Abstract
Background:
Chalcogenide nanoparticles (NPs), such as silver selenide NPs (Ag2Se NPs), are used as quantum dots in pharmaceutical formulations for the detection of cancer cells, as well as in solar cells, sensors, and electrical, optical, and magnetic instruments.Objectives:
The physical-chemical production of metal NPs is expensive and difficult and pollutes the environment due to the use of dangerous chemicals and by-products. Hence, green chemistry-based processes must be developed as a reliable alternative. This study investigated indigenous aquatic fungal isolates for Ag2Se NP synthesis.Methods:
Visual examination, UV-Vis spectroscopy, scanning electron microscopy (SEM), dynamic light scattering (DLS) analysis, and energy-dispersive X-ray spectroscopy (EDX) tests confirmed the synthesis of Ag2Se NPs. Based on the characteristics of the colony, the microscopic features, and the polymerase chain reaction (PCR) amplification of the ITS1-5.8S-ITS2-ITS4 areas, the selected fungal isolate’s identity was established.Results:
The SEM analysis revealed that Cladosporium sp. ssf15 produced spherical Ag2Se NPs with an average diameter of 37.84 nm. The average size of NPs synthesized by the fungal isolate was determined to be 40.92 nm using the DLS analysis, and the polydispersity index (PDI) was determined to be 0.26. Based on the results, regular spherical Ag2Se NPs with correct dispersion distribution were produced using 2 mM AgNO3 and 1mM selenious acid (H2SeO3).Conclusions:
The results of this research have the potential to contribute to the development of a biocompatible approach and innovative research methods for investigating the green synthesis of Ag2Se NPs using fungal isolates.Keywords
Aquatic Fungus Cell-free Extract Cladosporium Silver Selenide Nanoparticle Spectroscopy
1. Background
The transition from microparticles to nanoparticles (NPs) involves several noteworthy modifications, including a dramatic increase in the surface area relative to volume and quantum phenomena (1). The increase in the surface-to-volume ratio, which occurs gradually as the particle size decreases, causes the behavior of the atoms on the particle’s surface to take precedence over the behavior of the internal atoms. This phenomenon affects the particle’s properties and its interaction with other materials; due to the significantly larger number of molecules or atoms on the surface compared to those in the sample’s mass, the particles tend to accumulate (2). Silver selenide (Ag2Se) is a semiconducting substance that occurs naturally as a mineral. With an optical band gap of 1.8 - 2.1 eV, this material belongs to compounds I - VI. As a semiconductor, Ag2Se can be used in a variety of applications, including infrared sensors, photolithography layers, electrochemical batteries, and electrochemical potential memory devices (3). Ag2Se NPs are chalcogenide NPs that are employed in solar cells, sensors, and electrical, optical, and magnetic instruments, as well as in pharmaceutical formulations such as quantum dots for cancer cell detection (4). Many physicochemical processes, including chemical reduction, electrochemistry, chemical deposition, thermal evaporation, and hydrothermal reaction, are used to create Ag2Se NPs. However, besides being expensive and requiring multiple steps, the physiochemical synthesis of the aforementioned NPs also pollutes the environment because of the usage of harmful chemicals and the creation of hazardous by-products. Thus, developing a green chemistry-based method is crucial as an effective and trustworthy substitute (5). Minerals can be synthesized by microorganisms, both unicellular and multicellular. Biosynthesis is a bottom-up strategy in which NPs are formed by the reduction/oxidation of metal ions via biological substances such as enzymes, carbohydrates, and proteins released by microorganisms. However, the inclination of microbes to behave differently and interact with various metal ions differs depending on the type of organism (6). In the last 2 decades, there has been little study on the synthesis of Ag2Se NPs using physicochemical approaches such as thermal degradation (7, 8) and coprecipitation (9), as well as biological methods based on the utilization of plant extracts (10). Fungi have distinct properties for the creation of metal NPs as compared to their biological equivalents. Fungi produce huge amounts of proteins and enzymes per unit of biomass, resulting in the extracellular synthesis of large numbers of NPs. Recent studies have revealed that fungi have a high intracellular metal absorption volume, and the produced particles are typically smaller (11).
