Abstract
Keywords
1. Context
The Hippo signaling pathway (HSP), a conserved signaling pathway through evolution, plays critical roles in controlling organ size, tissue regeneration, and tumor suppression (1, 2). The YES-associated protein (YAP) and transcriptional coactivator with PDZ-binding motif (TAZ) are involved in HSP as transcriptional coactivators (3). Upon activation, YAP and TAZ initiate a transcriptional program promoting cell proliferation and enhancing stem cell self-renewal, which are essential for tissue regeneration stimulation. However, aberrant-activated YAP and TAZ can lead to malignant tumor formation (4). Here, we review the updates of the HSP in stem cell biology and its potential therapeutic targets.
2. Hippo Signaling Pathway
The HSP contains a highly conserved cascade of serine/threonine kinases, which negatively regulate the YAP and TAZ expression and activity. In mammals, the core members involved in the pathway include mammalian STE20-like protein kinase 1 (MST1) and MST2 and large tumor suppressor 1 (LATS1) and LATS2 (5, 6). These kinases' activity also depends on their interactions with different scaffolding proteins. Salvador homolog 1 (SAV1) forms complexes with MST1/2, while MOB kinase activator 1A (MOB1A) and MOB1B interact with LATS1/2 (7-9). After the HSP activation, MST1/2 phosphorylates and activates LATS1/2 and MOB1A/1B (10, 11). Then, the YAP and TAZ HXRXXS motifs are directly phosphorylated by LATS1/2 (12). Two main phosphorylation mechanisms inhibit YAP and TAZ activity. First, a binding site for 14-3-3 is created by phosphorylating YAP (on serine 127) and TAZ (on serine 89). The 14-3-3 complex promotes YAP and TAZ sequestration in the cytoplasm (13, 14). In the second mechanism, YAP serine 381 and TAZ serine 311 are phosphorylated, promoting casein kinase-1 mediated phosphorylation. The extra phosphorylation induces a motif targeted by β-transducin repeat-containing protein (β-TrCP), which results in YAP and TAZ degradation (15). In the nucleus, the accumulated YAP and TAZ promote the expression of specific genes when the HSP is deactivated (Figure 1). There is no DNA-binding domain in YAP and TAZ. They regulate the expression of the target genes by forming complexes with other transcription factors. In mammals, YAP and TAZ frequently bind to TEA domain transcription factors (TEAD1 to TEAD4). Studies reported interactions between other transcription factors, including p73, T-box transcription factor 5 (TBX5), and RUNT-related transcription factor 1 (RUNX1), RUNX2 with YAP/TAZ (16).
Hippo signaling pathway. The schematic diagram for the Hippo pathway's core components and signal transduction. A, when Hippo signaling is on (in high cell density), unknown upstream signaling leads to the activation of mammalian STE20-like protein kinase 1 (MST1)/2. Activated MST1/2 phosphorylate Salvador homolog 1 (SAV1), which, in turn, phosphorylates large tumor suppressor (LATS) and MOB kinase activator 1 (MOB1). The activated LATS/MOB phosphorylates YES-associated protein (YAP)/transcriptional coactivator with PDZ-binding motif (TAZ), resulting in cytoplasmic retention by the 14-3-3 protein and proteasomal degradation of YAP/TAZ; B, when Hippo signaling is off (in low cell density), the kinases MST1/2 and LATS are inactive, which inhibits the phosphorylation of YAP and TAZ. The stabilized YAP/TAZ in nuclei interacts with TEA domain transcription factor (TEAD) and enhances the transcription of target genes related to anti-apoptosis and proliferation.
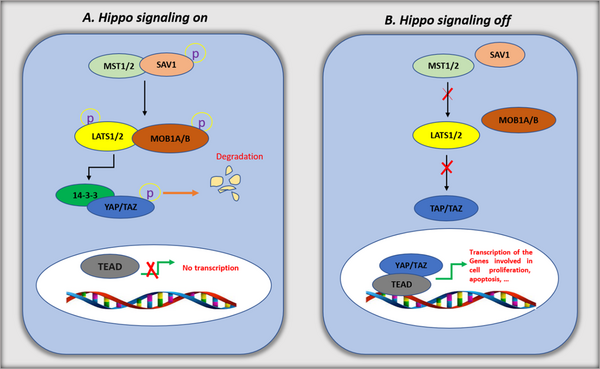
Recent evidence reported that the extracellular matrix and neighboring cells regulate YAP/TAZ through mechanical cues (17-19). Moreover, it was indicated that various extracellular hormones, including epinephrine, glucagon, lysophosphatidic acid (LPA), and thrombin, potently regulate the HSP (17, 20). It seems that the actin cytoskeleton mediates these mechanical and hormonal cues. Mechanically, F-actin stability results in YAP/TAZ activation, whereas F-actin disruption results in YAP/TAZ deactivation (21, 22). The transduction of extracellular hormonal cues through the actin cytoskeleton and RHO GTPases by G-protein-coupled receptors (GPCRs) modulates YAP/TAZ. The YAP/TAZ activity is simulated or inhibited by GPCR signaling. For example, YAP/TAZ is stimulated by G12/13 or Gq/11 activation and inhibited by Gs (20, 23, 24). Many other extra proteins have been reported to regulate the HSP beyond the main members of the HSP described above, including thousand-and-one amino acid protein kinases (TAOK1-3) (25), MAP/microtubule affinity-regulating kinases (MARK1-4) (26, 27), salt-inducible kinases (SIK1-3) (28), RAS association domain-containing family protein (RASSF) (29), multiple ankyrin repeats single KH domain-containing protein (MASK) (30), and homeodomain-interacting protein kinase 2 (HIPK2) (31).
3. Hippo Signaling Pathway in Embryogenesis and Embryonic Stem Cells
The HSP is the critical pathway that limits tissue and organ growth (4). Human embryonic stem cells (hESCs) are characterized by their capacity to self-renew and maintain pluripotency in the culture and are derived from the inner cell mass of the blastocyst. The first cell fate specification occurs during development between the morula and blastocyst stages, before the embryo's implantation into the uterus (preimplantation). The morula's outer cells get apicobasal polarity and differentiate into a cyst-like epithelial tissue in the blastocyst known as trophectoderm (TE). The TE is necessary for implantation and builds the placental tissues afterward. The inner cell mass (ICM), a collection of cells adhering to one side of the TE, comprises the nonpolar inner cells. The embryo proper and various extraembryonic tissues are produced by the ICM (32, 33). The specification of TE and ICM cell fates is regulated by the HSP. In the outer cells, it is dormant, allowing YAP to build up in the nucleus. When TEAD4 and nuclear YAP bind together, TEAD4 becomes a transcriptional activator. Cdx2 and Gata3 are transcription factors specific to TEs activated by the TEAD4-YAP complex, driving TE development (34-37). Hippo signaling pathway is active in the inner cells, which prevents YAP from building up in the nucleus. Through TEAD4 inactivation, nuclear YAP deficiency limits the production of transcription factors unique to TE (38). This permits the high-level production of the transcription factors Oct3/4, Nanog, and Sox2, which encourage the differentiation of cells into the ICM and are likely auto-activation mechanisms (32) (Figure 2).
