Abstract
Background:
The flavonoid 2′,4′-dihydroxy-5′-(1′′′,1′′′-dimethylalil)-8-prenylpinocembrin (8PP), obtained from Dalea elegans roots has demonstrated antifungal activities. It also overcomes the resistance to azoles in azole-resistant Candida albicans (RCa). It collaborates with fluconazole to decrease cell growth and viability and inhibits ABC cdr transporters at the carrying site and associated ATPase. In addition, 8PP reduces cell viability dependent on oxidoreductase activity in planktonic cultures. It shows a dual oxidant-antioxidant activity with accumulating endogenous reactive oxygen species (ROS) in RCa biofilms.Objectives:
This work evaluated the effects of 8PP, fluconazole, and their combination on RCa viability, which is dependent on cell permeability.Methods:
RCa 12 - 99 strain of oral origin was used. Its viability was assessed through propidium iodide (PI) cell uptake and observing yeast morphology by fluorescence microscopy.Results:
RCa 12 - 99 strain cells, collected in the mid-exponential phase, incorporated PI and completely stained red after incubation with 8PP or fluconazole at concentrations that inhibit cell growth and viability. When combined at 125 µM of each, 8PP and fluconazole appeared more effective than separately. The results indicated that the compounds tested impaired cell permeability directly or indirectly.Conclusions:
8PP and fluconazole and their combination caused alterations in cell permeability in azole-resistant C. albicans. They were effective both individually and in combination.Keywords
Prenylated Flavonoid Dalea elegans Fabaceae Antifungal Activity Azole-Resistant Candida albicans Cell Permeability Propidium Iodide Uptake
1. Background
Mycoses have become important in medical and dental clinics in recent decades. Among them, candidiasis has grown dramatically worldwide due to the increased number of immunodeficient patients. Regarding the species involved, Candida albicans is very prominent as it causes important diseases (1). In this context, its hyphae are demonstrated together with highly dangerous anaerobic bacteria in connective tissues of periodontal patients (2, 3).
The extent and severity of the mentioned mycoses and the emergence of resistance to antifungal drugs and pharmacological interventions necessitate the search for new medicines and pharmacological strategies against it (4-6).
The biological activity of the compound 2′, 4′-dihydroxy-5′-(1′′′,1′′′-dimethylalil)-8-prenylpinocembrin (8PP), isolated from roots of Dalea elegans, is demonstrated using different microorganisms including multi-resistant fungal species of nosocomial origin (7-9).
In further studies, the chemical structure of that prenylated flavonoid was reinterpreted as 2′,4′- dihydroxy-5′-(1′′′,1′′′-dimethylallyl)-8-prenylpinocembrin (8PP), which means that the prenyl group changed its position from C-6 to C-8 (10).
The 8PP was extracted with EtOH and chromatographed on a silica gel column using C,H,-EtOAc and increasing concentrations of EtOAc as eluent. Subsequently, the eluate was chromatographed again on silica gel TLC using C,H,-EtOH (7). The produced substance was identified by spectroscopic and spectrophotometric techniques (7, 10).
The compound 8PP reverses resistance in azole-resistant C. albicans (RCa), decreases cell growth and viability in planktonic cultures, and has a synergic effect with fluconazole through added interactions. In fact, besides destroying by itself, 8PP collaborates with fluconazole (FCZ) to achieve a virtually complete fungicidal effect (5, 11). This is crucial to eradicate fungal infections because the major shortcoming of azole antifungals is their fungistatic effect, which leads to drug resistance (12). This is of crucial importance as candidiasis is generally treated with azole antifungals, which have lately become ineffective (1, 12).
In this context, overcoming resistance by 8PP is correlated with inhibiting the active azole transport mediated through the carry sites of ABC cdr efflux pumps (5). Concomitantly, 8PP decreases the energy available for azole transport by inhibiting both, i.e., the site located at the ATPase associated with cdr pumps and the cell oxidoreductase activity (13).