2. Objectives
It is vital to develop methods based on green chemistry as an efficient and trustworthy alternative to the synthesis of NPs. It was attempted in this study to harness the potential of aquatic fungal isolates for the synthesis of Ag2Se NPs. This is the first study to report on the green synthesis of Ag2Se NPs in the Cladosporium fungus. Given the novelty of using Cladosporium sp. ssf15 fungus for extracellular synthesis of Ag2Se NPs via the cell-free extract (CFE) method, the findings of this study could be used as a biocompatible method in the field of green synthesis of Ag2Se NPs by other fungal and bacterial strains.
3. Methods
3.1. Sampling and Enrichment Conditions
Surface water samples from Saral Divandareh, Kurdistan Province, western Iran, were obtained from a depth of 15 to 30 cm. Water samples were collected in sterile containers and stored at 4°C in the laboratory until they were used. The collected water samples (25 mL) were transferred to sterile 45-mL falcon tubes and centrifuged at 5000g for 10 minutes. To screen fungal isolates, 500 µL of liquid was added to potato dextrose agar (PDA) culture media (200 g/L of potato infusion, 20 g/L of glucose, and 15 g/L of agar) supplemented with 0.5mM stocks of sodium selenite (Na2SeO3) and silver nitrate (AgNO3). Streptomycin, at a final concentration of 35 mg/L, was used as a selective agent to inhibit bacterial growth (12). The culture plates were incubated for 7 to 14 days at 25°C. On the PDA culture medium, fungal isolates were purified using the single spore and hyphal-tipping technique (13). The tolerance pattern of fungal isolates was examined by the agar dilution technique (14). To do this, 250-mL Erlenmeyer flasks containing 20 mL of PDA culture media were separately placed into 10-cm diameter Petri dishes with particular concentrations of Na2SeO3 and AgNO3 (1 mM, 2.5 mM, 5 mM, 5 mM, 7 mM, 10 mM, 12.5 mM, 15 mM, 17.5 mM, 20 mM, and 22.5 mM). To examine the tolerance of fungal isolates, a 5-mm disc was placed on the PDA culture medium at the edge of a 7- to 14-day culture of fungal isolates. The plates were examined after 2 weeks of incubation at 25°C in the dark. As a control, we used culture media incubated with fungal isolates without adding stock. All trials were conducted twice consecutively.
3.2. Isolation of Ag2Se NPs-Producing Fungi
The CFE technique was applied to the extracellular production of Ag2Se NPs (15). To prepare a CFE of fungal cells, fungal isolates with high tolerance to Na2SeO3 and AgNO3 were grown in potato dextrose broth (PDB) liquid culture medium in a shaker incubator set to 150 rpm at 25°C. Following 7 to 14 days of incubation, the mycelium was centrifuged (4000g for 30 minutes) and washed several times with sterile deionized water to purge the culture media. Then, under the same conditions, 10 g of the fungal biomass was added to 50 mL of sterile deionized water and incubated for 120 hours. Following this interval, the gained fungal extract was centrifuged and passed through 0.45-µm membrane filters to separate it from the fungal biomass. Ag2Se NPs were created using precursor salts and a coating agent. For this purpose, 50 mL of the CFE produced in 125 mL Erlenmeyer flasks was added. The conversion reaction mixture was then supplemented with silver nitrate stock at a concentration of 2 mM and sodium selenite stock at a concentration of 1 mM. Because the reaction is light sensitive, the inoculated Erlenmeyer flasks were kept in a shaker incubator for 48 hours at 25°C and 150 rpm. Meanwhile, control samples containing biological extracts but no stock salts were cultured under the same culture conditions (10). Visual observations and UV-Vis light spectroscopy were used to examine the characteristics of Ag2Se NPs produced in the conversion reaction mixture (16, 17).
3.3. Ag2Se NPs Characterization
The optical properties of Ag2Se NPs, such as absorbance and bandgap energy, were determined using UV-Vis spectroscopy (Specord 210, Germany). Field emission scanning electron microscopy (FESEM; TESCAN Mira 3-LMu, Czech Republic) was used to determine the size and shape of the NPs. It provides high-resolution photographs of individual particles and allows for the study of particle size distribution, size, and shape of NPs. Dynamic light scattering (DLS, SZ-100, HORIBA, Japan) was used to determine the hydrodynamic diameter of NPs in solution. The elemental composition of the NPs was determined using energy-dispersive X-ray spectroscopy (EDS; Mira 3-LMu). To precipitate and purify Ag2Se NPs produced by fungal extracts in the reaction mixture, the CFE was first filtered through 0.2-µm membrane filters, and then the samples were centrifuged at high speed (13000g for 30 minutes at 4°C). The precipitate was rinsed 3 times with sterile deionized water and 96% ethanol. A freeze dryer was used to dry the samples. The morphology, distribution size, and elemental content of the produced Ag2Se NPs were studied using a field emission scanning electron microscope equipped with X-ray energy diffraction spectroscopy. The images were taken using SEM and then processed using ImageJ software to build a histogram of particle size dispersion and estimate the average size of the NPs. Dynamic light scattering was used to detect the size of particles in liquids and suspensions ranging from several nanometers to several microns. An ultrasonic instrument was used to disperse the powdered samples of synthetic NPs in sterile distilled water until a homogeneous colloid was produced. The colloidal samples were then examined using a DLS machine to determine the average of NPs and the polydispersity index (PDI).
