Abstract
Background:
Colorectal cancer (CRC) is one of the most common gastrointestinal cancers worldwide. It is the second leading cause of death in women after breast cancer.Objectives:
The present study aimed to determine the cytotoxic effects of zinc oxide (ZnO) nanoparticles on colon cancer (SW480) viability and nitric oxide (NO) level in vitro.Methods:
In this experimental study, the values of 15.62, 31.25, 62.5, 125, 250, and 500 μg/mL of ZnO nanoparticles were considered to treat SW480 cells for 24 hours. The MTT method was used to determine cell viability. The amount of NO was also measured using the grease method. An analysis of variance (ANOVA) was used to analyze the data.Results:
Treatment of SW480 cells with ZnO nanoparticles resulted in decreased viability in a dose-dependent pattern. NO concentration significantly increased in response to an effective dose of ZnO nanoparticles (108 µg/mL) compared to the control group (P < 0.001).Conclusions:
ZnO nanoparticles have cytotoxic effects on colon cancer cells. The cytotoxic effect of ZnO nanoparticles on colon cancer cells is partly mediated by NO in cancer cells.Keywords
1. Background
Colorectal cancer (CRC) is the second and third most common cancer among women and men, respectively; it includes almost 10% of all cancer cases (1). CRC is an iatrogenic disease with different pathogenic mechanisms and closely relates to environmental factors, genetic factors, diet, and lifestyle (2). Intestinal polyp generally causes no clinical signs in the body, although they may turn into CRC over time. The signs of CRC are hematochezia, sudden weight loss, iron deficiency anemia, stomachache, and unknown and persistent hypogastric pain (3).
In recent years, scientists have sought to control cancer in different ways, such as chemotherapy, radiotherapy, and surgical interventions, all of which are effective in cancer therapy; however, they can cause damage to normal body cells due to their undesirable and non-selective effects of individual’s normal cells. Today, the development of medical nanotechnology, targeted drug delivery, and multipurpose inhibitors leads to novel therapeutic methods for inhibiting cancer cell proliferation (4). Nanoparticles are named as particles of dimensions 1 to 100 nm and exhibit different properties on the nanoscale (5). Nanoparticles with the capability of directed selection (selective cytotoxicity), increased effectiveness, and reduced side effects on normal cells become more vital in medical sciences. Every nanoparticle has unique properties, which turn it into a novel and effective tool for cancer treatment (6). Previous studies have revealed that nanoparticles can be employed as a proper approach to treat different types of cancer, including liver (7), lung (8), prostate (9), gastrointestinal (10), and breast cancers (11). Nanoparticles are highly soluble due to their small size (5, 12). Some studies indicate that nanoparticles of diameters lower than 10 nm aggregate effectively and penetrate to the deeper parts of the tumor. However, other studies show that nanoparticles smaller than 10 nm in diameter lead to a broad range of toxicity-related issues in normal cells (13). There are many nonorganic mineral nanoparticles such as zinc oxide (ZnO), cerium oxide, titanium dioxide, copper oxide, silica oxide, iron oxide, and other nanoparticles that have strong anticancer properties (14). ZnO has unique properties, such as high selectivity, biocompatibility, easy synthesis, and superior capability in oxidative stress induction. Thus, it is an important tool in the modern treatment of cancer and overshadows the conventional therapeutic methods as a promising anticancer factor (15). Studies have suggested that nanoparticles can affect different types of cancer, such as breast, gastrointestinal, and colorectal cancers (16-19). According to a study, ZnO nanoparticles, by the intervention of nitric oxide (NO) mediator, create the maximum inflammatory response in cells, which allows reactive oxygen species (ROS) to have the highest intracellular production. This increase in ROS levels significantly damages the DNA of cancer cells, stops the cell cycle, and finally leads to apoptosis and cell death (20). Although studies have shown that ZnO nanoparticles have significant anticancer effects on colon cancer cells, its mechanism of action is not yet clear.
2. Objectives
The aim of this study was to investigate the cytotoxicity effects of ZnO nanoparticles on the viability of SW480 cell lines and NO level in the culture medium to determine whether NO is mediated in the ZnO effect on SW480 cells.
