Abstract
Background:
Polycyclic aromatic hydrocarbons (PAHs) are persistence organic chemicals with proved carcinogenic and mutagenic hazards. These compounds are usually adsorbed in soils in vicinity of oil and gas industries. Bioremediation of PAHs contaminated soils is difficult due to hydrophobic nature of PAHs.Objectives:
The main purpose of the current study was to determine the pyrene removal efficiency in synthetically contaminated soil, using biosurfactant.Materials and Methods:
Four pure bacterial strains capable of pyrene degradation were isolated from contaminated soils via enrichment techniques. The soil samples were spiked with an initial pyrene concentration of 500 mg/kg and subjected to bioremediation using a mixed culture comprised of previously isolated strains, in addition to application of biosurfactant during 63 days.Results:
The pyrene removal efficiency in samples containing biosurfactant, without biosurfactant and controls, were 86.4%, 59.8% and 14%, respectively, after 63 days. The difference of pyrene removal efficiency between the biosurfactant-containing samples and the ones without it was significant (P < 0.05).Conclusions:
Application of rhamnolipid biosurfactant produced by Pseudomonas aeruginosa significantly improved pyrene removal in contaminated soils.Keywords
1. Background
Polycyclic aromatic hydrocarbons (PAHs) are organic chemicals made of more than two fused aromatic rings in a linear or clustered arrangement. The main source of PAHs production is incomplete combustion of hydrocarbons and fossil fuels. Both natural occurrences and anthropogenic activities contribute in PAHs production. Contamination of PAHs in environment, especially in soils around the oil and petrochemical industries, can be hardly verified by different reports (1). The hydrophobic nature of PAHs is a concerning aspect which increases their persistency and toxicity to the environment. Consequently, PAHs are easily adsorbed into solid matrixes and form tight bindings with soil organic matter. Soil is the main sink of PAHs, although they have been detected in air, water and vegetation (2). The US Environmental Protection Agency has signed PAHs as priority among pollutants because of their mutagenic and carcinogenic effects (3). Different remediation methods including solvent extraction, phytoremediation, chemical remediation, photo catalytic remediation, electrochemical remediation, thermal destruction and bioremediation, are investigated for remediation of soils contaminated with hydrocarbons (4-11). Bioremediation has gained more attention among other methods because of less environmental hazards, as well as economic and practical aspects. The major difficulty of bioremediation is the isolation and characterization of bacterial strains capable of using PAHs as the source of carbon and energy. Bacterial strains can only use dissolved carbon sources, and since PAHs are hydrophobic compounds, they make PAH-bacteria contact a major problem for bioremediation of PAHs contaminated sites (12-14). For a successful bioremediation, solubility of contaminant in the soil slurry should be enhanced. One of the most effective methods to increase the solubility of PAHs is application of biosurfactants to facilitate PAH desorption from soil texture (15, 16).
Biosurfactants are a group of surface-active molecules produced by variety of microorganisms. They are amphiphilic compounds containing hydrophilic and hydrophobic moieties. These compounds are produced on active surfaces or excreted extracellularly and also can accumulate between two fluid phases, which leads to reduction of the surface as well as interfacial tensions at the surface and the interface, respectively (17-20). Although chemical surfactants are presented and produced more quickly, biosurfactants have advantages including lower toxicity, biodegradability, selectivity, specific activity at extreme conditions (temperature, pH and salinity), and applications in environment protection and management, especially as an enhancer of polycyclic aromatic hydrocarbons bioavailability to microorganisms (11, 21, 22). Nikolopoulou et al. studied the application of biosurfactants for bioremediation of petroleum-contaminated soil, in which 80% of the initial oil concentration was removed (11). In addition, Nayak et al. reported the successful application of biosurfactants for bioremediation of hydrocarbon-contaminated soils (23). Saeki et al. studied bioremediation of oil spills through biosurfactant application and verified the removal process enhancement (19).
2. Objectives
In the current study, application of rhamnolipid biosurfactant produced by Pseudomonas aeruginosa was investigated for bioremediation of synthetic pyrene-contaminated soils.
3. Materials and Methods
3.1. Materials
The following chemicals, used in the current study, were all at the analytical grade and were purchased from Merck, Fluka, and Dr. Mojjalali: acetone, methanol, nutrient broth, nutrient agar, agar, pyrene, n-hexane, yeast extract, NaCl, K2HPO4, CuSO4.2H2O, KH2PO4, NH4NO3, Na2MoO4, MgSO4.7H2O, CaCl2.H2O, FeSO4.7H2O, C°Cl2.6H2O, MnSO4.H2O, H3BO3, ZnSO4.7H2O.