3.4. Isolate Characterization
The morphological examination was performed on cultures grown on PDA at 25°C for 14 days. After 14 days, colony diameters were measured, and mean colony growth was calculated. The color of the mycelia on the PDA and the development pattern were also documented. The genomic DNA of selected fungal isolates was extracted using the phenol/chloroform method and glass beads (18). The forward primer ITS1 (5-TCCGTAGGTGAACCTGCGG-3) and reverse primer ITS4 (5-TCCTCCGCTTGATATG-3) reported by White et al. were used for polymerase chain reaction (PCR) amplification (19). The purified PCR product was sequenced bidirectionally using an automated sequencer (Macrogen Inc, Seoul, Korea). The BLASTN software was used to match the sequences to identical sequences. The genome sequence was registered in GenBank using BankIt (a web-based application), and accession numbers were assigned.
4. Results
4.1. Biosynthesis of Ag2Se NPs Under Cell-free Extract Strategy
Using an enrichment cultivation technique in PDA culture media, 19 fungal isolates (named ssf1-ssf19) with different morphological characteristics were isolated and purified with sodium selenite and silver nitrate salts at a final concentration of 0.5 mM. Then, the tolerance pattern of fungal isolates was examined using the agar dilution method to get fungal isolates with high tolerance to sodium selenite oxyanion and silver nitrate salt. Based on the results, the tolerance of selected fungal isolates to sodium selenite oxyanion was determined to be between 1 mM and 20 mM and between 0.5 mM and 15 mM to silver nitrate salt. Among these, 4 fungal isolates (ssf8, ssf9, ssf13, and ssf15) with the highest resistance to selenite oxyanion (tolerance greater than 15 mM) and silver nitrate salt (tolerance greater than 10 mM) were chosen as superior fungal isolates for the biosynthesis of Ag2Se NPs. Visual inspection and UV-Vis light spectroscopy were used to assess the synthesis of Ag2Se NPs produced by the CFEs of selected fungal isolates. Visual observations revealed that after 48 hours of treating the CFEs of 4 fungal isolates (ssf8, ssf9, ssf13, and ssf15) with solutions of 2mM AgNO3 and 1mM selenious acid (H2SeO3), the color of the bioconversion reaction mixture changed from colorless to light brown (Figure 1A), showing the possible synthesis of Ag2Se NPs (20). A UV-Vis spectrometer was used to confirm the possible production of Ag2Se NPs in the colloidal solution. Figure 1B depicts the UV-Vis spectra obtained after treating the CFEs of the selected fungal isolates 48 hours after the reaction, which is consistent with the visual observations of the isolates. Fungi ssf8, ssf9, ssf13, and ssf15 showed unique absorption peaks at wavelengths ranging from 359 to 378 nm, indicating that Ag2Se NPs were present in the reaction mixture. According to reliable sources, the maximum absorption peak of Ag2Se NPs is between 359 and 385 nm (16). In the control solution (CFEs of fungal isolates not treated with selenious acid and silver nitrate stocks), there was no absorption peak at wavelengths between 300 and 400 nm.