On the other hand, 8PP demonstrates a pro-oxidant activity that increases the synthesis and accumulation of endogenous reactive oxygen species (ROS) and reactive nitrogen intermediates (RNI) and inhibits the formation of C. albicans biofilms (14). Excessive ROS can cause alterations to cell permeability and decrease viability, among other damages (15). Curiously, 8PP decreased viability depends on redox activity balance in RCa planktonic cultures at higher concentrations than those related to the ones mentioned above for RCa biofilms, thus indicating that the oxidoreductase activity would not be a crucial 8PP target in RCa in the first cultures (13). Thus, 8PP may act by other mechanisms that alter cell viability, such as plasma membrane lesions (1, 4).
2. Objectives
We studied the effects of 8PP on viability related to cell permeability using RCa planktonic cultures. Propidium iodide (PI) cell uptake was measured for this purpose.
3. Methods
3.1. Compounds
The flavonoid 2′,4′-dihydroxy-5′-(1′′′,1′′′-dimethylallyl)- 8-prenyl-pinocembrin (8PP), molecular weight: 424), formerly formulated as 2′,4′- dihydroxy-5′-(1′′′,1′′′-dimethylallyl)-6-prenyl-pinocembrin (6PP, molecular weight: 424), was provided by IMBIV (CONICET), and, as previously reported, was extracted and isolated from Dalea elegans roots and aerial parts (5, 7, 10). It was extracted with EtOH and chromatographed on a silica gel column using C,H,-EtOAc with increasing concentrations of EtOAc as eluent. The eluate was re-chromatographed on silica gel TLC using C,H,-EtOH (7). Its structure was characterized by UV, NMR1H and 13C, and HRMS (10). The purity of 8PP was 95%, as measured by a Varian (Palo Alto, CA) Pro Star HPLC equipment which was associated with a UV detector (Varian) (10). In detail, the elution was carried out in a Varian C18 column (Ø250 mm × 4.6 mm) to analyze the purity of 8PP under two different conditions. Method 1: Mobile phase: (A) H2O, (B) MeOH; elution program: Linear gradient from 50% B to 85% bin 60 min, followed by 100% B maintained for 10 min and finally linear gradient from100% B to 50% bin 10 min. Method 2: Mobile phase was composed of acetonitrile 1% acetic acid (A) and H2O 1% acetic acid; (B) with gradient elution system: 0 - 10 min, 10 - 40% A; 10 - 15 min, 40 - 50% A, maintained for 5 min; 20 - 25 min, 50 - 60% A; 25 - 30 min, 60 - 30% A; 30 - 35 min, 30 - 10% A, maintained for 5 min. Both methods were performed at a flow rate of 1.0 mL/min; detection wavelength: 290 nm; injection volume: 20 μL; temperature: 30°C.
Fluconazole and propidium iodide were purchased from Sigma Aldrich (Saint-Louis, MO, USA).
3.2. Microorganisms
This work used an azole-resistant C. albicans strain donated by Dr. Theodore White (University of Washington, Seattle, USA). It was previously isolated from the oral cavity of a patient with AIDS who presented with recurrent oropharyngeal candidiasis. It was characterized and reported to overexpress ABC transporters cdr1 and cdr2, as well as Mdr1 (16). Herein, the strain was named Isolate 17 g [isolate 12-99] and abbreviated 12 - 99 as in the same original paper (16).
Our labs stored the microorganism at -70°C in 15% glycerol in an aqueous medium or sterile distilled water. For the assays, it was cultured in yeast peptone dextrose (YPD) broth or Sabouraud agar medium and then was resuspended according to the experiment performed and described below.
3.3. Preparation of Azole-Resistant Candida albicans Planktonic Cultures
Cells were activated from an aqueous medium by adding YPD and incubating them at 36°C overnight. Subsequently, an aliquot of the suspension was plated on Sabouraud agar, and the resulting preparation was incubated overnight. Then, a portion of the resulting culture was suspended in YPD to obtain a value of 0.10 optical density u (OD u), which turned to 1,00 OD u (equivalent to 107 CFU/mL) after incubation at 36°C for 18 h in our experimental conditions. This latter suspension was diluted 1/10 v/v to obtain 0.10 OD units, corresponding to 106 CFU/mL, and used for PI uptake experiments described below. These procedures were extracted from kinetic studies on azole-resistant C. albicans growth (11). In this context, the yeasts were grown in YPD broth up to the late exponential phase and diluted to return to and remain in the mid-exponential phase throughout the experiment.