Differential Hippo signaling specifies distinct cell fates in the preimplantation embryo cell. The inner cells activate Hippo signaling, allowing the inner cells to express inner cell mass (ICM)-specific transcription factors and adopt the ICM fate. Hippo signaling is suppressed in the outer cells, promoting the differentiation of outer cells to trophectoderm (TE).
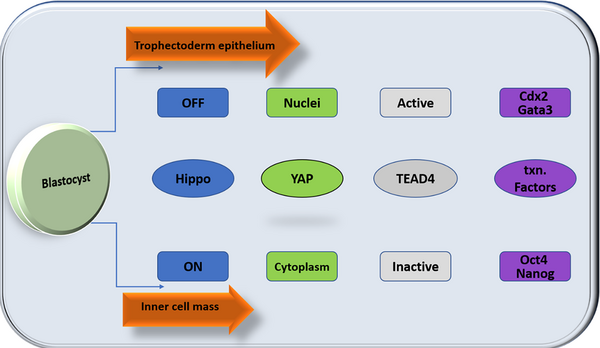
Recent research on YAP and TAZ has revealed that transcriptional coactivators control embryonic stem cell (ESC) self-renewal and differentiation (39). A study relating HSP to ESC biology found that TAZ controls the location of transcriptional regulators SMAD2/3-4, which mediate tissue growth factor (TGF)-signaling. Transcriptional coactivator with PDZ-binding motif binds SMAD2/3-4 proteins to encourage nuclear agglomeration and coupling to the mediator complex, enhancing their transcriptional activity after TGF stimulation. According to two studies published more recently, YAP is inactivated during (mouse) mESC development, and YAP or TEAD knock-down results in loss of pluripotency (40, 41). On the other hand, when it is ectopically overexpressed in induced pluripotent stem cells (iPSCs), activated YAP improves reprogramming effectiveness and prevents differentiation in mESCs (40). These investigations also discovered that YAP-TEAD stimulates the transcription of recognized stemness genes in mESCs but not mature cells, including Oct3/4, Sox2, Nanog, BMP signaling, and LIF targets. These results are consistent with the hypothesis that YAP/TAZ mediates BMP/TGF transcriptional activity and directly increases the expression of essential stemness genes to maintain ESC pluripotency in vitro (42, 43).
4. Hippo Signaling and Somatic Stem Cells
4.1. Liver Progenitor Cells
The HSP has a crucial role in different somatic stem cells. The most significant metabolic organ, the liver, has an important capability for regeneration. Even a 70% hepatectomy can lead to regeneration. Hepatocytes, the primary cell type in the adult liver, do not undergo mitosis. The liver has oval cells (OCs) that can produce a transit precursor compartment, but hepatocyte proliferation is necessary for the liver to regenerate. Although liver regeneration has long been understood, the underlying mechanisms and the method by which the liver determines when it has grown back to its original size are still unknown (44, 45).
Historic studies verified the HSP's physiological significance in mammalian liver using mouse models that conditionally overexpress YAP in hepatocytes (46). The postnatal liver underwent significant but reversible liver hyperplasia due to YAP activation, which resulted in a four-fold increase in the total mass of the organ. The primary cellular factor in hyperplasia was the excessive proliferation of mature hepatocytes. These results provide evidence that a human homolog of the Drosophila HSP can affect tissue size and provide the foundation for further research along this line. Other HSP elements have recently been postulated to inhibit liver development (42). After Mst1/2 deletion, mice developed liver tumors that exhibited traits of both cholangiocarcinoma (CC) and hepatocellular carcinoma (HCC), proving that these tumors originated from bipotential liver progenitor cells (47, 48). Cell lines generated from MST1/2 null livers experienced growth inhibition and extensive apoptosis upon YAP depletion (49, 50). These findings support the theory that the primary cause of liver enlargement associated with MST1/2 deficiency is YAP activation. These results suggest that HSP components may significantly regulate organ size and hepatocyte quiescence in animals. However, their dysregulation can result in stem cell proliferation, enlargement, and cancer via several mechanisms. The phenotypes observed in mice models with conditional deletion of various HSP components differ. Consequently, more research is required to thoroughly understand the functions of these HSP components in the regulation of liver progenitor cells, as well as their modes of action.
4.2. Neural Progenitor Cells
Neural progenitor cells are distributed throughout the ventricular zone of the neural tube in developing vertebrates. They produce several cell types that constitute the mature central nervous system (CNS) (51, 52). The YAP protein colocalizes with Sox2 and is expressed in mouse, frog, and chick neural tube progenitor zones (53). A significant increase in neural progenitors is caused by Mst1/2 or Lats1/2 loss, or YAP-TEAD activation, partly due to the overexpression of cell cycle re-entry and stemness genes and a barrier to differentiation by inhibiting critical genes. On the other hand, YAP deficiency increases cell death and premature neuronal differentiation (42, 54). In the perivascular niche of the cerebellum, endogenous YAP is present in high concentrations in cerebellar granule neural precursors (CGNPs) and tumor-repopulating cancer SCs (55, 56). High levels of YAP are also expressed in this area of the brain by an increase in cells with an undifferentiated CGNP phenotype, such as medulloblastomas, which are common in children (57, 58). Since the sonic hedgehog (Shh) pathway is activated in human medulloblastomas and CGNPs depend on Shh signaling to proliferate, the relationship between the Hippo and Shh pathways was investigated (59, 60). Shh signaling in CGNPs causes the expression and nuclear localization of YAP, and YAP then encourages the development of these cells. Recent research points to a new model of the brain in which YAP acts as a nexus between neural stem cell (NSC) proliferative pathways, including Notch and Shh (and maybe others), which were previously believed to cooperate to regulate brain development, to enhance NSC proliferation (61).
4.3. Skin Stem Cells
Animals' largest organ, the skin, is a barrier against environmental dangers and maintains the body's moisture. The self-renewal ability of epidermal SCs (eSCs) at the basal layer allows the skin to regenerate and continuously maintain its structural and functional integrity. Short-lived progenitor cells that stratify leave the basal layer and ascend upward via the suprabasal layers to the organ's surface are the product of asymmetric divisions in this SC compartment during terminal development (60, 62). In recent investigations, the significance of YAP in epidermal development and SC homeostasis has been emphasized (62-64). Two research groups demonstrated that YAP activation significantly thickens the epidermal layer using a mouse model with skin-inducible YAP expression. Surprisingly, this hyperplasia is fueled by the expansion of undifferentiated interfollicular stem and progenitor cells (65). Colony-formation tests showed that larger cells exhibited higher levels of clonogenic activity and a longer self-renewal period. However, skin-specific YAP deletion or genetic ablation of the YAP-TEAD interaction during epidermal development resulted in epidermal hypoplasia and failure of skin growth (65). Unexpectedly, a genetic investigation found that the classical Hippo kinases do not control the skin's YAP. A downstream negative regulator of YAP was shown to be -catenin, a part of adherens junctions (AJs). Based on the massive overgrowth symptoms produced by -catenin loss in the developing brain and skin, it was hypothesized that AJs could function as molecular biosensors of cell density and position (66, 67). This notion is confirmed and expanded upon by genetic and functional findings relating to YAP and -catenin, suggesting that YAP is a significant mediator of density regulation in the epidermis. In this paradigm, increased cellular density prevents SC expansion by inactivating YAP. When this molecular network is disturbed, hyperproliferation and cancer might develop.