(A) Visible observations (color changed from colorless to light brown) and (B) spectrophotometric absorption spectra of the cell-free extract of the selected fungal isolates (2mM silver nitrate and 1mM selenious acid) after 48 hours incubation on a shaker (150 rpm) at 25°C
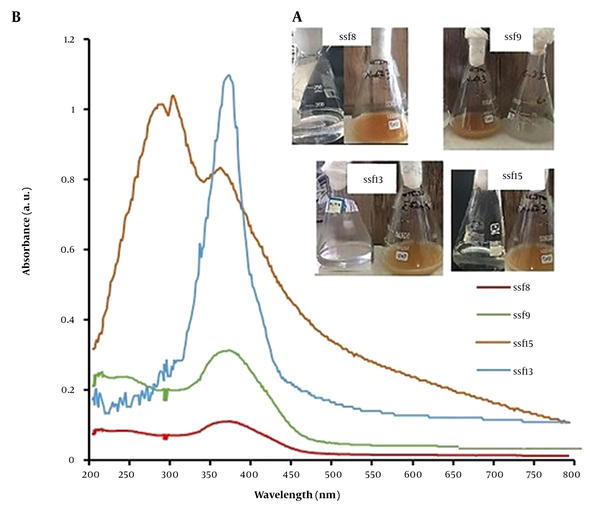
4.2. Validation of the Production of Ag2Se NPs by Microscopy and Spectroscopy Analyses
Fungal isolates ssf8, ssf9, ssf15, and ssf13 are based on the evaluation results of visual observations and UV-Vis spectroscopy. Since they could synthesize Ag2Se NPs, they were chosen for SEM observations. In the studied isolates, SEM micrograph images showed a uniform distribution of spherical and rod-shaped Ag2Se NPs ranging in size from 37 to 182 nm (Figure 2A, B, C, and 2D). Figure 3A depicts the SEM images and DLS analysis of Ag2Se NPs generated with CFE from isolate ssf15. As illustrated in the image, spherical NPs with a mean diameter of 40.92 nm and PDI of 0.26 were produced. The PDI for a homogenous colloidal sample of NPs is between 0.01 and 0.70. The PDI for non-uniform samples with improper dispersion is greater than 0.7. (21). Thus, the PDI of the Ag2Se NPs produced by fungal isolate ssf15 is appropriate. The SEM and DLS analyses of the surface structure of Ag2Se NPs produced by the fungal isolate ssf8 revealed the synthesis of spherical NPs with an average particle size of 59.69 nm, and their PDI was found to be 0.28 based on the results of the DLS study (Figure 2B). Ag2Se NPs synthesized by fungal isolate ssf9 revealed rod-shaped NPs with an average diameter of 169.51 nm, and PDI was 0.46 (Figure 2C). Then, SEM was used to analyze the morphology and size distribution range of the NPs generated by the CFE of fungal isolate ssf13. The synthesis of rod-shaped NPs with an average particle size of 191.66 nm and a PDI of 0.25 is presented in Figure 2D. Based on the findings, fungal isolate ssf15 was identified as the most suitable candidate for further analysis due to its favorable morphology and size, as well as its high monodispersity index. The effect of combined concentrations of the aforementioned stocks on the size and morphology of the Ag2Se NPs was explored using the CFE strategy to improve the biological reduction of selenious acid and silver nitrate stocks on Ag2Se NPs. The formation of spherical Ag2Se NPs with an average size of 26.6 nm and a PDI of 0.32 was reported in a reaction mixture containing 1mM AgNO3 and 0.5mM H2SeO3 (Figure 4A). In the presence of 2mM AgNO3 and 1mM H2SeO3, Ag2Se NPs with an average size of 37.8 nm and a PDI of 0.28 were identified (Figure 4B). Based on the results, Ag2Se NPs with uniform spherical morphologies and correct dispersion distribution were generated in the presence of 2mM AgNO3 and 1mM H2SeO3. Figure 3 depicts the energy-dispersive X-ray spectroscopy (EDX) analysis of Ag2Se NPs generated by fungal isolate ssf15 to evaluate constituent elements and product purity. The significant absorption peaks were associated with silver (70.37%) and selenium (29.63%), respectively, showing the creation of Ag2Se NPs as Ag2Se. The gained percentages, as observed, were compatible with the stoichiometric values of Ag2Se. The existence of minor oxygen and carbon absorption peaks is most likely due to trace levels of organic compounds in the fungal extract or coating agents involved in the stability of produced NPs.
