Abstract
Background:
Natural products such as Allium jesdianum (AJ) have pharmacological properties with negligible side effects. However, their therapeutic potential has been limited by their low bioavailability. Nanocarriers improve the bioavailability and stability of flavonoids as the most common polyphenolic antioxidant.Objectives:
This study aimed to assess the toxic effects of Allium jesdianum extract (AJE) loaded in microemulsion (AJE-ME) on the HT-29 cells, a human colon cancer cell line.Methods:
HT-29 cells were exposed to 50 µM/mL of AJE or AJE-ME for 24 h. Colony formation, cell viability percentage, flow cytometry, and gene expression studies were carried out to assess the impacts of the AJE-ME.Results:
The AJE-ME with the required characteristics and a slow-release AJE were prepared. AJE-ME significantly diminished the survival percentage and colony formation of HT-29 cells compared to the free AJE. Upregulation of mTOR and downregulation of Beclin1 and Atg5 indicated suppression of autophagy by the AJE-ME. Flow cytometry results showed that AJE-ME significantly increased the percentage of necrosis in the HT-29 cells. AJE-ME upregulated the expression of necroptosis-related genes such as receptor-interacting protein kinase 3 (RIP3) and mixed lineage kinase domain-like pseudokinase (MLKL).Conclusions:
These data collectively demonstrated that ME enhanced the toxic effect of AJE against human colon cancer cells by suppressing autophagy and activating necroptosis.Keywords
Apoptosis Necroptosis Autophagy Microemulsion Colon Cancer Allium jesdianum
1. Background
Colorectal cancer (CRC), the second leading cause of cancer death worldwide, was responsible for nearly 881000 deaths in 2018. Despite standard treatments such as surgery and chemotherapy, the mortality rate of CRC is high due to drug resistance and metastasis (1). Recent studies showed that many plants have compounds with anti-tumor effects and can inhibit the growth of cancer cells (2). Allium jesdianum extract (AJE) has a therapeutic effect on rheumatoid arthritis and some kidney disorders and is used in eastern medicine. It belongs to the Alliaceae family, which is native to Iran. AJE has antipyretic and antioxidant (3). However, its anti-cancer potential has not been sufficiently studied. Besides, recent evidence has shown insufficient efficacy of anticancer drugs due to their low bioavailability. Drug delivery systems such as liposomes, nanoparticles, microemulsions (MEs), and implants are used to enhance the bioavailability of low water-soluble drugs and decrease their side effects. The MEs have several advantages, such as stable thermodynamics, ease of formulation, low viscosity, high drug load capacity, small droplet size, and high penetration (4).
In this study, the ME technic was used to increase the bioavailability of AJE. Studies on the effect of AJE or AJE-loaded ME (AJE-ME) and the underlying mechanisms on cancer cells in the literature are sparse. Destruction of the cancer cells depends on activating cell death mechanisms such as apoptosis, autophagy, and necroptosis (5).
2. Objectives
In this study, we investigated the toxic effect of AJE-ME on a colon cancer cell line, namely HT-29 cells. In addition, the expression of apoptosis-related genes (Bax and Bcl-2), autophagy-related genes (Atg5, mTOR , and Beclin1), and necroptosis-mediated genes [receptor-interacting protein kinase 1 (RIP1), receptor-interacting protein kinase 3 (RIP3), and mixed lineage kinase domain-like pseudokinase (MLKL)] were investigated in the HT-29 colon cancer cell line.
3. Methods
3.1. Preparation of The Extract
The AJ was collected from the high-altitude regions of Zagros, Iran. This plant was identified by the Herbarium of Agricultural Centre in Ahvaz. The powdered plant was soaked in alcohol 80% for two days to provide the extract by the Soxhlet device. The obtained extract was kept at 4°C until use (3). The standard curve was plotted using various concentrations of AJE in PBS and ethanol. The absorbance was read at 367 nm using a spectrophotometer (Biochrom, England).