4.4. Cancer Stem Cells
The HSP is crucial in controlling organ size and carcinogenesis by inhibiting cell proliferation, inducing apoptosis, and controlling the growth of stem/progenitor cells (57, 58, 68). The nucleus contains unphosphorylated YAP/TAZ, encouraging tumor and cell proliferation. The cytoplasm is the central location of phosphorylated YAP/TAZ, which prevents tumor growth. Numerous malignant cancers are associated with elevated YAP/TAZ expression and activity (69-71). Breast cancer stem cells (CSCs) have also been discovered to be significantly regulated by TAZ (72). Additionally, highly expressed in medulloblastoma CSCs are YAP and TEAD (73). CSCs, a tiny subset of tumor cells that can self-renew, differentiate into different tumor cell types, and cause tumor growth, have recently been associated with tumor development. It is believed that CSCs resistant to chemotherapeutic agents are to blame for cancer spread and recurrence. Numerous human malignancies, including liver, lung, colorectal, ovarian, and prostate cancers, have been linked to HSP dysregulation (74-78). Studies have shown that higher expression and nuclear localization of YAP results in enhanced activity in human tumor tissues. This is in line with the inhibition of the HSP, which is known to restrict the function of YAP and TAZ by encouraging their cytoplasm localization and eventual degradation by ubiquitin. In numerous mouse and human tumor models, the YAP gene has also been found to be amplified (58, 79-81). These results suggest that unchecked YAP activation can circumvent conventional tumor suppressor checkpoints. Compared to other well-known carcinogenic signaling pathways, the direct mutation of an HSP component has only occasionally been associated with malignancies (4). According to numerous investigations, the HSP is crucial in human cancer. Inhibiting YAP/TAZ could be a potential treatment strategy for malignancies with imbalanced HSP. According to a recent study, HSP controls the YAP and TAZ transcriptional networks in mammalian systems. YAP1/TAZ transcriptional output downregulation revealed that prostate-derived ETS factor (PDEF), a tumor metastasis suppressor, positively regulates HSP. These results might provide a fresh avenue for treating prostate cancer (Figure 3) (8, 82).
Role of the Hippo signaling pathway in somatic stem cells
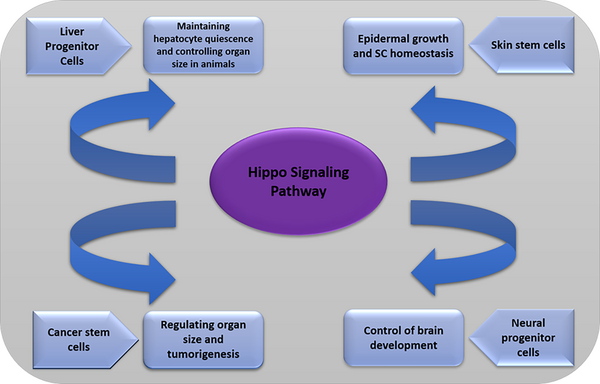
5. Drug Resistance
The most prevalent cancer treatments at present are chemotherapy and targeted therapy. Sadly, traditional treatments frequently fail to eradicate cancer cells that develop into CSCs, allowing for clinical recurrence caused by CSCs. Chemotherapeutic resistance is present in tumor cells with increased YAP/TAZ activity, partly due to the CSC characteristics brought on by YAP/TAZ activation. Prostate epithelial cells are transformed in vivo by YAP activation into androgen-insensitive cells with castration resistance (83). Recent research has revealed that miR-302/367-mediated LATS2 inhibition causes YAP activation and confers CSC status in prostate cancer cells (84). Metastatic activity and drug resistance to chemotherapeutic drugs, such as paclitaxel and doxorubicin, have been linked to YAP/TAZ activation in breast CSCs (72, 85). Numerous studies have shown that blocking the HSP or activating YAP/TAZ in various tumor types increases cancer cell survival against DNA-damaging medicines, including cisplatin, taxol, and fluorouracil (5-FU) (86-88). Additionally, YAP/TAZ promotes resistance to targeted therapy. A severe clinical issue is that YAP increases treatment resistance to agents that target RAF and MEK in tumor cells with BRAF, KRAS, or NRAS mutations (89). Actin remodeling is one way YAP becomes resistant to BRAF inhibitors in melanoma cells (90). Importantly, the YAP/TAZ-TEAD target genes of the secreted ligands play a role in drug resistance. Connective tissue growth factor (CTGF) promotes chemotherapeutic drug resistance in glioblastoma multiforme (GBM), breast cancer, and osteosarcoma (91). These results imply that non-cell autonomous processes may target YAP/TAZ-induced secreted ligands more effectively.
6. Pharmacological Interventions
A leading cause of death worldwide is cancer. Cancer stem cells that have undergone EMT can metastasize and pass on drug resistance. The Hippo-YAP/TAZ pathway has become a potent oncogenic driver in cancer cells with CSC characteristics. For small-molecule treatments, protein kinases, which function as oncogenes, are an excellent target. Sadly, because MST1/2 and LATS1/2 (the Hippo core kinases) are tumor suppressors, researchers have been seeking other therapeutic modalities (Figure 4). The most significant inhibitors of the Hippo-YAP/TAZ pathway are covered below.
Therapeutic targeting of the Hippo pathway. This figure shows the targeting strategies of YES-associated protein (YAP)/transcriptional coactivator with PDZ-binding motif (TAZ) activity in the Hippo pathway. Dasatinib, ROCK inhibitors, and statins represent substances that inhibit the activity of YAP/TAZ by activating large tumor suppressor (LATS). Rapamycin and metformin inhibit LATS-independent YAP/TAZ activity. Verteporfins represent substances that inhibit the interaction between YAP/TAZ and TEA domain transcription factor (TEAD).
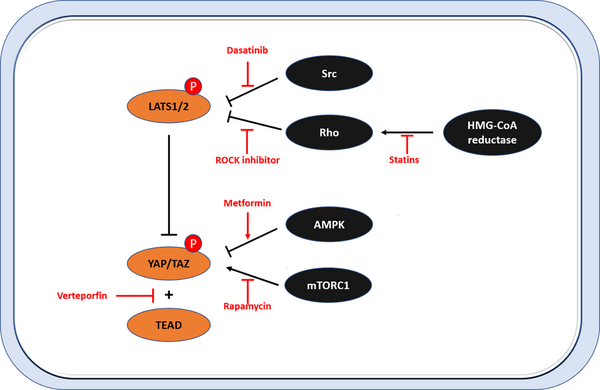
7. Verteporfin
Verteporfin, a US Food and Drug Administration (FDA)-approved photosensitizer for macular degeneration, is the most often utilized YAP inhibitor in research institutions (92, 93). It reduces YAP-TEAD-induced target gene transcription by blocking YAP-TEAD binding or increasing 14-3-3 expression (94). Verteporfin suppresses YAP-induced liver carcinogenesis and uveal melanoma in living organisms, pointing to the therapeutic benefit of cutting off the YAP/TAZ-TEAD linkages in cancer cells (67, 95). It works against tumors by directly attaching to YAP. It also has a cytotoxic effect independent of YAP, suggesting that its impact on YAP activity should be examined cautiously (96).