Field emission scanning electron microscopy images and dynamic light scattering analysis of extracellular Ag2Se NPs produced under the strategy of cell-free extract isolates ssf15 (A), ssf8 (B), ssf9 (C), and ssf13 (D)
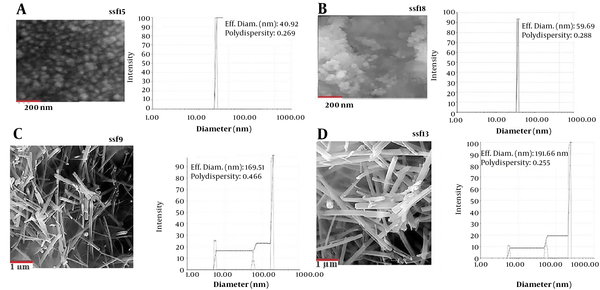
The energy-dispersive X-ray spectroscopy spectrum of Ag2Se NPs generated in a biotransformation reaction mixture, including 2 mM AgNO3 and 1mM H2SeO3 by the cell-free extract of fungal isolate ssf15
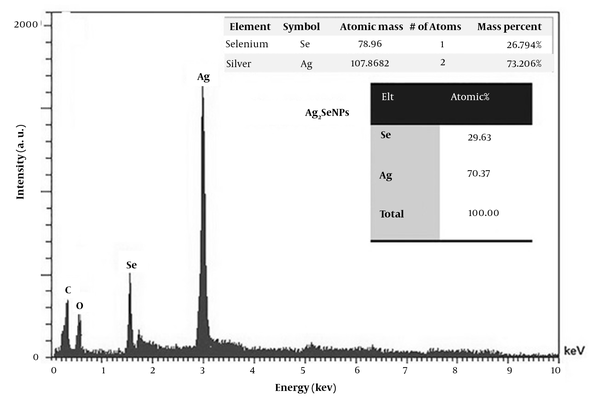
Field emission scanning electron microscopy images and a size distribution histogram of extracellular Ag2Se NPs synthesized using cell-free extract ssf15 in the presence of various amounts of silver nitrate and selenious acid precursor salts
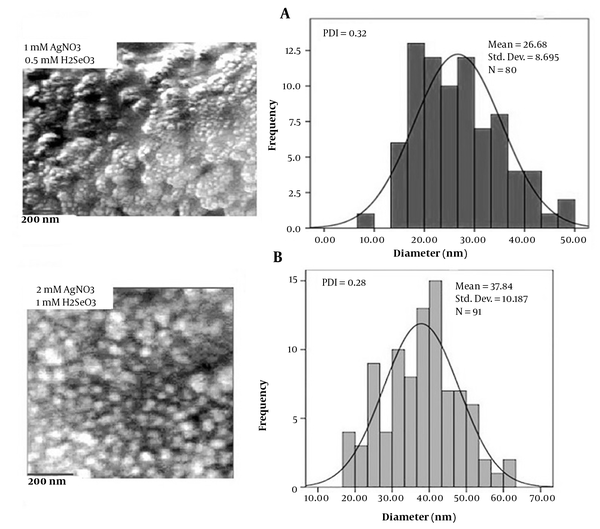
The effect of incubation time (24, 48, 72, 96, and 120 hours) was examined to improve the biological efficiency of Ag2Se NPs under the CFE of fungal isolate ssf15 in the reaction mixture containing 2 mM AgNO3 and 1mM H2SeO3 (Figure 5). The synthesis of Ag2Se NPs began after 24 hours of reaction and reached its peak efficiency after 96 hours. A steady decline in the absorbance spectrum of Ag2Se NPs was detected between 96 and 120 hours. The effect of incubation time (24, 48, 72, 96, and 120 hours) was examined to improve the biological efficiency of Ag2Se NPs under the CFE of fungal isolate ssf15 in the reaction mixture containing 2 mM AgNO3 and 1 mM H2SeO3 (Figure 5). The synthesis of Ag2Se NPs began after 24 hours of reaction and reached its peak efficiency after 96 hours. A steady decline in the absorbance spectrum of Ag2Se NPs was detected between 96 and 120 hours.