3. Methods
The SW480 cell lines were purchased from Pasteur Institute Cell Bank, Tehran, Iran. The SW480 cells were cultured in Dulbecco’s Modified Eagle’s Medium supplemented with 10% fetal bovine serum (FBS; Gibco, New York, NY, USA). Streptomycin and penicillin (each 100 μg/mL) were poured into T-25 tissue culture flasks and placed in an incubator under 5% CO2, and 95% humidified air at 37°C. The environment was changed every 2 - 3 days (21). ZnO nanoparticles (99% purity, 10 - 30 nm, nearly spherical particles, and 50 g net weight) were obtained from US Research Nanomaterial, Inc. Figure 1 shows a transmission electron microscopy (TEM) image of a zinc nanoparticle (Figure 1). All chemicals and solvents were of analytical grade and produced by Merck (Darmstadt, Germany) or Sigma-Aldrich (St. Louis, USA) companies. This study was approved by the Ethics Committee of Hamedan Azad University.
Transmission electron microscopy image of colloidal zinc oxide nanoparticles
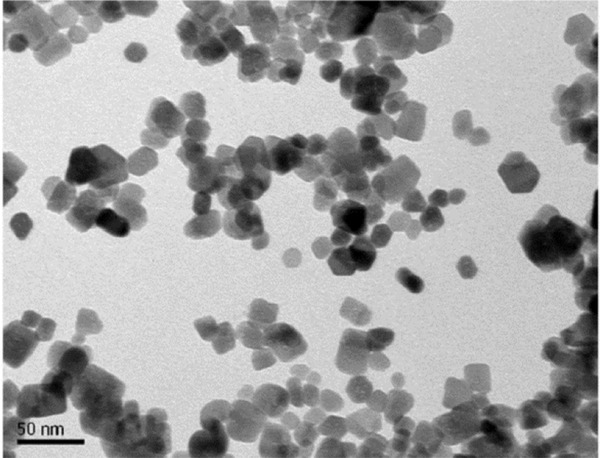
3.1. MTT
Cell viability was measured by the MTT assay. SW480 cells (5 × 103 cells/well) were divided into 96-well plates and cultured for 24 hours. Different concentrations (15.62, 31.25, 62.5, 125, 250, and 500 µg/mL) of ZnO nanoparticles were prepared in sterile micro-tubes. The cells were treated with ZnO nanoparticles in the treatment group and with the same volume of culture medium in the control group. Twenty-four hours after treatment, the MTT colorimetric method was used to evaluate the cytotoxicity of ZnO nanoparticles. Therefore, 100 μL of culture medium without calf embryo serum and 10 μL of MTT (5 mg/mL in PBS) were replaced with the previous culture medium in each well, and the plates were re-incubated. After 4 hours, the culture medium was removed, and the cells were lysed with 200 μL of DMSO. The formazan product was dissolved, and the absorbance was measured at 570 nm through an ELISA reader (Stat Fax-2100 Awareness, Mountain View, CA, USA). Relative viability was defined as the ratio of the treated sample to the control group absorbance (22, 23). Cell viability was determined using the following formula:
3.2. NO Measurement
To measure the NO amount in the culture medium, after treatment of SW40 cells with a 50% inhibitory concentration (IC50) dose of ZnO nanoparticles, the supernatant solution was collected, and NO concentration was measured using the Griess method that can detect the presence of nitrite ions in the solution (24). The Griess method involves a 2-step diazotization reaction. In this method, a NO-derived nitrosating agent (as well as dinitrogen trioxide [N2O3], which is formed from nitrous acid catalyzed by nitrite acid) reacts with a sulfonamide to produce a diazonium ion. This ion binds to N-(1-Naphthyl) ethylenediamine dihydrochloride (NED) to form a chromophoric azo product that absorbs at 540 nm. To measure NO levels, 50 µL of the supernatant solution was added to wells. Then, 50 µL of sulfonamide was added to samples within wells, and dilution and incubation were performed for 5 to 10 minutes. Next, 50 µL of NED was added to samples within wells, and incubation was conducted again for 10 minutes. Finally, the absorption was determined at 540 nm.
3.3. Data Analysis
A linear regression method was used to calculate IC50 (25). All statistical analyses were performed using SPSS version 19 (SPSS Inc, Chicago, Ill, USA). The Kolmogorov-Smirnov test was used to normalize the data. Data were analyzed using an analysis of variance (ANOVA). P values less than 0.05 were considered statistically significant.