3.2. Preparation of Microemulsion
The ME was prepared using the titration method, as previously described. Based on this method, the preparation of AJE-ME was performed in three phases as follows:
Phase I: A stock solution of surfactant (Span 20 and Labrador) and co-surfactant (Pluroloeiqn) were prepared. Oleic acid was used as the oil phase and was mixed with different ratios of surfactant and cosurfactant. AJE (1%) was added to the oil phase and stirred thoroughly until clear.
Phase II: Water was mixed gradually with different ratios of surfactant, and cosurfactant, and stirred until clear.
Phase III: Mixtures of oil and water phases were prepared with different concentrations and were titrated using different amounts of surfactant and co-surfactant.
Finally, they were plotted in triangular coordinates after calculating titrated sample composition and constructing the pseudo ternary phase diagram (6).
3.3. Characterization of AJE-ME
The particle size distribution and mean particle size of the ME formulation of AJE were evaluated by a DLS (dynamic light scattering) device at room temperature (RT). The charge of the AJE-loaded ME (zeta potential) was determined by a Zeta-sizer system (Malvern, UK). The morphology and size of the AJE-ME were evaluated by the TEM (Transmission Electron Microscopy) method.
To determine how much the AJE has been encapsulated and loaded in the synthesized NPs, an indirect method (spectrophotometer) was employed. The entrapment efficiency (EE) and drug loading (DL) percentages were obtained using the following formula:
To determine AJE release from the ME, the prepared AJE-ME was stored in a dialysis bag (cellulose membrane MW cut-off 12 KDa), placed in a 110 ml PBS at 37°C, and stirred.
Then, the samples were analyzed using spectrophotometry at 384 nm (at different time intervals), and the percentage of AJE release was estimated by the following formula:
3.4. Study Design
The human HT-29 and NIH-3T3 cell lines were procured from the Genetic and Biological Reserves of Iran. The cells were stored in DMEM/F12 medium supplemented with FBS 10% and penicillin/ streptomycin and kept in a standard cell culture incubator. Then, the cells were divided into the following groups:
Control: Exposed only to the DMEM/F12 medium
Blank-ME: Received 50 µM/mL of ME without AJE
AJE: Treated with 50 µM/mL of AJE
AJE-ME: Exposed to 50 µM/mL of AJE-ME
The concentration and duration of AJE and AJE-ME were based on our pilot study. The HT-29 and NIH-3T3 cells were exposed to IC50 concentrations of AJE-ME. AJE dissolved in 1% Dimethyl sulfoxide (DMSO) and then diluted in the media culture.
3.5. Cell Viability
Both cell lines were grown on 96-well plates (10,000 cells/ well) and treated with AJE or AJE-MEs for 24 hours. After treatment, the cells were incubated with MTT (0.5 mg/mL) for 3 hours at 37°C. The supernatants were discarded, and DMSO (100 µL) was added to the wells. Finally, the absorbance (OD) was measured at 570 nm.
3.6. Colony Assay
The cells were grown on 4-well dishes (400 cells/well) and treated with AJE or AJE-MEs for 24 hours. The media was discarded, and only the media was added to the wells and maintained for 15 days. The cells were incubated in crystal violet (0.1%) for 10 min at RT. The wells were then washed with PBS and dried for colony counting.
3.7. Cell Apoptosis Analysis Based on Flow Cytometry
The cells were harvested in 6-well dishes (106 cells/ well) and exposed to AJE or AJE-MEs for 24 hours. Then, an Annexin V (FITC)/ PI (propidium iodide) kit (Invitrogen, USA) was used to determine the percentages of the dead or survived cells by flow cytometry. The FITC-/PI- cells were viable, while FITC+/PI-, FITC+/PI+, and FITC-/PI+ cells demonstrated the signs of early apoptosis late apoptosis, and necrosis, respectively.
3.8. Real-Time Polymerase Chain Reaction
The RNAs of the cells (107 cells) were isolated using a RNeasy kit (Qiagen, Germany) and converted to cDNA by a cDNA kit (Qiagen, Germany). An amount of 2 μL of the prepared cDNA was amplified in a 25 μL PCR reaction containing SYBR Green and the primers, which were designed by the Primer-BLAST program (Appendix 1 in Supplementary File). The following program was used in this study: 95°C for 10 min, then 40 cycles consisting of 95°C for 15 s and 60°C for 60 s. The 2-ΔΔCT method was applied to analyze the data.