3.4. Propidium Iodide Cell Uptake
The assays were conducted according to Shreaz et al. (17). A confocal scanning laser microscope (CSLM) was used to study the effect of 8PP and other drugs on the architecture of yeast cells.
3.5. Preparation of Drug Solutions
Fluconazole and 8PP were dissolved in DMSO/water 1/10 v/v. Propidium iodide was dissolved in sterile distilled water to prepare a 1 mg/mL concentration. Subsequently, it was diluted 1/10 v/v in FS to obtain a 100 µg/mL concentration for adding to the tubes. The first two mentioned PI solutions were kept in the refrigerator at 5°C.
3.6. Incubation of RCa 12 - 99 Cells with Different Compounds
Briefly, 135 µL of planktonic yeasts (106 cells/mL), grown up to mid-exponential phase and obtained as described above, were incubated in the dark at 37°C for 30 min with 15 µL of the compounds tested, i.e., fluconazole (125 µM), 8PP (125 or 250 µM) or their combination at 125 µM. As a reference active drug, ethanol at 70% v/v concentration was used. Control samples were treated in the absence of drugs and the presence of their respective solvents. Incubation was made in a final volume of 150 µL using Eppendorf tubes under the abovementioned conditions.
3.7. Preparation and Observation of RCa 12 - 99 Cells
After incubation, the samples were centrifuged twice at 3000 rpm/min in a Rolco centrifuge, with subsequent washings and resuspension in FS. Then, propidium iodide at 100 µg/mL was added to the cell suspensions to obtain a final 10 µg/mL concentration. The cells were then examined by CSLM at 543 nm through red fluorescence produced by PI staining inside the damaged cells. ALeica TCS SPE CSLM (Manheim, Germany) was used.
The GraphPad Prism version 5.00 software (GraphPad Software San Diego, CA, USA) was used to plot and analyze results.
4. Results
4.1. Effect of 8PP on Azole-Resistant Candida albicans Cell Permeability
This effect was studied by detecting cell propidium iodide uptake through fluorescence microscopy. As demonstrated, the CSLM technique is used for obtaining high-resolution optical images with depth selectivity. It was used herein to identify C. albicans dead cells after incubation with 8PP or other drugs and further exposure to PI. This compound is membrane-impermeant and is generally excluded from viable cells. Cells with great membrane lesions internalize PI and produce an increased red fluorescence (18).
As expected, in our work, untreated cells (control) demonstrated no visible staining (Figure 1A). By contrast, the reference compound ethanol at 70% w/w visualized a considerable number of red fluorescent yeasts (69%; Figure 1B).
The activity of 2′,4′-dihydroxy-5′-(1′′′,1′′′-dimethylallyl)-8-prenylpinocembrin (8PP), fluconazole (FCZ) and their combination on propidium iodide (PI) uptake by azole-resistant Candida albicans 12 - 99. The cells were preincubated with the tested compounds, and their PI uptake was detected as staining through confocal scanning laser microscopy (CSLM) as described in the materials and methods. Fluorescence was measured at 543 nm. A, control cells; B, 70% w/w ethanol; C, 125 µM fluconazole; D, 125 µM 8PP; E, 250 µM 8PP; F, FCZ + 8PP at 125 µM of each compound. Control cells were studied in the absence of the drugs tested. Ethanol was used as a reference drug. B - F, cells treated with the compounds at the mentioned concentrations. Images of the living and dead (stained red in the right panel) yeasts are shown at 40x magnification. Results from one of three typical experiments are shown.