The effect of incubation time on the creation of Ag2Se NPs in a reaction involving the cell-free extract of fungal isolate ssf15 treated with 2 mM AgNO3 and 1mM H2SeO3 as measured by spectrophotometric absorption spectra
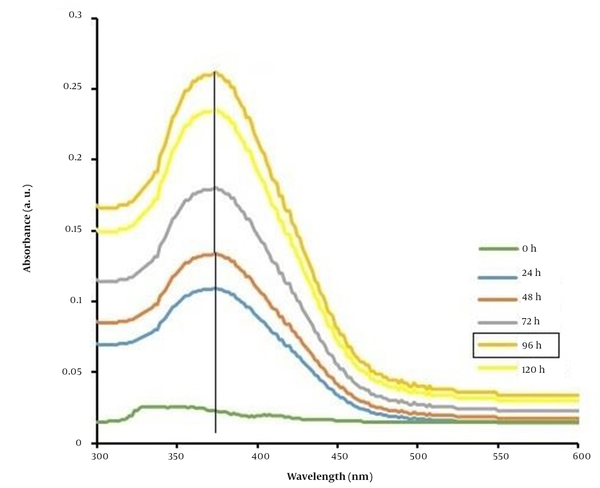
4.3. Characterization of Isolate ssf15
Based on the characteristics of the colony and microscopic features, the chosen fungal isolate was identified morphologically. After 14 days, the fungal colony on PDA culture media at 25°C has grown by 3.8 to 4.2 cm, which is visible as a black-green color. Conidia with dark pigment is produced, along with one to several cells that are organized into simple or branched chains. To precisely identify the chosen fungal isolate, the ITS1-5.8S-ITS2 sections were subsequently amplified by PCR using the universal ITS1 and ITS4 primers. Cladosporium sp. strain ssf15 was identified as isolate ssf15 (GenBank accession number OP242915) by BLAST analysis, which revealed 99% similarity with strains from the genus Cladosporium.
5. Discussion
Recent studies have revealed that the synthesis of NPs through green chemistry-based methods using microbes and plants is a safe, economical, and environmentally-friendly option Plants and microbes have long shown the ability to absorb and accumulate inorganic metal ions from their surroundings. Because of this characteristic, many microorganisms have effective biofactories capable of considerably reducing environmental pollution and recovering metals from industrial waste. To date, microbes’ capability to interact with, remove, and accumulate metal elements from their surroundings has been exploited in a variety of biotechnology applications, such as bioremediation and NP production (22). Fungi are currently used for the biosynthesis of nanoparticles because of their ability to tolerate toxins, vast storage capacity, effective biomass management, ease of manipulation and large-scale exploitation, and their capacity to produce a variety of compounds that support preservation and homeostasis. They may therefore thrive in harsh environments with poor nutrition and toxic substances (23). The major objectives of this study were to isolate and identify aquatic fungal isolates resistant to sodium selenite oxyanion and silver nitrate, as well as to produce Ag2Se NPs. In this context, the production of spherical Ag2Se NPs with an average size of 40.92 nm and a PDI of 0.26 was reported for the first time in fungal isolate ssf15 belonging to the genus Cladosporium under CFE conditions. The chemicals and substances excreted by the fungi outside the cell are used as an effective factor in the production of the desired NPs. Because of the release of reducing compounds from the microbial cell and their presence in the reaction mixture, this method enhances the production of NPs (15). Although several Cladosporium species have been described in previous studies because of the synthesis of Ag NPs (24), Au NPs (25), and ZnO NPs (26), this is the first study to describe the production of Ag2Se NPs in the genus Cladosporium. Throughout the last 2 decades, little research has been conducted on the synthesis of Ag2Se NPs, which has primarily relied on physicochemical and biological techniques based on plant extracts (10). Ng et al. were successful in synthesizing Ag2Se NPs via thermal degradation. In this approach, the precursor molecule (PPh3)3Ag2(SeC(50)Ph) was thermally decomposed in a solution containing trioctylphosphine and hexadecylamine for 2 hours at 180°C, and Ag2Se NPs were produced (7). Khanna et al. synthesized Ag2Se NPs by coprecipitation at 130°C for 5 hours using cycloheptane 1, 2, and 3-selenadiazole as a source of selenium and silver nitrate as a silver source in the presence of dimethylformamide as a solvent (9). Under nitrogen gas, Ag2Se NPs were produced using selenium powder as a source of selenium, silver nitrate as a source of silver, and trioctylphosphine, 1-octadecylamine, oleic acid, and 1-octadecine as surfactants. The disadvantage of this process is that it requires expensive materials, an enormous volume of raw materials, and a long reaction time (8). Mirzaei et al. explored the biological synthesis of Ag2Se nanochalcogens using an aqueous extract of the plant Melilotus officinalis (yellow alfalfa) with biological activity. The antibacterial, antibiotic, antioxidant, and cytotoxic properties of biosynthesized Ag2Se NPs were promising (10). In fact, a low-cost, non-toxic, and environmentally friendly synthesis technique based on fungal extracts for the synthesis of Ag2Se NPs with acceptable size and high monodispersity index was reported in this study.