3.9. Statistical Analysis
Data were analyzed by means of SPSS software (version 22.0, USA) using one-way analysis of variance followed by a post-hoc pairwise comparison through the application of Tukey or LSD tests, or Kruskal-Wallis for non-parametric data. The P-values < 0.05 were statistically significant.
4. Result
4.1. Characterization of AJE-ME
In TEM pictures, the AJE-ME were discrete and had a regular round morphology with a mean particle size of 118.3 ± 16.7 nm. The zeta potential of the AJE-ME was high enough to prevent particle aggregation (Table 1). The amount of AJE loaded on ME (%DL) in our formulation was 29.2, and the amount of AJE encapsulated in ME (%EE) was approximately 96%.
Characteristics of the AJE/Blank-ME Formulation
Characterization | AJE-ME | Blank-ME |
---|---|---|
Particle size (nm) | 118.3 ± 15.7 | 117.71 ± 12.5 |
PDI | 0.21 ± 0.05 | 0.23 ± 0.07 |
Zeta potential (mV) | -29.8 ± 0.25 | -27.4 ± 0.34 |
EE (%) | 96.2 ± 1.5 | - |
DL (%) | 29.2 ± 3.9 | - |
The dynamic dialysis method was used for the measurement of the AJE-release patterns from AJE-ME in vitro. The cumulative release of AJE-ME showed an initial burst release of ~65% in 5 hours and nearly 74% sustainably within 48 hours (Figure 1).
Characterization of AJE-ME. Size distribution (A); Zeta potential distribution (B) of AJE-loaded ME; TEM image of AJE-ME: A spherical morphology and a lipid layer as pale rings around the internal aqueous media are observed (C); In vitro cumulative percent drug release vs. in time. Data expressed as mean ± SD (n = 6).
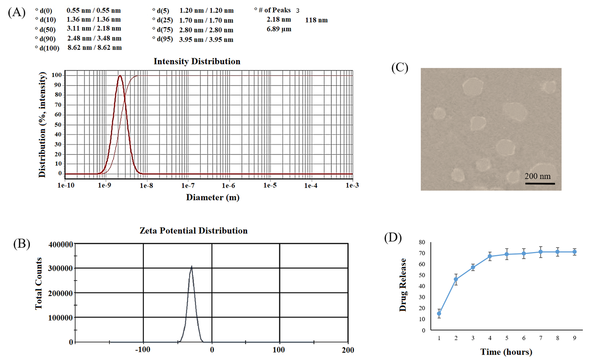
4.2. Cell Viability and Colony Formation
IC50 values were determined (Table 2) to evaluate the cytotoxic effects of AJE-ME on the HT-29 cell growth. The IC50 of AJE-ME was significantly less than that of free AJE at different times. The IC50 of free AJE and AJE-ME in 24 h was slightly more than 48 h. Therefore, the IC50 concentration of AJE-ME in 24 h (50 µM/mL) was used to compare the toxic impacts of AJE and AJE-ME on HT-29 cells.
Treatment/Cells | 12 Hours | 24 Hours | 48 Hours |
---|---|---|---|
AJE | |||
HT-29 | 146.7 ± 9.35 | 98.4 ± 6.54 | 97.8 ± 7.29 |
NIH-3T3 | 291.9 ± 5.13 | 275.4 ± 5.61 | 268.5 ± 3.50 |
AJE-ME | |||
HT-29 | 96.2 ± 6.65 | 50.04 ± 1.37* | 49.7 ± 3.31* |
NIH-3T3 | 273.1 ± 11.75 | 242.4 ± 13.9 | 233.1 ± 12.74 |
Blank-ME | |||
HT-29 | 292.29 ± 5.01 | 288.74 ± 4.3 | 286.82 ± 2.76 |
NIH-3T3 | 310.7 ± 23.63 | 303.1 ± 24.56 | 298.7 ± 13.84 |
AJE could not significantly decrease the viability and colony formation compared to the control cells. The cell survival and colony numbers diminished considerably in the AJE-ME group compared to the control and AJE-exposed cells (P < 0.01). The cell growth and colony formation were not affected by the Blank-ME compared to the control (Figure 2). AJE-ME had no considerable impact on the viability of NIH-3T3 cells (Appendix 2 in Supplementary File).