3.4. Statistical Analysis
SPSS version 19 (SPSS Inc, Chicago, Ill, USA) was used for statistical analysis. The normality of the studied variables was evaluated by the Kolmogorov-Smirnov test. Continuous variables are presented as means and SDs, and classified variables are presented as numbers (percentages). Then, due to the dependence of the groups, a repeated-measures test was used to examine the 3 groups, and the corresponding post hoc tests were used for significant variables. A paired t-test was also used to evaluate the 2 groups. A P-value less than 0.05 was statistically significant.
4. Results
To measure IC50, the survival percentage of cells (Y-axis) against different concentrations of nanoparticles (X-axis) was plotted using a linear regression method (Figure 2), and then the IC50 value was estimated based on the associated line (25). To analyze the data, The Kolmogorov-Smirnov test was used to normalize the data.
IC50 dose calculation
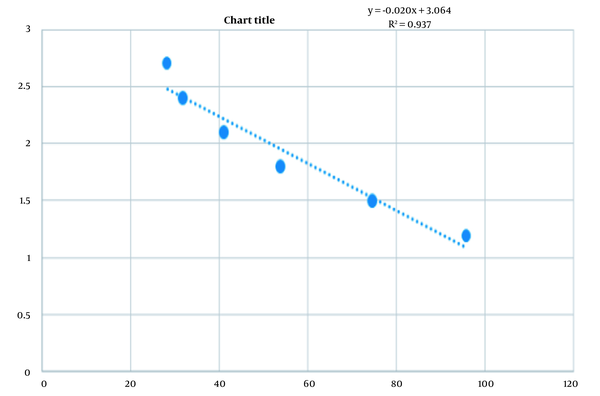
4.1. Physical and Microscopic Characterization of the Prepared ZnO Nanoparticles
The physicochemical properties of ZnO nanoparticles are known to govern their interaction with biological systems; hence, the study was started by characterizing ZnO nanoparticles. In the present study, the commercially available ZnO nanoparticles were used. Gray ZnO nanoparticle powder was prepared from zinc metal by an evaporation process and had a purity of 99.9%, an approximate concentration of 0.2 - 0.4 g/m3, and a specific surface area of 0.2 - 0.4 g/m3. The zinc particles produced during the evaporation process are of high purity, very small, and very reactive. The size and shape of the zinc particles were determined by TEM. Zinc nanoparticles were spherical with a size of 10 - 30 nm.
4.2. Cell Viability Assay After Exposure to ZnO Nanoparticles
According to the MTT assay, there were no significant differences between the viability of cells treated with a 15.62 µg/mL dose of ZnO nanoparticles and the control group. However, there were more viable cells in the samples treated with a 31.25 µg/mL dose of ZnO nanoparticles compared to the control group (P < 0.01). Significant cell viability was observed in cells treated with 62.5, 125, 250, and 500 μg/mL of ZnO nanoparticles compared to the control group (P < 0.001; Figure 3).
Cell viability of SW480 cells treated with different concentrations of zinc oxide nanoparticles. NS indicates no significant difference compared to the control group. * Shows meaningful difference compared to the control group (** P < 0.01 and *** P < 0.001).
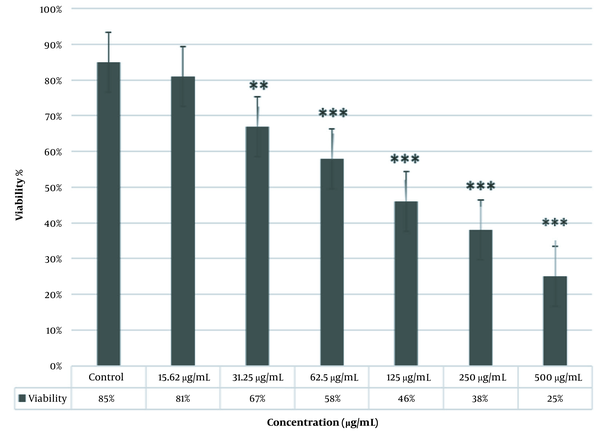
4.3. NO Production in SW480 Cells Treated with ZnO Nanoparticles
The results of the MTT test showed that IC50 was 108 µg/mL. NO levels of SW480 cells treated with an IC50 dose of ZnO nanoparticles significantly increased compared to the control group (P < 0.001; Figure 4).
Nitrite oxide levels in the culture medium of SW40 cells treated with an IC50 dose (treatment group) of ZnO nanoparticles compared to the control group (*** P < 0.001).