8. Statins
Through FDA-approved drug library screening for drugs that result in YAP/TAZ cytoplasmic translocation in breast cancer cells, statins were found to be potent YAP inhibitors (97, 98). By blocking 3-hydroxy-3-methylglutaryl coenzyme A (HMG-CoA) reductase, statins help people with hypercholesterolemia lower their cholesterol levels. Geranylgeranyl pyrophosphate synthesis, vital for appropriate Rho GTPase activity, is decreased when the HMG-CoA to mevalonate conversion is inhibited. Therefore, statin medication inhibits Rho GTPases, which reduces YAP/TAZ (97, 99).
9. LATS Kinase Inhibitors
In mouse models and human cell lines, the knocking-out of NF2, Sav1, MST1/2, and Mob1 causes cancer development, demonstrating the tumor-suppressive function of the Hippo core kinases (1). Due to the lack of small-molecule kinase activators, Hippo kinases are still challenging to target. The LATS1/2 kinase, however, was recently found to serve an unexpected role in obstructing anti-tumor immunity (100). By inhibiting type I interferon response mediated by the toll-like receptors-MYD88/TRIF pathway, LATS1/2 lowers the antitumor immune response. Inhibition of upstream Hippo kinases, either alone or in combination with immune checkpoint drugs, maybe a potential strategy for suppressing tumor growth. Drugs that target the HSP may aid in tissue regeneration and wound repair in addition to having anticancer properties.
10. Conclusions
Although most HSP components were initially identified in Drosophila, recent human and animal model studies have demonstrated the pathway's crucial function in stem cell development, cancer genesis, and tissue homeostasis. As essential downstream effectors of the HSP, YAP/TAZ is involved in embryonic stem cells, tissue-specific stem cell self-renewal, tissue regeneration, and homeostasis in the liver, colon, pancreas, heart, skin, and CNS. There is also strong evidence that YAP/TAZ contributes to developing cancer stem cell traits. Therefore, elements of the HSP might be promising therapeutic targets for diseases such as cancer and degeneration. Oncogenic driver mutations in the main parts of the HSP are infrequent despite their significance in cancer development. Finding the CSC-specific YAP/TAZ oncogenic signaling network will, therefore, open up new avenues for eliminating CSCs and treating cancer progression.
References
-
1.
Yu FX, Zhao B, Guan KL. Hippo Pathway in Organ Size Control, Tissue Homeostasis, and Cancer. Cell. 2015;163(4):811-28. [PubMed ID: 26544935]. [PubMed Central ID: PMC4638384]. https://doi.org/10.1016/j.cell.2015.10.044.
-
2.
Khalilian S, Hojati Z, Dehghanian F, Shaygannejad V, Imani SZH, Kheirollahi M, et al. Gene expression profiles of YAP1, TAZ, CRB3, and VDR in familial and sporadic multiple sclerosis among an Iranian population. Sci Rep. 2021;11(1):7713. [PubMed ID: 33833274]. [PubMed Central ID: PMC8032816]. https://doi.org/10.1038/s41598-021-87131-z.
-
3.
Battilana G, Zanconato F, Piccolo S. Mechanisms of YAP/TAZ transcriptional control. Cell Stress. 2021;5(11):167-72. [PubMed ID: 34782888]. [PubMed Central ID: PMC8561301]. https://doi.org/10.15698/cst2021.11.258.
-
4.
Calses PC, Crawford JJ, Lill JR, Dey A. Hippo Pathway in Cancer: Aberrant Regulation and Therapeutic Opportunities. Trends Cancer. 2019;5(5):297-307. [PubMed ID: 31174842]. https://doi.org/10.1016/j.trecan.2019.04.001.
-
5.
Ma S, Meng Z, Chen R, Guan KL. The Hippo Pathway: Biology and Pathophysiology. Annu Rev Biochem. 2019;88:577-604. [PubMed ID: 30566373]. https://doi.org/10.1146/annurev-biochem-013118-111829.
-
6.
Yan F, Qian M, He Q, Zhu H, Yang B. The posttranslational modifications of Hippo-YAP pathway in cancer. Biochim Biophys Acta Gen Subj. 2020;1864(1):129397. [PubMed ID: 31306710]. https://doi.org/10.1016/j.bbagen.2019.07.006.
-
7.
Misra JR, Irvine KD. The Hippo Signaling Network and Its Biological Functions. Annu Rev Genet. 2018;52:65-87. [PubMed ID: 30183404]. [PubMed Central ID: PMC6322405]. https://doi.org/10.1146/annurev-genet-120417-031621.
-
8.
Mohajan S, Jaiswal PK, Vatanmakarian M, Yousefi H, Sankaralingam S, Alahari SK, et al. Hippo pathway: Regulation, deregulation and potential therapeutic targets in cancer. Cancer Lett. 2021;507:112-23. [PubMed ID: 33737002]. [PubMed Central ID: PMC10370464]. https://doi.org/10.1016/j.canlet.2021.03.006.
-
9.
Gundogdu R, Hergovich A. MOB (Mps one Binder) Proteins in the Hippo Pathway and Cancer. Cells. 2019;8(6):569. [PubMed ID: 31185650]. [PubMed Central ID: PMC6627106]. https://doi.org/10.3390/cells8060569.
-
10.
Rawat SJ, Chernoff J. Regulation of mammalian Ste20 (Mst) kinases. Trends Biochem Sci. 2015;40(3):149-56. [PubMed ID: 25665457]. [PubMed Central ID: PMC4340714]. https://doi.org/10.1016/j.tibs.2015.01.001.
-
11.
Dehghanian F, Hosseini Imani SZ, Naghsh-Nilchi A, Khalilian S. Hippo Signaling Pathway and Cancer: Molecular Regulation and Therapeutic Targets. Precis Med Clin OMICS. 2022;2(1):e124241. https://doi.org/10.5812/pmco-124241.
-
12.
Guo L, Teng L. YAP/TAZ for cancer therapy: opportunities and challenges (review). Int J Oncol. 2015;46(4):1444-52. [PubMed ID: 25652178]. https://doi.org/10.3892/ijo.2015.2877.
-
13.
Zhou X, Lei QY. Regulation of TAZ in cancer. Protein Cell. 2016;7(8):548-61. [PubMed ID: 27412635]. [PubMed Central ID: PMC4980330]. https://doi.org/10.1007/s13238-016-0288-z.
-
14.
Abylkassov R, Xie Y. Role of Yes-associated protein in cancer: An update. Oncol Lett. 2016;12(4):2277-82. [PubMed ID: 27698789]. [PubMed Central ID: PMC5038596]. https://doi.org/10.3892/ol.2016.4955.
-
15.
Warren JSA, Xiao Y, Lamar JM. YAP/TAZ Activation as a Target for Treating Metastatic Cancer. Cancers (Basel). 2018;10(4):115. [PubMed ID: 29642615]. [PubMed Central ID: PMC5923370]. https://doi.org/10.3390/cancers10040115.
-
16.
Pocaterra A, Romani P, Dupont S. YAP/TAZ functions and their regulation at a glance. J Cell Sci. 2020;133(2):jcs230425. [PubMed ID: 31996398]. https://doi.org/10.1242/jcs.230425.
-
17.