3.2. Instruments
The instruments and devises used in the current study are listed in Table 1.
Instrument | Model | Manufacturing Country | Application |
---|---|---|---|
Gas chromatography | Chrompack CP 9001 | USA | Pyrene determination |
GC-MS | Konik O12 | China | Determination of metabolites |
Tensiometer | Kruss K 100 | China | Determination of surface tension |
XRD | Philips PW2404 | The Netherlands | Determination of soil characteristics |
XRF | Philips PW2404 | The Netherlands | Determination of soil characteristics |
Shaker incubator | IKM 4000 ci | Germany | Incubation |
Spectrophotometer | Philips PU8620 | The Netherlands | Monitoring of bacterial growth |
3.3. Methods
3.3.1. Isolation of Pyrene Degrading Bacteria
Isolation and enrichment of pyrene degrading bacteria was based on the method described by Youssef et al. (12). Briefly, 10 g of soil from the oil fields in south of Iran, contaminated with different hydrocarbons, was transferred to a 250-mL flask containing 100 mL of phosphate buffered mineral salt (PMS) solution; it was shaken vigorously for 10 minutes and then was allowed to settle. An aliquot of 5 mL supernatant was then transferred into a 250-mL flask containing 95 mL sterilized PMS. Composition of the liquid PMS used in this study was as follows (g/L): K2HPO4, 6.3; KH2PO4, 1.8; yeast extract 1; MgSO4.7H2O, 0.1; CaCl2.H2O, 0.1; FeSO4.7H2O, 0.1; MnSO4.H2O, 0.1 and 1 mL/L of trace elements solution (23), pH = 7. The trace elements solution contained (g/L) H3BO3, 0.03; ZnSO4.7H2O, 0.01; CaCl2.6H2O, 0.02; Na2MoO4, 0.006; CuSO4.2H2O, 0.001, pH = 7. The NaOH and HCl solutions were used to adjust pH at the desired value. Pyrene was used as the sole source of carbon and energy for enrichment of pyrene degrading consortium and was added to the medium at 200 mg/L. Pyrene powder with water solubility of 0.135 mg/L was dissolved in n-hexane to form an emulsion in water, which could be accessible by bacteria. All the enrichment mediums were sterilized by autoclaving. The flasks were incubated at 30°C on an orbital shaker at 150 rpm (IKM 4000 ci, Germany) for seven days. Growth was monitored by measuring the absorbance at 600 nm. After seven days of incubation, an aliquot of 5 mL enriched culture was transferred into another 250-mL flask containing 95 mL of fresh pyrene PMS medium. This procedure was repeated eight times to obtain a pyrene degrading enriched consortium. To isolate pure bacterial strains, 1 mL of the supernatant culture was diluted to 10-4 times, spread onto pyrene-coated mineral agar plates and incubated at 37°C for 48-72 hours. Thereafter, the colonies demonstrated sufficient growth on pyrene + agar mineral medium were determined as strains capable of degrading pyrene and maintained on nutrient agar slant at 4°C. Four pure strains of S1, S2, S3 and S4 were selected for bioremediation test.
3.3.2. Preparation of Biosurfactant
The rhamnolipid biosurfactant used in the current study was produced by Pseudomonas aeruginosa. The isolation method of the biosurfactant-producing bacteria as well as extraction and characterization of biosurfactant have been presented in details by Jorfi et al. (24). Briefly, the mentioned biosurfactant was a glycolipid with critical micelle concentration (CMC) of 60 mg/L, emulsifying index (E24) of 66%, ability to decrease the surface tension of liquid medium to 29.5 mN/m, and acceptable stability against a wide range of pH values and temperatures.
3.3.3. Soil Preparation
The soil was derived from an oil industrial zone in south of Iran. The Soil samples were collected from the upper layer (0-20 cm) by soil cores, air-dried, and passed through a 2-mm sieve. Afterwards, the sieved soil samples were washed three times with acetone, autoclaved, and stored in plastic containers at 4°C. The resulting samples were spiked with a solution of 500 mg/kg pyrene, dissolved in n-hexane through PTFE filters, shacked, and left under the hood for 48 hours, till the n-hexane evaporated.