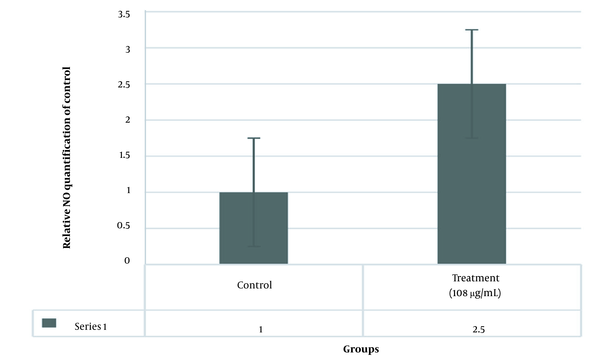
5. Discussion
Numerous studies have evaluated ZnO nanoparticle effects on different cell lines, and the results of the present study are in line with previous studies. Selvakumari et al. studied the antitumor effect of ZnO nanoparticles on the MCF-7 cell line in breast cancer; they indicated that 31 µg of this nanoparticle killed 50% of tumor cells (26). In another study, it was indicated that ZnO nanoparticles had an impressive antitumor effect on the tumor cells of glioma (T98G), and in the meantime, no cytotoxicity has been observed in HEK293 cells. Moreover, it was shown that ZnO nanoparticles with a genotoxicity effect induce apoptosis in these tumor cells. Also, the results of more detailed studies suggested that ZnO nanoparticles affect the induction of apoptosis through activation of the ROS pathway. Wahab et al treated the HepG2 liver cancer cell line and MCF-7 breast cancer cell line with ZnO nanoparticles. Their results showed that 25 µg/mL of this nanoparticle induced apoptosis, and the percentage of viable cells decreased to lower than 10%, and 70 µg/mL of ZnO nanoparticles could decrease the viability of SW40 cells by 50% (27). ZnO nanoparticles can be absorbed through the respiratory system and gastrointestinal wall. These nanoparticles can activate the ROS path of producing free oxygen radicals and eliminate tumor cells (28). Previous studies have indicated that the inclusion of ZnO nanoparticles over tumor cells causes the activation of an isoform of NO (iNOS) synthesis enzyme and production of NO; this also leads to the production of interleukin 1 (IL-1), interferons, and tumor necrosis factor (TNF) (29). All 3 factors cause inflammation; they stimulate the iNOS enzyme activity after entering the tumor cells, create positive feedback, and produce more NO. The NO produced by cells goes through 2 paths; in the first path, it provides the apoptosis of cells affecting cell DNA and, then, leads to deeper penetration of ZnO nanoparticles and more damage to the cells by the membrane lipid peroxidation (30). NO also can decrease the proliferation and strength of lymphocytes, which is responsible for creating the anti-inflammatory response, prohibits the formation of anti-inflammatory factors, and augments the apoptosis signals; thus, NO causes more damage to tumor tissue and stops the activity of anti-inflammatory lymphocytes (28).
5.1. Conclusions
ZnO nanoparticles have cytotoxic effects on colon cancer cells. The cytotoxic effect of ZnO nanoparticles on colon cancer cells is partly mediated by NO in cancer cells. Overall, ZnO nanoparticles have the potential to prevent the growth of colorectal tumor cells.
References
-
1.
Tariq K, Ghias K. Colorectal cancer carcinogenesis: a review of mechanisms. Cancer Biol Med. 2016;13(1):120-35. [PubMed ID: 27144067]. [PubMed Central ID: PMC4850121]. https://doi.org/10.28092/j.issn.2095-3941.2015.0103.
-
2.
Nguyen LH, Goel A, Chung DC. Pathways of Colorectal Carcinogenesis. Gastroenterology. 2020;158(2):291-302. [PubMed ID: 31622622]. [PubMed Central ID: PMC6981255]. https://doi.org/10.1053/j.gastro.2019.08.059.
-
3.
Faghfoori MH, Nosrati H, Rezaeejam H, Charmi J, Kaboli S, Johari B, et al. Anticancer effect of X-Ray triggered methotrexate conjugated albumin coated bismuth sulfide nanoparticles on SW480 colon cancer cell line. Int J Pharm. 2020;582:119320. [PubMed ID: 32278720]. https://doi.org/10.1016/j.ijpharm.2020.119320.
-
4.