Totaro A, Panciera T, Piccolo S. YAP/TAZ upstream signals and downstream responses. Nat Cell Biol. 2018;20(8):888-99. [PubMed ID: 30050119]. [PubMed Central ID: PMC6186418]. https://doi.org/10.1038/s41556-018-0142-z.
-
18.
Kim MK, Jang JW, Bae SC. DNA binding partners of YAP/TAZ. BMB Rep. 2018;51(3):126-33. [PubMed ID: 29366442]. [PubMed Central ID: PMC5882219]. https://doi.org/10.5483/bmbrep.2018.51.3.015.
-
19.
Ferraiuolo M, Verduci L, Blandino G, Strano S. Mutant p53 Protein and the Hippo Transducers YAP and TAZ: A Critical Oncogenic Node in Human Cancers. Int J Mol Sci. 2017;18(5):961. [PubMed ID: 28467351]. [PubMed Central ID: PMC5454874]. https://doi.org/10.3390/ijms18050961.
-
20.
Luo J, Yu FX. GPCR-Hippo Signaling in Cancer. Cells. 2019;8(5):426. [PubMed ID: 31072060]. [PubMed Central ID: PMC6563442]. https://doi.org/10.3390/cells8050426.
-
21.
Zanconato F, Battilana G, Cordenonsi M, Piccolo S. YAP/TAZ as therapeutic targets in cancer. Curr Opin Pharmacol. 2016;29:26-33. [PubMed ID: 27262779]. [PubMed Central ID: PMC6175256]. https://doi.org/10.1016/j.coph.2016.05.002.
-
22.
Seo J, Kim J. Regulation of Hippo signaling by actin remodeling. BMB Rep. 2018;51(3):151-6. [PubMed ID: 29353600]. [PubMed Central ID: PMC5882222]. https://doi.org/10.5483/bmbrep.2018.51.3.012.
-
23.
Santinon G, Pocaterra A, Dupont S. Control of YAP/TAZ Activity by Metabolic and Nutrient-Sensing Pathways. Trends Cell Biol. 2016;26(4):289-99. [PubMed ID: 26750334]. https://doi.org/10.1016/j.tcb.2015.11.004.
-
24.
Zindel D, Mensat P, Vol C, Homayed Z, Charrier-Savournin F, Trinquet E, et al. G protein-coupled receptors can control the Hippo/YAP pathway through Gq signaling. FASEB J. 2021;35(7):e21668. [PubMed ID: 34114695]. https://doi.org/10.1096/fj.202002159R.
-
25.
Plouffe SW, Meng Z, Lin KC, Lin B, Hong AW, Chun JV, et al. Characterization of Hippo Pathway Components by Gene Inactivation. Mol Cell. 2016;64(5):993-1008. [PubMed ID: 27912098]. [PubMed Central ID: PMC5137798]. https://doi.org/10.1016/j.molcel.2016.10.034.
-
26.
Davis JR, Tapon N. Hippo signalling during development. Development. 2019;146(18):dev167106. [PubMed ID: 31527062]. [PubMed Central ID: PMC7100553]. https://doi.org/10.1242/dev.167106.
-
27.
Richardson HE, Portela M. Tissue growth and tumorigenesis in Drosophila: cell polarity and the Hippo pathway. Curr Opin Cell Biol. 2017;48:1-9. [PubMed ID: 28364663]. https://doi.org/10.1016/j.ceb.2017.03.006.
-
28.
Sun Z, Jiang Q, Li J, Guo J. The potent roles of salt-inducible kinases (SIKs) in metabolic homeostasis and tumorigenesis. Signal Transduct Target Ther. 2020;5(1):150. [PubMed ID: 32788639]. [PubMed Central ID: PMC7423983]. https://doi.org/10.1038/s41392-020-00265-w.
-
29.
Oceandy D, Amanda B, Ashari FY, Faizah Z, Azis MA, Stafford N. The Cross-Talk Between the TNF-alpha and RASSF-Hippo Signalling Pathways. Int J Mol Sci. 2019;20(9):2346. [PubMed ID: 31083564]. [PubMed Central ID: PMC6539482]. https://doi.org/10.3390/ijms20092346.
-
30.
DeAngelis MW, McGhie EW, Coolon JD, Johnson RI. Mask, a component of the Hippo pathway, is required for Drosophila eye morphogenesis. Dev Biol. 2020;464(1):53-70. [PubMed ID: 32464117]. [PubMed Central ID: PMC7416169]. https://doi.org/10.1016/j.ydbio.2020.05.002.
-
31.
He M, Zhou Z, Shah AA, Hong Y, Chen Q, Wan Y. New insights into posttranslational modifications of Hippo pathway in carcinogenesis and therapeutics. Cell Div. 2016;11:4. [PubMed ID: 27042197]. [PubMed Central ID: PMC4818516]. https://doi.org/10.1186/s13008-016-0013-6.
-
32.
Hirate Y, Sasaki H. The role of angiomotin phosphorylation in the Hippo pathway during preimplantation mouse development. Tissue Barriers. 2014;2(1):e28127. [PubMed ID: 24843842]. [PubMed Central ID: PMC4022607]. https://doi.org/10.4161/tisb.28127.
-
33.
Hassani SN, Moradi S, Taleahmad S, Braun T, Baharvand H. Transition of inner cell mass to embryonic stem cells: mechanisms, facts, and hypotheses. Cell Mol Life Sci. 2019;76(5):873-92. [PubMed ID: 30420999]. https://doi.org/10.1007/s00018-018-2965-y.
-
34.
Sasaki H. Roles and regulations of Hippo signaling during preimplantation mouse development. Dev Growth Differ. 2017;59(1):12-20. [PubMed ID: 28035666]. https://doi.org/10.1111/dgd.12335.
-
35.
Watanabe Y, Miyasaka KY, Kubo A, Kida YS, Nakagawa O, Hirate Y, et al. Notch and Hippo signaling converge on Strawberry Notch 1 (Sbno1) to synergistically activate Cdx2 during specification of the trophectoderm. Sci Rep. 2017;7:46135. [PubMed ID: 28401892]. [PubMed Central ID: PMC5389439]. https://doi.org/10.1038/srep46135.
-
36.
Gharanfoli S, Shahverdi A, Dalman A, Ghaznavi P, Alipour H, Eftekhari-Yazdi P. Effect of Maternal Age on Hippo Pathway Related Gene Expressions and Protein Localization Pattern in Human Embryos. Cell J. 2020;22(Suppl 1):74-80. [PubMed ID: 32779436]. [PubMed Central ID: PMC7481894]. https://doi.org/10.22074/cellj.2020.6860.
-
37.
Dehghanian F, Hojati Z, Esmaeili F, Masoudi-Nejad A. Network-based expression analyses and experimental validations revealed high co-expression between Yap1 and stem cell markers compared to differentiated cells. Genomics. 2019;111(4):831-9. [PubMed ID: 29775782]. https://doi.org/10.1016/j.ygeno.2018.05.007.
-
38.
Luo J, Li P. Context-dependent transcriptional regulations of YAP/TAZ in stem cell and differentiation. Stem Cell Res Ther. 2022;13(1):10. [PubMed ID: 35012640]. [PubMed Central ID: PMC8751096]. https://doi.org/10.1186/s13287-021-02686-y.
-
39.