Morphology (A), the percentage of the viability (B), and Colony number (C and D) in the control and experimental groups (mean ± SD). * P < 0.001; and * indicate comparison to the control.
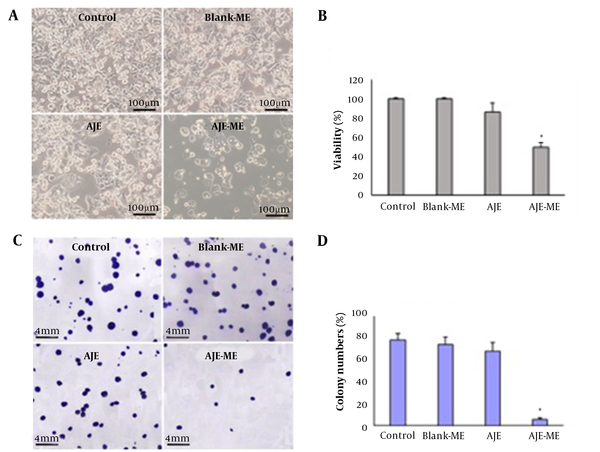
4.3. Annexin V-FITC/Propidium Iodide Assay
In both AJE and AJE-ME exposed HT-29 cells, early apoptosis, late apoptosis, and necrosis percentage were similar to the control cells. AJE-ME significantly increased the rate of necrosis in HT-29 cells compared to the free AJE group (P < 0.05). The apoptosis and necrosis percentages of HT-29 cells had not significantly changed by Blank-ME (Figure 3).
Annexin/PI staining in various groups (mean ± standard deviations). Lower left quadrant: Live cells; Lower right quadrant: Early apoptosis; Upper right quadrant: Late apoptosis; Upper left quadrant: Necrotic cells. * P < 0.05, ** P < 0.01, *** P < 0.001, # P < 0.01, ## P < 0.001; * and # respectively show comparison to control and AJE groups.
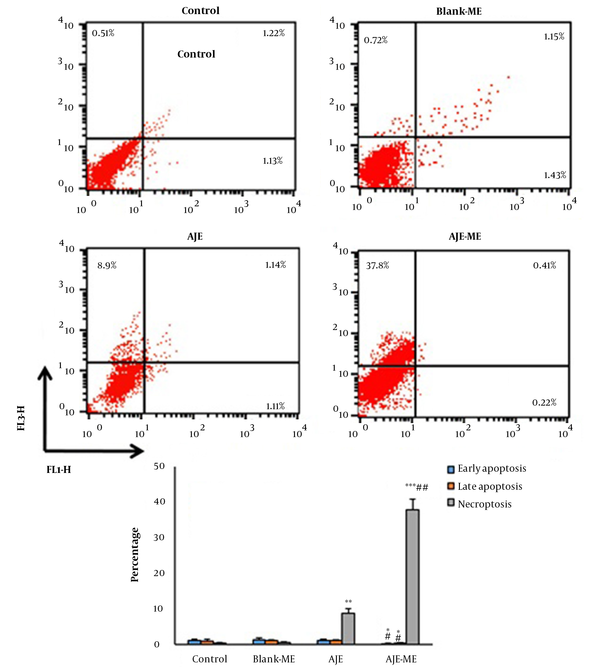
4.4. Real-Time Polymerase Chain Reaction
In AJE-ME-treated HT-29 cells, the expression of Beclin1 and Atg5 genes were significantly decreased while mTOR expression was increased. AJE-ME-treated cells showed a high expression of MLKL and RIP3 compared to the control group (P < 0.05), while the expression of RIP1 was reduced in this group.