Jurj A, Braicu C, Pop LA, Tomuleasa C, Gherman CD, Berindan-Neagoe I. The new era of nanotechnology, an alternative to change cancer treatment. Drug Des Devel Ther. 2017;11:2871-90. [PubMed ID: 29033548]. [PubMed Central ID: PMC5628667]. https://doi.org/10.2147/DDDT.S142337.
-
5.
Wang R, Billone PS, Mullett WM. Nanomedicine in Action: An Overview of Cancer Nanomedicine on the Market and in Clinical Trials. J Nanomater. 2013;2013:629681. https://doi.org/10.1155/2013/629681.
-
6.
Bisht G, Rayamajhi S. ZnO Nanoparticles: A Promising Anticancer Agent. Nanobiomedicine (Rij). 2016;3:9. [PubMed ID: 29942384]. [PubMed Central ID: PMC5998263]. https://doi.org/10.5772/63437.
-
7.
Yao Y, Zang Y, Qu J, Tang M, Zhang T. The Toxicity Of Metallic Nanoparticles On Liver: The Subcellular Damages, Mechanisms, And Outcomes. Int J Nanomedicine. 2019;14:8787-804. [PubMed ID: 31806972]. [PubMed Central ID: PMC6844216]. https://doi.org/10.2147/IJN.S212907.
-
8.
Mottaghitalab F, Farokhi M, Fatahi Y, Atyabi F, Dinarvand R. New insights into designing hybrid nanoparticles for lung cancer: Diagnosis and treatment. J Control Release. 2019;295:250-67. [PubMed ID: 30639691]. https://doi.org/10.1016/j.jconrel.2019.01.009.
-
9.
Malugin A, Ghandehari H; Arnida. Cellular uptake and toxicity of gold nanoparticles in prostate cancer cells: a comparative study of rods and spheres. J Appl Toxicol. 2010;30(3):212-7. [PubMed ID: 19902477]. https://doi.org/10.1002/jat.1486.
-
10.
Jefremow A, Neurath MF. Nanoparticles in Gastrooncology. Visc Med. 2020;36(2):88-94. [PubMed ID: 32355665]. [PubMed Central ID: PMC7184848]. https://doi.org/10.1159/000506908.
-
11.
Divakaran D, Lakkakula JR, Thakur M, Kumawat MK, Srivastava R. Dragon fruit extract capped gold nanoparticles: Synthesis and their differential cytotoxicity effect on breast cancer cells. Mater Lett. 2019;236:498-502. https://doi.org/10.1016/j.matlet.2018.10.156.
-
12.
McNeil SE. Nanoparticle therapeutics: a personal perspective. Wiley Interdiscip Rev Nanomed Nanobiotechnol. 2009;1(3):264-71. [PubMed ID: 20049796]. https://doi.org/10.1002/wnan.6.
-
13.
Huang K, Ma H, Liu J, Huo S, Kumar A, Wei T, et al. Size-dependent localization and penetration of ultrasmall gold nanoparticles in cancer cells, multicellular spheroids, and tumors in vivo. ACS Nano. 2012;6(5):4483-93. [PubMed ID: 22540892]. [PubMed Central ID: PMC3370420]. https://doi.org/10.1021/nn301282m.
-
14.
Vinardell MP, Mitjans M. Antitumor Activities of Metal Oxide Nanoparticles. Nanomaterials (Basel). 2015;5(2):1004-21. [PubMed ID: 28347048]. [PubMed Central ID: PMC5312892]. https://doi.org/10.3390/nano5021004.
-
15.
Mishra PK, Mishra H, Ekielski A, Talegaonkar S, Vaidya B. Zinc oxide nanoparticles: a promising nanomaterial for biomedical applications. Drug Discov Today. 2017;22(12):1825-34. [PubMed ID: 28847758]. https://doi.org/10.1016/j.drudis.2017.08.006.
-
16.
Farasat M, Niazvand F, Khorsandi L. Zinc oxide nanoparticles induce necroptosis and inhibit autophagy in MCF-7 human breast cancer cells. Biologia. 2020;75(1):161-74. https://doi.org/10.2478/s11756-019-00325-9.
-
17.
Tang Q, Xia H, Liang W, Huo X, Wei X. Synthesis and characterization of zinc oxide nanoparticles from Morus nigra and its anticancer activity of AGS gastric cancer cells. J Photochem Photobiol B. 2020;202:111698. [PubMed ID: 31734436]. https://doi.org/10.1016/j.jphotobiol.2019.111698.
-
18.