Wu Y, Aegerter P, Nipper M, Ramjit L, Liu J, Wang P. Hippo Signaling Pathway in Pancreas Development. Front Cell Dev Biol. 2021;9:663906. [PubMed ID: 34079799]. [PubMed Central ID: PMC8165189]. https://doi.org/10.3389/fcell.2021.663906.
-
40.
Li Y, Xiao C, Li R, Zhong W, Xu G, Zhang W. Role of Yes-associated protein (YAP) in regulation of mesenchymal stem cell tenogenic differentiation. J Mol Histol. 2022;53(2):273-83. [PubMed ID: 35048214]. https://doi.org/10.1007/s10735-022-10059-9.
-
41.
Yan HC, Sun Y, Zhang MY, Zhang SE, Sun JD, Dyce PW, et al. YAP regulates porcine skin-derived stem cells self-renewal partly by repressing Wnt/beta-catenin signaling pathway. Histochem Cell Biol. 2022;157(1):39-50. [PubMed ID: 34586448]. https://doi.org/10.1007/s00418-021-02034-4.
-
42.
Ramos A, Camargo FD. The Hippo signaling pathway and stem cell biology. Trends Cell Biol. 2012;22(7):339-46. [PubMed ID: 22658639]. [PubMed Central ID: PMC3383919]. https://doi.org/10.1016/j.tcb.2012.04.006.
-
43.
Cao X, Wang C, Liu J, Zhao B. Regulation and functions of the Hippo pathway in stemness and differentiation. Acta Biochim Biophys Sin (Shanghai). 2020;52(7):736-48. [PubMed ID: 32445460]. https://doi.org/10.1093/abbs/gmaa048.
-
44.
Ozaki M. Cellular and molecular mechanisms of liver regeneration: Proliferation, growth, death and protection of hepatocytes. Semin Cell Dev Biol. 2020;100:62-73. [PubMed ID: 31669133]. https://doi.org/10.1016/j.semcdb.2019.10.007.
-
45.
Li W, Li L, Hui L. Cell Plasticity in Liver Regeneration. Trends Cell Biol. 2020;30(4):329-38. [PubMed ID: 32200807]. https://doi.org/10.1016/j.tcb.2020.01.007.
-
46.
Wang EY, Cheng JC, Thakur A, Yi Y, Tsai SH, Hoodless PA. YAP transcriptionally regulates ErbB2 to promote liver cell proliferation. Biochim Biophys Acta Gene Regul Mech. 2018;1861(9):854-63. [PubMed ID: 30025876]. https://doi.org/10.1016/j.bbagrm.2018.07.004.
-
47.
Hong L, Cai Y, Jiang M, Zhou D, Chen L. The Hippo signaling pathway in liver regeneration and tumorigenesis. Acta Biochim Biophys Sin (Shanghai). 2015;47(1):46-52. [PubMed ID: 25476204]. https://doi.org/10.1093/abbs/gmu106.
-
48.
Zhang S, Zhou D. Role of the transcriptional coactivators YAP/TAZ in liver cancer. Curr Opin Cell Biol. 2019;61:64-71. [PubMed ID: 31387016]. https://doi.org/10.1016/j.ceb.2019.07.006.
-
49.
Avruch J, Zhou D, Bardeesy N. YAP oncogene overexpression supercharges colon cancer proliferation. Cell Cycle. 2012;11(6):1090-6. [PubMed ID: 22356765]. [PubMed Central ID: PMC3335916]. https://doi.org/10.4161/cc.11.6.19453.
-
50.
He L, Yuan L, Yu W, Sun Y, Jiang D, Wang X, et al. A Regulation Loop between YAP and NR4A1 Balances Cell Proliferation and Apoptosis. Cell Rep. 2020;33(3):108284. [PubMed ID: 33086070]. https://doi.org/10.1016/j.celrep.2020.108284.
-
51.
Miyamoto Y, Sakane F, Hashimoto K. N-cadherin-based adherens junction regulates the maintenance, proliferation, and differentiation of neural progenitor cells during development. Cell Adh Migr. 2015;9(3):183-92. [PubMed ID: 25869655]. [PubMed Central ID: PMC4594476]. https://doi.org/10.1080/19336918.2015.1005466.
-
52.
Deryckere A, Styfhals R, Elagoz AM, Maes GE, Seuntjens E. Identification of neural progenitor cells and their progeny reveals long distance migration in the developing octopus brain. Elife. 2021;10:e69161. [PubMed ID: 34425939]. [PubMed Central ID: PMC8384421]. https://doi.org/10.7554/eLife.69161.
-
53.
Wang Y, Yu A, Yu FX. The Hippo pathway in tissue homeostasis and regeneration. Protein Cell. 2017;8(5):349-59. [PubMed ID: 28130761]. [PubMed Central ID: PMC5413598]. https://doi.org/10.1007/s13238-017-0371-0.
-
54.
Cao X, Pfaff SL, Gage FH. YAP regulates neural progenitor cell number via the TEA domain transcription factor. Genes Dev. 2008;22(23):3320-34. [PubMed ID: 19015275]. [PubMed Central ID: PMC2600760]. https://doi.org/10.1101/gad.1726608.
-
55.
Tremblay AM, Camargo FD. Hippo signaling in mammalian stem cells. Semin Cell Dev Biol. 2012;23(7):818-26. [PubMed ID: 23034192]. https://doi.org/10.1016/j.semcdb.2012.08.001.
-
56.
Fernandez LA, Northcott PA, Dalton J, Fraga C, Ellison D, Angers S, et al. YAP1 is amplified and up-regulated in hedgehog-associated medulloblastomas and mediates Sonic hedgehog-driven neural precursor proliferation. Genes Dev. 2009;23(23):2729-41. [PubMed ID: 19952108]. [PubMed Central ID: PMC2788333]. https://doi.org/10.1101/gad.1824509.
-
57.
Shibata M, Ham K, Hoque MO. A time for YAP1: Tumorigenesis, immunosuppression and targeted therapy. Int J Cancer. 2018;143(9):2133-44. [PubMed ID: 29696628]. [PubMed Central ID: PMC6540999]. https://doi.org/10.1002/ijc.31561.
-
58.
Barron DA, Kagey JD. The role of the Hippo pathway in human disease and tumorigenesis. Clin Transl Med. 2014;3:25. [PubMed ID: 25097728]. [PubMed Central ID: PMC4112623]. https://doi.org/10.1186/2001-1326-3-25.
-
59.
Jin L, Huang H, Ni J, Shen J, Liu Z, Li L, et al. Shh-Yap signaling controls hepatic ductular reactions in CCl4 -induced liver injury. Environ Toxicol. 2021;36(2):194-203. [PubMed ID: 32996673]. https://doi.org/10.1002/tox.23025.
-
60.
Santucci M, Vignudelli T, Ferrari S, Mor M, Scalvini L, Bolognesi ML, et al. The Hippo Pathway and YAP/TAZ-TEAD Protein-Protein Interaction as Targets for Regenerative Medicine and Cancer Treatment. J Med Chem. 2015;58(12):4857-73. [PubMed ID: 25719868]. https://doi.org/10.1021/jm501615v.
-
61.
