silver nanoparticles. J Nanosci Nanotechnol. 2014;14(2):2038-49. [PubMed ID: 24749472]. https://doi.org/10.1166/jnn.2014.9019.
-
28.
Bin-Jumah M, Al-Abdan M, Albasher G, Alarifi S. Effects of Green Silver Nanoparticles on Apoptosis and Oxidative Stress in Normal and Cancerous Human Hepatic Cells in vitro. Int J Nanomedicine. 2020;15:1537-48. [PubMed ID: 32210550]. [PubMed Central ID: PMC7074819]. https://doi.org/10.2147/IJN.S239861.
-
29.
Raj RK, D E, S R. beta-Sitosterol-assisted silver nanoparticles activates Nrf2 and triggers mitochondrial apoptosis via oxidative stress in human hepatocellular cancer cell line. J Biomed Mater Res A. 2020;108(9):1899-908. [PubMed ID: 32319188]. https://doi.org/10.1002/jbm.a.36953.
-
30.
Stojnev S, Ristic-Petrovic A, Jankovic-Velickovic L. Reactive oxygen species, apoptosis and cancer. Vojnosanit Pregl. 2013;70(7):675-8. [PubMed ID: 23984617]. https://doi.org/10.2298/vsp1307675s.