Condello M, De Berardis B, Ammendolia MG, Barone F, Condello G, Degan P, et al. ZnO nanoparticle tracking from uptake to genotoxic damage in human colon carcinoma cells. Toxicol In Vitro. 2016;35:169-79. [PubMed ID: 27317967]. https://doi.org/10.1016/j.tiv.2016.06.005.
-
19.
Moos PJ, Chung K, Woessner D, Honeggar M, Cutler NS, Veranth JM. ZnO particulate matter requires cell contact for toxicity in human colon cancer cells. Chem Res Toxicol. 2010;23(4):733-9. [PubMed ID: 20155942]. https://doi.org/10.1021/tx900203v.
-
20.
Setyawati MI, Tay CY, Leong DT. Mechanistic Investigation of the Biological Effects of SiO2, TiO2, and ZnO Nanoparticles on Intestinal Cells. Small. 2015;11(28):3458-68. [PubMed ID: 25902938]. https://doi.org/10.1002/smll.201403232.
-
21.
Astaneh M, Ghafouri-Fard S, Fazeli Z, Taherian-Esfahani Z, Dashti S, Motevaseli E. Assessment of anti-cancer effects of koenimbine on colon cancer cells. Hum Antibodies. 2020;28(3):185-90. [PubMed ID: 32116245]. https://doi.org/10.3233/HAB-200405.
-
22.
Chang KH, Park JS, Choi JH, Kim CJ, Paik HD. Cytotoxic effects of partially purified substances from Bacillus polyfermenticus SCD supernatant toward a Varity of tumor cell lines. Food Sci Biotechnol. 2007;16(1):163-6.
-
23.
Kim JY, Woo HJ, Kim YS, Kim KH, Lee HJ. Cell cycle dysregulation induced by cytoplasm of Lactococcus lactis ssp lactis in SNUC2A, a colon cancer cell line. Nutr Cancer. 2003;46(2):197-201. [PubMed ID: 14690796]. https://doi.org/10.1207/S15327914NC4602_13.
-
24.
Daniel WL, Han MS, Lee JS, Mirkin CA. Colorimetric nitrite and nitrate detection with gold nanoparticle probes and kinetic end points. J Am Chem Soc. 2009;131(18):6362-3. [PubMed ID: 19368386]. https://doi.org/10.1021/ja901609k.
-
25.
Chen YC, Tsai TL, Ye XH, Lin TH. Anti-proliferative effect on a colon adenocarcinoma cell line exerted by a membrane disrupting antimicrobial peptide KL15. Cancer Biol Ther. 2015;16(8):1172-83. [PubMed ID: 26147829]. [PubMed Central ID: PMC4622422]. https://doi.org/10.1080/15384047.2015.1056407.
-
26.
Selvakumari D, Deepa R, Mahalakshmi V, Subhashini P, Lakshminarayan N. Anti cancer activity of ZnO nanoparticles on MCF7 (breast cancer cell) and A549 (lung cancer cell). ARPN J Eng Appl Sci. 2015;10(12):5418-21.
-
27.
Wahab R, Dwivedi S, Umar A, Singh S, Hwang IH, Shin HS, et al. ZnO nanoparticles induce oxidative stress in Cloudman S91 melanoma cancer cells. J Biomed Nanotechnol. 2013;9(3):441-9. [PubMed ID: 23621000]. https://doi.org/10.1166/jbn.2013.1593.
-
28.
Djurisic AB, Leung YH, Ng AM, Xu XY, Lee PK, Degger N, et al. Toxicity of metal oxide nanoparticles: mechanisms, characterization, and avoiding experimental artefacts. Small. 2015;11(1):26-44. [PubMed ID: 25303765]. https://doi.org/10.1002/smll.201303947.
-
29.
Dinarello CA, Cannon JG, Wolff SM, Bernheim HA, Beutler B, Cerami A, et al. Tumor necrosis factor (cachectin) is an endogenous pyrogen and induces production of interleukin 1. J Exp Med. 1986;163(6):1433-50. [PubMed ID: 3486936]. [PubMed Central ID: PMC2188124]. https://doi.org/10.1084/jem.163.6.1433.
-
30.
Lu H, Ouyang W, Huang C. Inflammation, a key event in cancer development. Mol Cancer Res. 2006;4(4):221-33. [PubMed ID: 16603636]. https://doi.org/10.1158/1541-7786.MCR-05-0261.