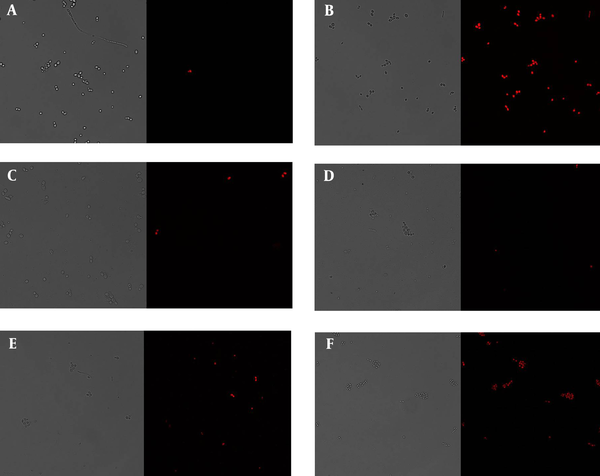
To compare drugs and ensure a high level of yeasts growth inhibition and death in the experimental conditions, we used 8PP at 125 µM and 250 µM, which are in the range of its minimum inhibitory concentration (MIC; 150 µM) (5) and fungicidal concentration 50% (FC50:150 µM) (11). As in previous viability studies and considering the low activity of FCZ on the azole-resistant C. albicans, a concentration of 125 µM was chosen for this compound to start with a value lower than its FC50 (280 µM) (11), and MIC (1126 µM (5), following the criteria of Klepser et al. (19).
As shown in Figures 1C, D, E, and 2, FCZ produced 5% PI uptake, whereas 8PP showed values of 8% at 125 µM and 39% at 250 µM. The combination of FCZ with 8PP at 125 µM of each compound produced a greater effect than them individually (50%; Figures 1C, D, F, and 2). These results, demonstrated in Figures 1 and 2, were similar to those obtained in two more independent experiments. The results demonstrated some cell wall and membrane damage leading to subsequent PI uptake and nucleic acid staining. The damages mentioned above could have occurred directly on the cell membrane or indirectly as a consequence of the action on other targets.
Percentage activity of 8PP, fluconazole (FCZ), and their combination on propidium iodide (PI) uptake by azole-resistant Candida albicans 12 - 99 strain. The percentage of PI-stained cells was calculated concerning the cells observed in each sample. The data represent the experiment depicted in Figure 1.
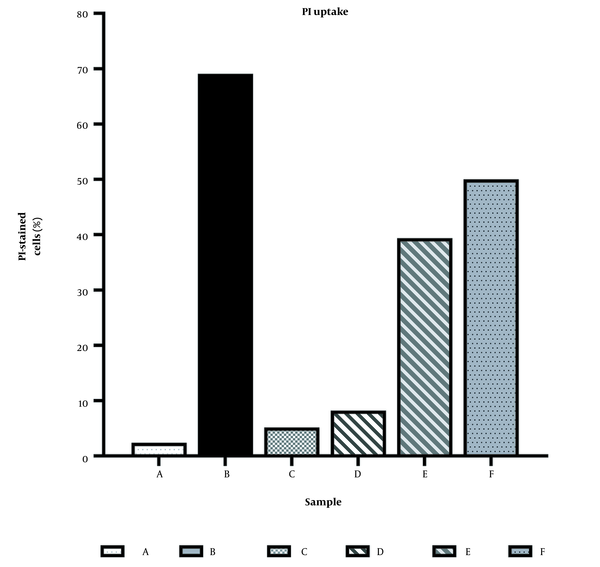
5. Discussion
The flavonoid 8PP inhibited the cell viability dependent on oxido-reductase activity in azole-resistant C. albicans planktonic cultures (13). This effect was weaker than others previously reported for the same cultures (5, 11) or biofilms (14).
In this work, the effects of 8PP on viability dependent on alterations to cell permeability were studied through the PI uptake technique. Therefore, cells with damaged membranes internalized PI and produced red fluorescence within them (18).
Whereas the reference drug ethanol 70% v/v showed 69% PI uptake, that of 8PP were 8 and 39% at 125 and 250 µM, respectively. The first 8PP’s concentration corresponded to its MIC and FC50, whereas the second one doubled its value (Figures 1 and 2). The loss of permeability detected could be due to, at least in part, the fungicidal effect detected through other techniques, such as CFU counting (11).