In the AJE-ME exposed HT-29 cells, expression of the Bax was slightly decreased while the expression of the Bcl-2 was slightly increased compared to the control and AJE-treated cells (Figures 4). In Blank-ME exposed cells, the expression of all genes was similar to the control.
Gene expression in different groups. Expression normalized to the average of the GAPDH gene (mean ± SD). * P < 0.05, # P < 0.05; * and # respectively show comparison to control and AJE-treated cells.
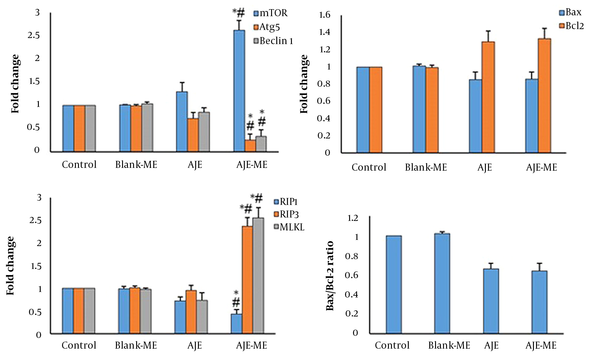
5. Discussion
In the present study, AJE was successfully loaded on ME by means of a micro emulsification method. The AJE-ME showed a homogenous size distribution with acceptable stability. The release profile showed that the AJE-ME could provide a slow release of AJE during treatment which may be suitable for delayed drug release in chemotherapy of colon cancers.
The AJE-ME effectively decreased the survival of the HT-29 cells. The IC50 of AJE-ME was markedly lower than that of the free AJE, indicating higher toxicity of the AJE-ME on the HT-29 cells. The cell growth was lower than free AJE, indicating that the AJE-ME is a sound delivery system for the colon cancer cells, which is more effective than AJE alone in HT-29 cells.
Chen et al. showed the inhibitory effects of Curcuma Longa extract loaded in ME on the growth of HT-29 cells (7). The enhanced preventive effect of AJE-ME on the growth of HT-29 cells may be related to the lipophilic property of the carrier, which increases the cellular uptake. Chen et al. showed that Caffeic acid phenethyl ester (CAPE) loaded in ME markedly increased cellular uptake of CAPE in colon cancer cells (8). We examined the potentials of AJE-ME in inducing apoptosis in HT-29 cells by flow cytometry and gene expression. The expression level of Bcl-2 had slightly increased while the expression of Bax slightly decreased in AJE-ME- treated HT-29 cells. This finding indicates that AJE-ME can partially suppress apoptosis in HT-29 cells. Flow cytometry results also demonstrated a meager apoptosis rate in AJE-ME-treated colon cancer cells. Despite the inhibition of apoptosis, the viability of the HT-29 cells was decreased by AJE-ME treatment. Other modes of cell death may be involved in the AJE-ME-induced cytotoxicity. Several studies have demonstrated a close relationship between autophagy and Bcl2 family proteins. Bcl-2 (anti-apoptotic protein) connects to Beclin-1 and inhibits autophagy. Bcl-2 down-regulation can cause autophagy in human leukemic HL60 cells (9). Hence, in the present study, genes associated with autophagy were evaluated. Our findings demonstrated that AJE-ME could upregulate mTOR expression in the HT-29 cells. mTOR acts as a negative autophagy mechanism regulator and inhibits autophagy activation (10). In contrast, expressions of autophagy-related gene 5 (Atg5) and Beclin-1 were downregulated. These observations are in line with the studies that showed autophagy inhibition could increase cancer cell death (11). Autophagy inhibition via the mTOR pathway increases necroptotic cell death (12). Our flow cytometry results showed more necrosis in AJE-ME treated cells than in control untreated cells. Necrosis often occurs in a regulated manner, namely necroptosis. Besides, when apoptosis and autophagy processes are blocked, necroptosis is activated to destroy cancer cells (13). In this study, the expression of central regulators of necroptosis was investigated in the HT-29 cells. The expressions of RIP3 and MLKL were enhanced in AJE-ME treated HT-29 cells. RIP3 and MLKL play a vital role in necroptosis. Phosphorylated MLKL translocates to plasma membranes and results in permeabilization of the endoplasmic reticulum, mitochondria, and lysosome (14, 15). Expression of RIP3 is reduced in patients with breast cancer, and RIP3 defect is associated with an increased cancer rate (16). Oxaliplatin and anthracyclines activate necroptosis in cancer cell lines through the expression RIP3 and MLKL (17). Decreased MLKL expression has been reported in gastric (18), cervical (19), and pancreatic cancers (20). MLKL is more influential than RIP3 in terms of whether or not a cell undergoes apoptosis or necroptosis (21). It has been reported that the expression of RIP3 is commonly silenced in cancers, rendering most cancer cells incapable of undergoing necroptosis (16). It has been demonstrated that RIP3 is only activated following binding to RIP1.