5.1. Conclusions
Due to the tremendous diversity and environmental adaptability of aquatic fungal isolates, they can be exploited as potential biological nanofactories for the extracellular synthesis of different metal NPs. Cladosporium is one of the most common types of fungi, and its species have been used in the production of antibiotics, bioremediation, biofuels, and biopesticides. This species has been used in the nanobiotechnology field for the biosynthesis of metal NPs, such as silver, gold, and zinc oxide. The production of Ag2Se NPs in the genus Cladosporium was first documented in this study. This study’s approach is based on using an extract free of fungal cells and carefully selecting a solvent medium, environmental regenerating agent, and non-toxic materials to maintain the stability of NPs in the reaction medium. By following the principles of green chemistry, our method reduces environmental concerns, making it a promising option for numerous applications, particularly in the field of biomedicine.
References
-
1.
Zang H, Zhang X, Zhu B, Fatikow S. Recent advances in non-contact force sensors used for micro/nano manipulation. Sens Actuators A: Phys. 2019;296:155-77. https://doi.org/10.1016/j.sna.2019.07.007.
-
2.
Miyazaki K, Miyaji G. Nanograting formation through surface plasmon fields induced by femtosecond laser pulses. J Appl Phys. 2013;114(15). https://doi.org/10.1063/1.4826078.
-
3.
Ayele DW. A facile one-pot synthesis and characterization of Ag2Se nanoparticles at low temperature. Egypt j basic appl sci. 2019;3(2):149-54. https://doi.org/10.1016/j.ejbas.2016.01.002.
-
4.
Sibiya NP, Moloto MJ. Shape control of silver selenide nanoparticles using green capping molecules. Green Process Synth. 2017;6(2):183-8. https://doi.org/10.1515/gps-2016-0057.
-
5.
Liu YT, Gong PH, Xiao FQ, Shao S, Zhao DQ, Yan MM, et al. Chemical constituents and antioxidant, anti-inflammatory and anti-tumor activities of melilotus officinalis (Linn.) Pall. Molecules. 2018;23(2). [PubMed ID: 29382154]. [PubMed Central ID: PMC6017420]. https://doi.org/10.3390/molecules23020271.
-
6.
Shivaji S, Arvind M, Zygmunt S. Biosynthesis, optimization, purification and characterization of gold nanoparticles. Afr J Microbiol Res. 2014;8(2):138-46. https://doi.org/10.5897/ajmr10.143.
-
7.
Ng MT, Boothroyd C, Vittal JJ. Shape and size control of Ag2Se nanocrystals from a single precursor [(Ph3P)3Ag2(SeCOPh)2]. Chem Commun (Camb). 2005;(30):3820-2. [PubMed ID: 16041428]. https://doi.org/10.1039/b506203a.
-
8.
Sahu A, Qi L, Kang MS, Deng D, Norris DJ. Facile synthesis of silver chalcogenide (Ag2E; E=Se, S, Te) semiconductor nanocrystals. J Am Chem Soc. 2011;133(17):6509-12. [PubMed ID: 21486029]. https://doi.org/10.1021/ja200012e.
-
9.
Khanna PK, Singh N, Charan S, Patil KR. Synthesis of β-Ag2Se from organo-selenium precursor and silver nitrate in DMF via simultaneous reduction of selenium and silver salt. Materials Letters. 2006;60(17-18):2080-5. https://doi.org/10.1016/j.matlet.2005.12.079.
-
10.
Mirzaei SZ, Lashgarian HE, Karkhane M, Shahzamani K, Alhameedawi AK, Marzban A. Bio-inspired silver selenide nano-chalcogens using aqueous extract of Melilotus officinalis with biological activities. Biores Bioproc. 2021;8(1). https://doi.org/10.1186/s40643-021-00412-3.
-
11.
Narayanan KB, Sakthivel N. Biological synthesis of metal nanoparticles by microbes. Adv Colloid Interface Sci. 2010;156(1-2):1-13. [PubMed ID: 20181326]. https://doi.org/10.1016/j.cis.2010.02.001.
-
12.
Oladipo OG, Awotoye OO, Olayinka A, Bezuidenhout CC, Maboeta MS. Heavy metal tolerance traits of filamentous fungi isolated from gold and gemstone mining sites. Braz J Microbiol. 2018;49(1):29-37. [PubMed ID: 28844883]. [PubMed Central ID: PMC5790576]. https://doi.org/10.1016/j.bjm.2017.06.003.
-
13.