Our results were consistent with other studies using the PI technique and several antifungal flavonoids that, per se, can disrupt cell membranes of the genus Candida. Among them, we can mention isoquercitrin, which mediates the fungicidal effect through disorganization and depolarization of the membrane, as well as perturbation of its lipid bilayer in azole-sensitive ATCC 90028 C. albicans (20).
It is tempting to attribute the loss of membrane permeability to excessive production of ROS, as reported for many compounds, such as the phenylpropanoids methyl chavicol and linalool obtained from Ocimum sanctum (15, 21). This is consistent with the accumulation of endogenous ROS and RNI elicited by 8PP in C. albicans biofilms (14). However, the redox activity balance is not strongly modified by 8PP in RCa planktonic cultures at the concentrations used here. This may be explained by the fact that a dual 8PP’s antioxidant-prooxidant activity operates in planktonic cultures, as reported in C. albicans biofilms (13, 14), but with different magnitudes. Nonetheless, the concentrations used in the PI study (125, 250 µM) are included in the range of those utilized in the MTT assay (30 - 1000 µM).
Our preliminary PI capture results suggested a concentration-response relationship for 8PP, possibly with different parameters related to MTTs. Moreover, much higher activity was demonstrated by the combination of FCZ and 8PP at 125 M in the PI study (Figures 1C, D, F, and 2) in contrast with that from the MTT’s one. As cell oxidoreductase activity does not seem to be a major target for 8PP in RCa planktonic cultures (13), these results suggest that 8PP may act on another biological site, such as the plasma membrane. For instance, it may modify ergosterol synthesis or content, as suggested for cinnamaldehyde derivatives by Shreaz et al. (17) in a similar context. This hypothesis should be tested.
Cell wall deformation may also be involved in the permeability alterations caused by 8PP and observed through PI staining. In this sense, similar effects have been reported for the flavonoid glabridin (22). It is worth to mention that the derived prenylated glabridin induces apoptosis through cell deformation (23). It would be interesting to explore the effects of 8PP regarding apoptosis in RCa planktonic cultures.
On the other hand, the cooperation between 8PP and FCZ shown in this study could add other similar interactions previously reported regarding fungistatic and fungicidal effects and inhibition of ATPase associated with cdr transporters (5, 11, 13). Other flavonoids, such as quercetin, catechin, or epigallocatechin gallate, collaborate with FCZ to induce apoptosis on azole-resistant C. tropicalis by exposure of phosphatidyl serine in its plasma membranes and mitochondrial depolarization, among other effects (24). Interestingly, the compound 8PP produces oxidative phosphorylation decoupling, alteration in cell permeability, and death in human cancer cells HEp-2 (25).
Considering all reports published on 8PP’s efficacy and safety hitherto (5, 11, 13, 25), further investigations on its pharmacological effects are warranted.
5.1. Conclusions
At fungistatic or fungicidal concentrations, the compounds 8PP and fluconazole and their combination can alter cell permeability with different magnitudes. Cooperative interaction of 8PP and at 125 µM of each compound appears to be responsible for this effect.
Acknowledgements
References
-
1.
Kim J, Sudbery P. Candida albicans, a major human fungal pathogen. J Microbiol. 2011;49(2):171-7. [PubMed ID: 21538235]. https://doi.org/10.1007/s12275-011-1064-7.
-
2.
Reynaud AH, Nygaard-Ostby B, Boygard GK, Eribe ER, Olsen I, Gjermo P. Yeasts in periodontal pockets. J Clin Periodontol. 2001;28(9):860-4. [PubMed ID: 11493356]. https://doi.org/10.1034/j.1600-051x.2001.028009860.x.
-
3.
Rubio NA, Puia S, Toranzo S, Brusca MI. [Fungal invasion of connective tissue in patients with gingival-periodontal disease]. Rev Iberoam Micol. 2015;32(1):20-4. Spanish. [PubMed ID: 22824245]. https://doi.org/10.1016/j.riam.2012.07.002.