5.1. Conclusions
In summary, the present study demonstrated that AJE-ME at the dose of 50 µM/mL could significantly suppress the viability and proliferation of HT-29 cells by activating necroptosis and suppressing autophagy signaling pathways.
References
-
1.
Siegel RL, Miller KD, Fuchs HE, Jemal A. Cancer statistics, 2022. CA Cancer J Clin. 2022;72(1):7-33. [PubMed ID: 35020204]. https://doi.org/10.3322/caac.21708.
-
2.
Sznarkowska A, Kostecka A, Meller K, Bielawski KP. Inhibition of cancer antioxidant defense by natural compounds. Oncotarget. 2017;8(9):15996-6016. [PubMed ID: 27911871]. [PubMed Central ID: PMC5362541]. https://doi.org/10.18632/oncotarget.13723.
-
3.
Khaksarian M, Gholami E, Alipour M, Sabooteh T, Asadi-Samani M. Investigation of the effects of the essence and extract of Allium jesdianum on the activity of COX-1 and COX-2 enzymes. Int J Adv Biotechnol Res. 2017;8(2):1095-101.
-
4.
Ghosh PK, Murthy RS. Microemulsions: a potential drug delivery system. Curr Drug Deliv. 2006;3(2):167-80. [PubMed ID: 16611003]. https://doi.org/10.2174/156720106776359168.
-
5.
Ouyang L, Shi Z, Zhao S, Wang FT, Zhou TT, Liu B, et al. Programmed cell death pathways in cancer: a review of apoptosis, autophagy and programmed necrosis. Cell Prolif. 2012;45(6):487-98. [PubMed ID: 23030059]. [PubMed Central ID: PMC6496669]. https://doi.org/10.1111/j.1365-2184.2012.00845.x.
-
6.
Salimi A, Sharif Makhmal Zadeh B, Moghimipour E. Preparation and characterization of cyanocobalamin (vit B12) microemulsion properties and structure for topical and transdermal application. Iran J Basic Med Sci. 2013;16(7):865-72. [PubMed ID: 23997918]. [PubMed Central ID: PMC3758059].
-
7.
Chen YC, Chen BH. Preparation of curcuminoid microemulsions from Curcuma longa L. to enhance inhibition effects on growth of colon cancer cells HT-29. RSC Adv. 2018;8(5):2323-37. [PubMed ID: 35541476]. [PubMed Central ID: PMC9077335]. https://doi.org/10.1039/c7ra12297g.
-
8.
Chen H, Guan Y, Baek SJ, Zhong Q. Caffeic acid phenethyl ester loaded in microemulsions: enhanced in vitro activity against colon and breast cancer cells and possible cellular mechanisms. Food Biophysics. 2019;14(1):80-9.
-
9.
Qian HR, Shi ZQ, Zhu HP, Gu LH, Wang XF, Yang Y. Interplay between apoptosis and autophagy in colorectal cancer. Oncotarget. 2017;8(37):62759-68. [PubMed ID: 28977986]. [PubMed Central ID: PMC5617546]. https://doi.org/10.18632/oncotarget.18663.
-
10.
Jung CH, Ro SH, Cao J, Otto NM, Kim DH. mTOR regulation of autophagy. FEBS Lett. 2010;584(7):1287-95. [PubMed ID: 20083114]. [PubMed Central ID: PMC2846630]. https://doi.org/10.1016/j.febslet.2010.01.017.