3.3.4. The Bioremediation Procedure
All the experiments were carried out in a batch mode operation in 250 mL flasks. Briefly, 20 g of pre-prepared soil, 100 mL of PMS, 5 mL of inoculums with OD 600 nm = 1 (consortium comprised of four pure strains: S1 to S4), and the biosurfactant with constant concentration of 4 CMC were added to each flask. The experimental flasks were placed on a shaker incubator at 150 rpm and 31°C. Before incubation, pH of the samples was adjusted to 7 ± 0.2 by HCl and NaOH solutions. The controls included the samples without biosurfactant and the samples free of bacterial seed. To evaluate the differences of the results, statistical analysis of T-test with P value of 0.05 was used.
3.9. Analytical Methods
The bacterial density was evaluated according to the most probable number (MPN) (25). Determination and extraction of pyrene was performed according to USEPA method (26) by gas chromatography system (Chrompack CP 9001), equipped with a flame ionization detector (FID), using a 30-m HP5 capillary column, with 0.32 mm inside diameter and 0.2 µm coated film thickness. α-naphtol was used as an internal standard. Nitrogen was used as the carrier gas by the rate of 2 mL/minute. The temperature program was as follows: the column temperature was held at 120°C for 1 minute and then ramped to 240°C at a rate of 20°C/minute. This temperature was held at 240°C for 1 minute. The injector and detector temperatures were set 250 and 300°C, respectively (26, 27). The samples were analyzed weekly during 63 days. The soil type was determined by particle size analysis and the composition and elements were assessed with XRD (X-ray diffraction) and XRF (X-ray fluorescence). The XRD instrument characteristics included: Philips model PW2404, made of Netherland, tube Cu k α, ʎ 1.54056 angstrom, step size: 0.02 o/s, voltage: 40 kv, current: 30 mA. The intermediate metabolites were determined by GC-MS.
4. Results
4.1. Soil Composition
The soil classification was silty sand with 10.3% clay, 73.6% silt, 16.1% sand, and porosity of 24.7%. Findings of XRF analysis quantification of soil constituents and XRD for kind of constituents (Figure 1,Table 2) are presented below.
. Physical and Chemical Characteristics of the Studied soil According to X-Ray Diffraction and X-Ray Fluorescence Analysis
Parameter | Amount, % |
---|---|
Sand | 16.1 |
Clay | 10.3 |
Silt | 76.1 |
Porosity | 24.7 |
Moisture | 6.78 |
LOI | 10.7 |
Na2O | 1.944 |
MgO | 2.046 |
Al2O3 | 16.095 |
SiO2 | 54.798 |
P2O5 | 0.202 |
K2O | 3.163 |
CaO | 8.842 |
TiO2 | 0.569 |
Fe2O3 | 1.4155 |
Cu | 0.158 |
Sr | 0.047 |
Zr | 0.019 |
SiO2 | 54.798 |
X-Ray Diffraction Analysis of the Studied Soil Sample
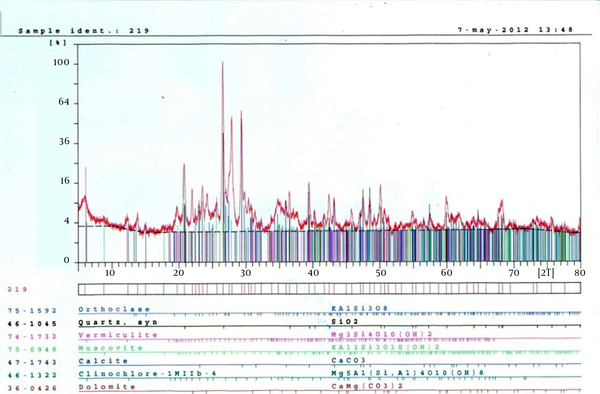
4.2. Pyrene Removal Efficiency
The residual pyrene concentration in the soil sample with initial pyrene concentration of 500 mg/kg and application of rhamnolipid biosurfactant gradually decreased to 77 mg/kg (removal efficiency 84.6%) during 63 days (Figure 2). The bacterial density of inoculums (OD 600 nm = 1) was 500 × 107. According to the curve of Figure 2 for pyrene concentration variation, similar to many batch mode systems, the bacterial density was first increased after a short lag phase and decreased thereafter gradually. The most bacterial density of 900 × 1012 and the least of 60 × 105 were observed at days 21 and 63, respectively.
The residual pyrene concentration in the soil sample with initial pyrene concentration of 500 mg/kg and without any biosurfactant gradually decreased to 201 mg/kg (removal efficiency of 59.8%) during 63 days (Figure 3). According to the observed data, the most bacterial density of 500 × 1011 and the least of 7 × 106 were observed at days 14 and 63, respectively.