-
4.
Sharma M, Manoharlal R, Shukla S, Puri N, Prasad T, Ambudkar SV, et al. Curcumin modulates efflux mediated by yeast ABC multidrug transporters and is synergistic with antifungals. Antimicrob Agents Chemother. 2009;53(8):3256-65. [PubMed ID: 19470507]. [PubMed Central ID: PMC2715616]. https://doi.org/10.1128/AAC.01497-08.
-
5.
Peralta MA, Calise M, Fornari MC, Ortega MG, Diez RA, Cabrera JL, et al. A prenylated flavanone from Dalea elegans inhibits rhodamine 6 G efflux and reverses fluconazole-resistance in Candida albicans. Planta Med. 2012;78(10):981-7. [PubMed ID: 22673834]. https://doi.org/10.1055/s-0031-1298627.
-
6.
Muthular M, Balsamo F, Passero P, Jewtuchowicz V, Miozza V, Villalba MB, et al. Effects of tamoxifen on periodontal disease and Candida albicans of patients with breast cancer and other pathologies. Future Microbiol. 2019;14:129-37. [PubMed ID: 30672328]. https://doi.org/10.2217/fmb-2018-0272.
-
7.
Caffaratti M, Ortega MG, Scarafia ME, Ariza Espinar L, Juliani HR. Prenylated flavanones from Dalea elegans. Phytochemistry. 1994;36(4):1082-4. https://doi.org/10.1016/s0031-9422(00)90498-9.
-
8.
Ortega MG, Scarafia ME, Juliani HR. Antimicrobial Agents in Dalea elegans. Fitoterapia. 1996;67(1):81.
-
9.
Perez C, Tiraboschi IN, Ortega MG, Agnese AM, Cabrera JL. Further Antimicrobial Studies of 2'4'-dihidroxy-5'-(1?-dimethylallyl)-6-prenylpinocembrin from Dalea elegans. Pharm Biol. 2003;41(3):171-4. https://doi.org/10.1076/phbi.41.3.171.15090.
-
10.
Peralta MA, Santi MD, Agnese AM, Cabrera JL, Ortega MG. Flavanoids from Dalea elegans: Chemical reassignment and determination of kinetics parameters related to their anti-tyrosinase activity. Phytochem Lett. 2014;10:260-7. https://doi.org/10.1016/j.phytol.2014.10.012.
-
11.
Barcelo S, Peralta M, Calise M, Finck S, Ortega G, Diez RA, et al. Interactions of a prenylated flavonoid from Dalea elegans with fluconazole against azole- resistant Candida albicans. Phytomedicine. 2017;32:24-9. [PubMed ID: 28732804]. https://doi.org/10.1016/j.phymed.2017.05.001.
-
12.
Pfaller MA, Sheehan DJ, Rex JH. Determination of fungicidal activities against yeasts and molds: lessons learned from bactericidal testing and the need for standardization. Clin Microbiol Rev. 2004;17(2):268-80. [PubMed ID: 15084501]. [PubMed Central ID: PMC387411]. https://doi.org/10.1128/CMR.17.2.268-280.2004.
-
13.
Passero P, Muthular M, Barcelo S, Miozza V, Perez C. Inhibition of azole-resistant Candida albicans ATPase and oxidoreductase activity by a flavonoid from Dalea elegans. J Mycol Med. 2022;32(2):101247. [PubMed ID: 35124537]. https://doi.org/10.1016/j.mycmed.2022.101247.
-
14.
Peralta MA, da Silva MA, Ortega MG, Cabrera JL, Paraje MG. Antifungal activity of a prenylated flavonoid from Dalea elegans against Candida albicans biofilms. Phytomedicine. 2015;22(11):975-80. [PubMed ID: 26407939]. https://doi.org/10.1016/j.phymed.2015.07.003.
-
15.
Wong-Ekkabut J, Xu Z, Triampo W, Tang IM, Tieleman DP, Monticelli L. Effect of lipid peroxidation on the properties of lipid bilayers: a molecular dynamics study. Biophys J. 2007;93(12):4225-36. [PubMed ID: 17766354]. [PubMed Central ID: PMC2098729]. https://doi.org/10.1529/biophysj.107.112565.