-
11.
Gurkan AC, Arisan ED, Yerlikaya PO, Ilhan H, Unsal NP. Inhibition of autophagy enhances DENSpm-induced apoptosis in human colon cancer cells in a p53 independent manner. Cell Oncol (Dordr). 2018;41(3):297-317. [PubMed ID: 29492901]. https://doi.org/10.1007/s13402-017-0369-x.
-
12.
Su Z, Yang Z, Xie L, DeWitt JP, Chen Y. Cancer therapy in the necroptosis era. Cell Death Differ. 2016;23(5):748-56. [PubMed ID: 26915291]. [PubMed Central ID: PMC4832112]. https://doi.org/10.1038/cdd.2016.8.
-
13.
Hu B, Shi D, Lv X, Chen S, Huang Q, Xie M, et al. Prognostic and clinicopathological significance of MLKL expression in cancer patients: a meta-analysis. BMC Cancer. 2018;18(1):736. [PubMed ID: 30005626]. [PubMed Central ID: PMC6044038]. https://doi.org/10.1186/s12885-018-4655-4.
-
14.
Dhuriya YK, Sharma D. Necroptosis: a regulated inflammatory mode of cell death. J Neuroinflammation. 2018;15(1):199. [PubMed ID: 29980212]. [PubMed Central ID: PMC6035417]. https://doi.org/10.1186/s12974-018-1235-0.
-
15.
Koo GB, Morgan MJ, Lee DG, Kim WJ, Yoon JH, Koo JS, et al. Methylation-dependent loss of RIP3 expression in cancer represses programmed necrosis in response to chemotherapeutics. Cell Res. 2015;25(6):707-25. [PubMed ID: 25952668]. [PubMed Central ID: PMC4456623]. https://doi.org/10.1038/cr.2015.56.
-
16.
Yang H, Ma Y, Chen G, Zhou H, Yamazaki T, Klein C, et al. Contribution of RIP3 and MLKL to immunogenic cell death signaling in cancer chemotherapy. Oncoimmunology. 2016;5(6). e1149673. [PubMed ID: 27471616]. [PubMed Central ID: PMC4938314]. https://doi.org/10.1080/2162402X.2016.1149673.
-
17.
Ertao Z, Jianhui C, Kang W, Zhijun Y, Hui W, Chuangqi C, et al. Prognostic value of mixed lineage kinase domain-like protein expression in the survival of patients with gastric caner. Tumour Biol. 2016;37(10):13679-85. [PubMed ID: 27473085]. https://doi.org/10.1007/s13277-016-5229-1.
-
18.
Ruan J, Mei L, Zhu Q, Shi G, Wang H. Mixed lineage kinase domain-like protein is a prognostic biomarker for cervical squamous cell cancer. Int J Clin Exp Pathol. 2015;8(11):15035-8. [PubMed ID: 26823841]. [PubMed Central ID: PMC4713627].
-
19.
He L, Peng K, Liu Y, Xiong J, Zhu FF. Low expression of mixed lineage kinase domain-like protein is associated with poor prognosis in ovarian cancer patients. Onco Targets Ther. 2013;6:1539-43. [PubMed ID: 24204164]. [PubMed Central ID: PMC3817086]. https://doi.org/10.2147/OTT.S52805.
-
20.
Su X, Wang H, Lin Y, Chen F. RIP1 and RIP3 mediate hemin-induced cell death in HT22 hippocampal neuronal cells. Neuropsychiatr Dis Treat. 2018;14:3111-9. [PubMed ID: 30532542]. [PubMed Central ID: PMC6247969]. https://doi.org/10.2147/NDT.S181074.
-
21.
Moriwaki K, Chan FK. Regulation of RIPK3- and RHIM-dependent Necroptosis by the Proteasome. J Biol Chem. 2016;291(11):5948-59. [PubMed ID: 26786097]. [PubMed Central ID: PMC4786728]. https://doi.org/10.1074/jbc.M115.700997.