The control samples without application of any bacterial seed were considered as chemical controls. Pyrene concentration was also decreased along with time because of processes other than biological reactions. It is believed that sequestration and adsorption to soil particles is the main reason of pyrene removal in control samples. Pyrene concentration decreased to 430 mg/kg (removal efficiency of 14%) at the end of day 63 (Figure 4).
Pyrene Removal Efficiency Against Bacterial Density Variations in the Soil Sample With Initial Pyrene Concentration of 500 mg/kg and Biosurfactant Application
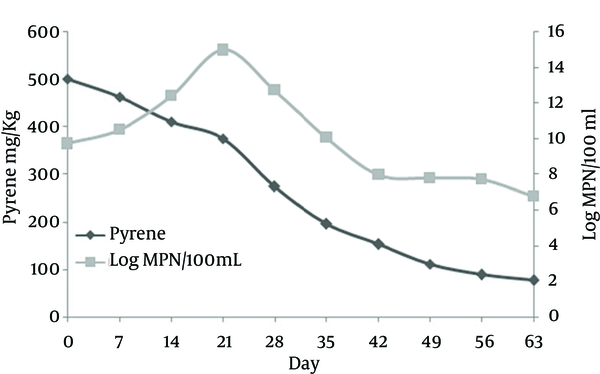
Pyrene Removal Efficiency Against Bacterial Density Variations in the Soil Sample With Initial Pyrene Concentration of 500 mg/kg and Without biosurfactant Application
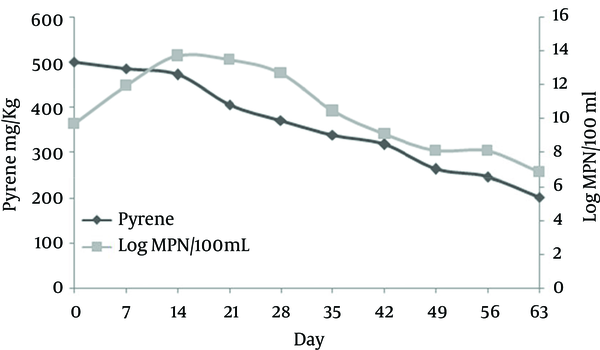
Pyrene Removal Efficiency in Chemical Controls
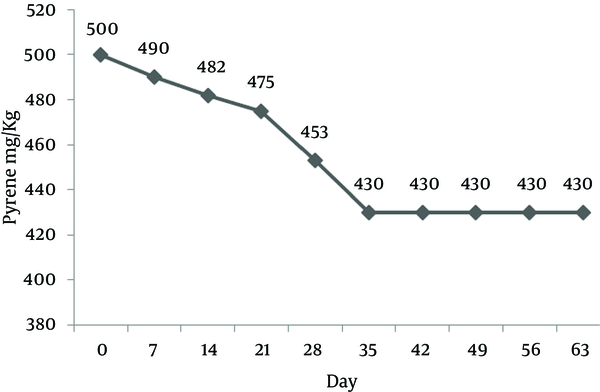
4.3. Comparison of Pyrene Removal in Different Conditions of Excrement
The pyrene removal efficiency for initial concentration of 500 mg/kg, with the same reaction time of 63 days and varying conditions in term of biosurfactant and inoculums application, is compared in Figure 5. The samples with biosurfactant application exhibited the best results with 84.6% removal efficiency, followed by samples without biosurfactant and chemical controls without bacterial seed, corresponded to 59.8% and 14 removal efficiencies, respectively.
Pyrene Removal Efficiency for Different Experimental Conditions
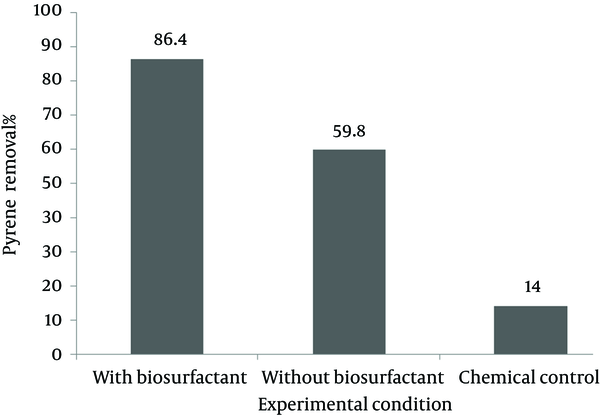
4.4. Intermediate Metabolites
The most important metabolites of bioremediation with biosurfactant application are presented in Figure 6. These metabolites were 1) tricosane, 2) hexadecane, and 3) 2-methyl butyl ester.