-
16.
White TC, Holleman S, Dy F, Mirels LF, Stevens DA. Resistance mechanisms in clinical isolates of Candida albicans. Antimicrob Agents Chemother. 2002;46(6):1704-13. [PubMed ID: 12019079]. [PubMed Central ID: PMC127245]. https://doi.org/10.1128/AAC.46.6.1704-1713.2002.
-
17.
Shreaz S, Wani WA, Behbehani JM, Raja V, Irshad M, Karched M, et al. Cinnamaldehyde and its derivatives, a novel class of antifungal agents. Fitoterapia. 2016;112:116-31. [PubMed ID: 27259370]. https://doi.org/10.1016/j.fitote.2016.05.016.
-
18.
Pina-Vaz C, Sansonetty F, Rodrigues AG, Costa-Oliveira S, Tavares C, Martinez-de-Oliveira J. Cytometric approach for a rapid evaluation of susceptibility of Candida strains to antifungals. Clin Microbiol Infect. 2001;7(11):609-18. [PubMed ID: 11737085]. https://doi.org/10.1046/j.1198-743x.2001.00307.x.
-
19.
Klepser ME, Wolfe EJ, Jones RN, Nightingale CH, Pfaller MA. Antifungal pharmacodynamic characteristics of fluconazole and amphotericin B tested against Candida albicans. Antimicrob Agents Chemother. 1997;41(6):1392-5. [PubMed ID: 9174207]. [PubMed Central ID: PMC163923]. https://doi.org/10.1128/AAC.41.6.1392.
-
20.
Yun J, Lee H, Ko HJ, Woo ER, Lee DG. Fungicidal effect of isoquercitrin via inducing membrane disturbance. Biochim Biophys Acta. 2015;1848(2):695-701. [PubMed ID: 25445674]. https://doi.org/10.1016/j.bbamem.2014.11.019.
-
21.
Khan A, Ahmad A, Akhtar F, Yousuf S, Xess I, Khan LA, et al. Induction of oxidative stress as a possible mechanism of the antifungal action of three phenylpropanoids. FEMS Yeast Res. 2011;11(1):114-22. [PubMed ID: 21114624]. https://doi.org/10.1111/j.1567-1364.2010.00697.x.
-
22.
Liu W, Li LP, Zhang JD, Li Q, Shen H, Chen SM, et al. Synergistic antifungal effect of glabridin and fluconazole. PLoS One. 2014;9(7):e103442. [PubMed ID: 25058485]. [PubMed Central ID: PMC4110026]. https://doi.org/10.1371/journal.pone.0103442.
-
23.
Moazeni M, Hedayati MT, Nabili M, Mousavi SJ, Abdollahi Gohar A, Gholami S. Glabridin triggers over-expression of MCA1 and NUC1 genes in Candida glabrata: Is it an apoptosis inducer? J Mycol Med. 2017;27(3):369-75. [PubMed ID: 28595940]. https://doi.org/10.1016/j.mycmed.2017.05.002.
-
24.
da Silva CR, de Andrade Neto JB, de Sousa Campos R, Figueiredo NS, Sampaio LS, Magalhaes HI, et al. Synergistic effect of the flavonoid catechin, quercetin, or epigallocatechin gallate with fluconazole induces apoptosis in Candida tropicalis resistant to fluconazole. Antimicrob Agents Chemother. 2014;58(3):1468-78. [PubMed ID: 24366745]. [PubMed Central ID: PMC3957875]. https://doi.org/10.1128/AAC.00651-13.
-
25.
Elingold I, Isollabella MP, Casanova MB, Celentano AM, Perez C, Cabrera JL, et al. Mitochondrial toxicity and antioxidant activity of a prenylated flavonoid isolated from Dalea elegans. Chem Biol Interact. 2008;171(3):294-305. [PubMed ID: 18078919]. https://doi.org/10.1016/j.cbi.2007.10.005.