Ouyang T, Meng W, Li M, Hong T, Zhang N. Recent Advances of the Hippo/YAP Signaling Pathway in Brain Development and Glioma. Cell Mol Neurobiol. 2020;40(4):495-510. [PubMed ID: 31768921]. https://doi.org/10.1007/s10571-019-00762-9.
-
62.
Rognoni E, Walko G. The Roles of YAP/TAZ and the Hippo Pathway in Healthy and Diseased Skin. Cells. 2019;8(5):411. [PubMed ID: 31058846]. [PubMed Central ID: PMC6562585]. https://doi.org/10.3390/cells8050411.
-
63.
Mendoza-Reinoso V, Beverdam A. Epidermal YAP activity drives canonical WNT16/beta-catenin signaling to promote keratinocyte proliferation in vitro and in the murine skin. Stem Cell Res. 2018;29:15-23. [PubMed ID: 29562208]. https://doi.org/10.1016/j.scr.2018.03.005.
-
64.
Andl T, Zhou L, Yang K, Kadekaro AL, Zhang Y. YAP and WWTR1: New targets for skin cancer treatment. Cancer Lett. 2017;396:30-41. [PubMed ID: 28279717]. https://doi.org/10.1016/j.canlet.2017.03.001.
-
65.
Schlegelmilch K, Mohseni M, Kirak O, Pruszak J, Rodriguez JR, Zhou D, et al. Yap1 acts downstream of alpha-catenin to control epidermal proliferation. Cell. 2011;144(5):782-95. [PubMed ID: 21376238]. [PubMed Central ID: PMC3237196]. https://doi.org/10.1016/j.cell.2011.02.031.
-
66.
Silvis MR, Kreger BT, Lien WH, Klezovitch O, Rudakova GM, Camargo FD, et al. alpha-catenin is a tumor suppressor that controls cell accumulation by regulating the localization and activity of the transcriptional coactivator Yap1. Sci Signal. 2011;4(174):ra33. [PubMed ID: 21610251]. [PubMed Central ID: PMC3366274]. https://doi.org/10.1126/scisignal.2001823.
-
67.
Feng X, Degese MS, Iglesias-Bartolome R, Vaque JP, Molinolo AA, Rodrigues M, et al. Hippo-independent activation of YAP by the GNAQ uveal melanoma oncogene through a trio-regulated rho GTPase signaling circuitry. Cancer Cell. 2014;25(6):831-45. [PubMed ID: 24882515]. [PubMed Central ID: PMC4074519]. https://doi.org/10.1016/j.ccr.2014.04.016.
-
68.
Liu C, Wu Y, Ma J. Interaction of non-coding RNAs and Hippo signaling: Implications for tumorigenesis. Cancer Lett. 2020;493:207-16. [PubMed ID: 32822816]. https://doi.org/10.1016/j.canlet.2020.08.012.
-
69.
Dong X, Meng L, Liu P, Ji R, Su X, Xin Y, et al. YAP/TAZ: a promising target for squamous cell carcinoma treatment. Cancer Manag Res. 2019;11:6245-52. [PubMed ID: 31360073]. [PubMed Central ID: PMC6625644]. https://doi.org/10.2147/CMAR.S197921.
-
70.
Ortega A, Vera I, Diaz MP, Navarro C, Rojas M, Torres W, et al. The YAP/TAZ Signaling Pathway in the Tumor Microenvironment and Carcinogenesis: Current Knowledge and Therapeutic Promises. Int J Mol Sci. 2021;23(1):430. [PubMed ID: 35008857]. [PubMed Central ID: PMC8745604]. https://doi.org/10.3390/ijms23010430.
-
71.
Gao J, Han W, He Y, Zhou J, Miao J, Zhang G. Livin promotes tumor progression through YAP activation in ovarian cancer. Am J Cancer Res. 2020;10(10):3179-93. [PubMed ID: 33163264]. [PubMed Central ID: PMC7642671].
-
72.
Bartucci M, Dattilo R, Moriconi C, Pagliuca A, Mottolese M, Federici G, et al. TAZ is required for metastatic activity and chemoresistance of breast cancer stem cells. Oncogene. 2015;34(6):681-90. [PubMed ID: 24531710]. https://doi.org/10.1038/onc.2014.5.
-
73.
Strepkos D, Markouli M, Papavassiliou KA, Papavassiliou AG, Piperi C. Emerging roles for the YAP/TAZ transcriptional regulators in brain tumour pathology and targeting options. Neuropathol Appl Neurobiol. 2022;48(2):e12762. [PubMed ID: 34409639]. https://doi.org/10.1111/nan.12762.
-
74.
MacDonagh L, Gray SG, Breen E, Cuffe S, Finn SP, O'Byrne KJ, et al. Lung cancer stem cells: The root of resistance. Cancer Lett. 2016;372(2):147-56. [PubMed ID: 26797015]. https://doi.org/10.1016/j.canlet.2016.01.012.
-
75.
Pan Q, Li Q, Liu S, Ning N, Zhang X, Xu Y, et al. Concise Review: Targeting Cancer Stem Cells Using Immunologic Approaches. Stem Cells. 2015;33(7):2085-92. [PubMed ID: 25873269]. [PubMed Central ID: PMC4478204]. https://doi.org/10.1002/stem.2039.
-
76.
Moharil RB, Dive A, Khandekar S, Bodhade A. Cancer stem cells: An insight. J Oral Maxillofac Pathol. 2017;21(3):463. [PubMed ID: 29391738]. [PubMed Central ID: PMC5763886]. https://doi.org/10.4103/jomfp.JOMFP_132_16.
-
77.
Phi LTH, Sari IN, Yang YG, Lee SH, Jun N, Kim KS, et al. Cancer Stem Cells (CSCs) in Drug Resistance and their Therapeutic Implications in Cancer Treatment. Stem Cells Int. 2018;2018:5416923. [PubMed ID: 29681949]. [PubMed Central ID: PMC5850899]. https://doi.org/10.1155/2018/5416923.
-
78.
Dehghanian F, Azhir Z, Akbari A, Hojati Z. New Insights into the Roles of Yes-Associated Protein (YAP1) in Colorectal Cancer Development and Progression. Ann Colorectal Res. 2019;7(2):e96458. https://doi.org/10.5812/acr.96458.
-
79.
Lorenzetto E, Brenca M, Boeri M, Verri C, Piccinin E, Gasparini P, et al. YAP1 acts as oncogenic target of 11q22 amplification in multiple cancer subtypes. Oncotarget. 2014;5(9):2608-21. [PubMed ID: 24810989]. [PubMed Central ID: PMC4058031]. https://doi.org/10.18632/oncotarget.1844.
-
80.
Mo JS, Park HW, Guan KL. The Hippo signaling pathway in stem cell biology and cancer. EMBO Rep. 2014;15(6):642-56. [PubMed ID: 24825474]. [PubMed Central ID: PMC4197875]. https://doi.org/10.15252/embr.201438638.
-
81.
Zanconato F, Cordenonsi M, Piccolo S. YAP/TAZ at the Roots of Cancer. Cancer Cell. 2016;29(6):783-803. [PubMed ID: 27300434]. [PubMed Central ID: PMC6186419]. https://doi.org/10.1016/j.ccell.2016.05.005.
-
82.