Nelson PE, Toussoun TA, Marassas WFO. Fusarium species: An illustrated manual for identification. New York: Pennsylvania State University Press, University Park; 1993. 193 p.
-
14.
Ashengroph M. Isolation and characterization of a native strain of Aspergillus niger ZRS14 with capability of high resistance to zinc and its supernatant application towards extracellular synthesis of zinc oxide nanoparticles. Biol J Microorganism. 2013;2(7):29-44.
-
15.
Bolbanabad EM, Ashengroph M, Darvishi F. Development and evaluation of different strategies for the clean synthesis of silver nanoparticles using Yarrowia lipolytica and their antibacterial activity. Process Biochem. 2020;94:319-28. https://doi.org/10.1016/j.procbio.2020.03.024.
-
16.
Delgado-Beleño Y, Martinez-Nuñez CE, Cortez-Valadez M, Flores-López NS, Flores-Acosta M. Optical properties of silver, silver sulfide and silver selenide nanoparticles and antibacterial applications. Mater Res Bull. 2018;99:385-92. https://doi.org/10.1016/j.materresbull.2017.11.015.
-
17.
Olawale F, Ariatti M, Singh M. Biogenic synthesis of silver-core selenium-shell nanoparticles using ocimum tenuiflorum l.: Response surface methodology-based optimization and biological activity. Nanomaterials (Basel). 2021;11(10). [PubMed ID: 34684956]. [PubMed Central ID: PMC8539562]. https://doi.org/10.3390/nano11102516.
-
18.
Hanna M, Xiao W. Isolation of nucleic acids. Yeast Protocol. 2006:15-20.
-
19.
White TJ, Bruns T, Lee S, Taylor J. Amplification and Direct Sequencing of Fungal Ribosomal Rna Genes for Phylogenetics. PCR Protocols. 1990. p. 315-22. https://doi.org/10.1016/b978-0-12-372180-8.50042-1.
-
20.
Garcia DA, Mendoza L, Vizuete K, Debut A, Arias MT, Gavilanes A, et al. Sugar-mediated green synthesis of silver selenide semiconductor nanocrystals under ultrasound irradiation. Molecules. 2020;25(21). [PubMed ID: 33171592]. [PubMed Central ID: PMC7664687]. https://doi.org/10.3390/molecules25215193.
-
21.
Barabadi H, Honary S. Biological synthesis of silver nanoparticles using standard fungus of Penicillium chrysogenum. Razi J Med Sci. 2014;21(122):20-8.
-
22.
Galucio JMP, de Souza SGB, Vasconcelos AA, Lima AKO, da Costa KS, de Campos Braga H, et al. Synthesis, characterization, applications, and toxicity of green synthesized nanoparticles. Curr Pharm Biotechnol. 2022;23(3):420-43. [PubMed ID: 34355680]. https://doi.org/10.2174/1389201022666210521102307.
-
23.
Ingale AG, Chaudhari AN. Biogenic synthesis of nanoparticles and potential applications: An eco-friendly approach. J Nanomed Nanotechol. 2013;4(165):1-7.
-
24.
Popli D, Anil V, Subramanyam AB, M NN, V RR, Rao SN, et al. Endophyte fungi, Cladosporium species-mediated synthesis of silver nanoparticles possessing in vitro antioxidant, anti-diabetic and anti-Alzheimer activity. Artif Cells Nanomed Biotechnol. 2018;46(sup1):676-83. [PubMed ID: 29400565]. https://doi.org/10.1080/21691401.2018.1434188.
-
25.
Munawer U, Raghavendra VB, Ningaraju S, Krishna KL, Ghosh AR, Melappa G, et al. Biofabrication of gold nanoparticles mediated by the endophytic Cladosporium species: Photodegradation, in vitro anticancer activity and in vivo antitumor studies. Int J Pharm. 2020;588:119729. [PubMed ID: 32768527]. https://doi.org/10.1016/j.ijpharm.2020.119729.
-
26.
Sumanth B, Lakshmeesha TR, Ansari MA, Alzohairy MA, Udayashankar AC, Shobha B, et al. Mycogenic Synthesis of Extracellular Zinc Oxide Nanoparticles from Xylaria acuta and Its Nanoantibiotic Potential. Int J Nanomedicine. 2020;15:8519-36. [PubMed ID: 33173290]. [PubMed Central ID: PMC7646447]. https://doi.org/10.2147/IJN.S271743.