Jaiswal PK, Mohajan S, Koul S, Wang F, Shi R, Koul HK. Prostate-Derived ETS Factor (PDEF) Modulates Yes Associated Protein 1 (YAP1) in Prostate Cancer Cells: A Potential Cross-Talk between PDEF and Hippo Signaling. Pharmaceuticals (Basel). 2019;12(4):181. [PubMed ID: 31835563]. [PubMed Central ID: PMC6958407]. https://doi.org/10.3390/ph12040181.
-
83.
Zhang L, Yang S, Chen X, Stauffer S, Yu F, Lele SM, et al. The hippo pathway effector YAP regulates motility, invasion, and castration-resistant growth of prostate cancer cells. Mol Cell Biol. 2015;35(8):1350-62. [PubMed ID: 25645929]. [PubMed Central ID: PMC4372700]. https://doi.org/10.1128/MCB.00102-15.
-
84.
Guo Y, Cui J, Ji Z, Cheng C, Zhang K, Zhang C, et al. miR-302/367/LATS2/YAP pathway is essential for prostate tumor-propagating cells and promotes the development of castration resistance. Oncogene. 2017;36(45):6336-47. [PubMed ID: 28745315]. https://doi.org/10.1038/onc.2017.240.
-
85.
Cordenonsi M, Zanconato F, Azzolin L, Forcato M, Rosato A, Frasson C, et al. The Hippo transducer TAZ confers cancer stem cell-related traits on breast cancer cells. Cell. 2011;147(4):759-72. [PubMed ID: 22078877]. https://doi.org/10.1016/j.cell.2011.09.048.
-
86.
Ciamporcero E, Shen H, Ramakrishnan S, Yu Ku S, Chintala S, Shen L, et al. YAP activation protects urothelial cell carcinoma from treatment-induced DNA damage. Oncogene. 2016;35(12):1541-53. [PubMed ID: 26119935]. [PubMed Central ID: PMC4695331]. https://doi.org/10.1038/onc.2015.219.
-
87.
Cheng H, Zhang Z, Rodriguez-Barrueco R, Borczuk A, Liu H, Yu J, et al. Functional genomics screen identifies YAP1 as a key determinant to enhance treatment sensitivity in lung cancer cells. Oncotarget. 2016;7(20):28976-88. [PubMed ID: 26716514]. [PubMed Central ID: PMC5045371]. https://doi.org/10.18632/oncotarget.6721.
-
88.
Zhao Y, Khanal P, Savage P, She YM, Cyr TD, Yang X. YAP-induced resistance of cancer cells to antitubulin drugs is modulated by a Hippo-independent pathway. Cancer Res. 2014;74(16):4493-503. [PubMed ID: 24812269]. https://doi.org/10.1158/0008-5472.CAN-13-2712.
-
89.
Lin L, Sabnis AJ, Chan E, Olivas V, Cade L, Pazarentzos E, et al. The Hippo effector YAP promotes resistance to RAF- and MEK-targeted cancer therapies. Nat Genet. 2015;47(3):250-6. [PubMed ID: 25665005]. [PubMed Central ID: PMC4930244]. https://doi.org/10.1038/ng.3218.
-
90.
Kim MH, Kim J, Hong H, Lee SH, Lee JK, Jung E, et al. Actin remodeling confers BRAF inhibitor resistance to melanoma cells through YAP/TAZ activation. EMBO J. 2016;35(5):462-78. [PubMed ID: 26668268]. [PubMed Central ID: PMC4772854]. https://doi.org/10.15252/embj.201592081.
-
91.
Tsai HC, Huang CY, Su HL, Tang CH. CTGF increases drug resistance to paclitaxel by upregulating survivin expression in human osteosarcoma cells. Biochim Biophys Acta. 2014;1843(5):846-54. [PubMed ID: 24462773]. https://doi.org/10.1016/j.bbamcr.2014.01.007.
-
92.
Liu-Chittenden Y, Huang B, Shim JS, Chen Q, Lee SJ, Anders RA, et al. Genetic and pharmacological disruption of the TEAD-YAP complex suppresses the oncogenic activity of YAP. Genes Dev. 2012;26(12):1300-5. [PubMed ID: 22677547]. [PubMed Central ID: PMC3387657]. https://doi.org/10.1101/gad.192856.112.
-
93.
Gibault F, Bailly F, Corvaisier M, Coevoet M, Huet G, Melnyk P, et al. Molecular Features of the YAP Inhibitor Verteporfin: Synthesis of Hexasubstituted Dipyrrins as Potential Inhibitors of YAP/TAZ, the Downstream Effectors of the Hippo Pathway. ChemMedChem. 2017;12(12):954-61. [PubMed ID: 28334506]. https://doi.org/10.1002/cmdc.201700063.
-
94.
Wang C, Zhu X, Feng W, Yu Y, Jeong K, Guo W, et al. Verteporfin inhibits YAP function through up-regulating 14-3-3sigma sequestering YAP in the cytoplasm. Am J Cancer Res. 2016;6(1):27-37. [PubMed ID: 27073720]. [PubMed Central ID: PMC4759394].
-
95.
Yu FX, Luo J, Mo JS, Liu G, Kim YC, Meng Z, et al. Mutant Gq/11 promote uveal melanoma tumorigenesis by activating YAP. Cancer Cell. 2014;25(6):822-30. [PubMed ID: 24882516]. [PubMed Central ID: PMC4075337]. https://doi.org/10.1016/j.ccr.2014.04.017.
-
96.
Dasari VR, Mazack V, Feng W, Nash J, Carey DJ, Gogoi R. Verteporfin exhibits YAP-independent anti-proliferative and cytotoxic effects in endometrial cancer cells. Oncotarget. 2017;8(17):28628-40. [PubMed ID: 28404908]. [PubMed Central ID: PMC5438678]. https://doi.org/10.18632/oncotarget.15614.
-
97.
Sorrentino G, Ruggeri N, Specchia V, Cordenonsi M, Mano M, Dupont S, et al. Metabolic control of YAP and TAZ by the mevalonate pathway. Nat Cell Biol. 2014;16(4):357-66. [PubMed ID: 24658687]. https://doi.org/10.1038/ncb2936.
-
98.
Oku Y, Nishiya N, Shito T, Yamamoto R, Yamamoto Y, Oyama C, et al. Small molecules inhibiting the nuclear localization of YAP/TAZ for chemotherapeutics and chemosensitizers against breast cancers. FEBS Open Bio. 2015;5:542-9. [PubMed ID: 26199863]. [PubMed Central ID: PMC4506957]. https://doi.org/10.1016/j.fob.2015.06.007.
-
99.
Wang Z, Wu Y, Wang H, Zhang Y, Mei L, Fang X, et al. Interplay of mevalonate and Hippo pathways regulates RHAMM transcription via YAP to modulate breast cancer cell motility. Proc Natl Acad Sci U S A. 2014;111(1):E89-98. [PubMed ID: 24367099]. [PubMed Central ID: PMC3890879]. https://doi.org/10.1073/pnas.1319190110.
-
100.
Moroishi T, Hayashi T, Pan WW, Fujita Y, Holt MV, Qin J, et al. The Hippo Pathway Kinases LATS1/2 Suppress Cancer Immunity. Cell. 2016;167(6):1525-39-e17. [PubMed ID: 27912060]. [PubMed Central ID: PMC5512418]. https://doi.org/10.1016/j.cell.2016.11.005.