Intermediate Metabolites of Bioremediation of Pyrene Artificially Contaminated Soil
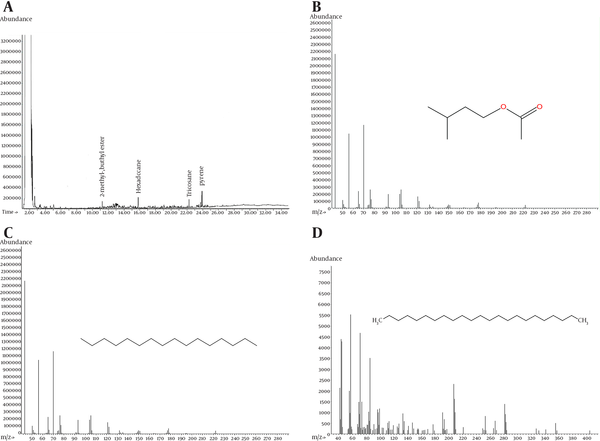
5. Discussion
A bacterial consortium comprised of four pure strains was used for pyrene degradation. The effect of produced rhamnolipid biosurfactant in pyrene removal from synthetically contaminated soil via enhancing the solubility and bioavailability of the contaminant was studied. The contaminant model, pyrene, is a four ring PAH, which is not as carcinogenic as benzo (a) pyrene, but is severely mutagenic and a usual soil contaminant near oil and gas industries (28). In addition, pyrene has low solubility in water and well simulates the condition of poor bioavailability of hydrocarbons to bacteria in harsh environments. Pyrene removal of 84.6% for initial concentration of 500 mg/kg and biosurfactant application in the soil remediation process after 63 days was more than soil samples without biosurfactant application with removal efficiency of 59.8%. The pyrene removal efficiency in samples with biosurfactant application improved only 5% from day 56 to 63, which was not considerable. Therefore, the reaction time can be decreased to 56 days for initial pyrene concentrations of 500 mg/kg. A shorter time is especially important for integrated processes, where bioremediation is supported by other remediation techniques such as chemical oxidation in the case of highly contaminated soils. The pyrene removal difference in samples with biosurfactant application and samples without it was significant according to T-test analysis (P < 0.05). The superiority of biosurfactant-containing samples can be referred to their ability to dissolve hydrophobic pyrene and improve the bioavailability of hydrocarbon to bacteria. Reddy et al. used biosurfactant-producing bacteria for degradation of phenanthrene with an initial concentration of 250 mg/kg. The removal efficiency was negligible in the two first days and then increased rapidly to 93% till day six. Their study was based on the produced biosurfactant by phenanthrene degrading bacteria and without any external source of biosurfactant (20). Pei et al. studied the effects of rhamnolipid and Tween 80 in the same concentrations of 50 mg/L for bioremediation of solutions containing 40 mg/L phenanthrene. The phenanthrene removal efficiency for samples containing Tween 80 was 33.5% after 10 days, compared to 99.5% for biosurfactant-containing samples in the same reaction time (29). In a study by Jia et al. the produced biosurfactants were used by two pure strains of Zooglea and Aspergillus niger in the same concentrations of 12 g/kg for degradation of an initial pyrene concentration of 100 mg/kg. They reported a pyrene removal efficiency of 80% after 35 days (28). The obtained removal efficiency by Jia et al. was more than the findings of the current study, perhaps due to higher concentration of biosurfactant application, soil characteristics, application a mixture of two biosurfactant, and the type of bacterial consortium. Lu et al. also studied pyrene biodegradation in contaminated soil without any biosurfactant addition. The removal efficiency for initial pyrene concentrations of 13, 28, 61, 121, 171 and 236 mg/kg ranged between 47-55% during 50 days (30), which were less than the amounts obtained in the current study for samples containing biosurfactant, but were similar to those without any biosurfactant in the same reaction times. A possible reason for pyrene removal in samples without any biosurfactant addition, other than inherent ability of bacterial strains to metabolize some of the pyrene content and a little solution of pyrene in the reaction medium, can be referred to production of exopolymeric substances (EPS) from the present bacteria and emission of EPS to the solution after they died. This phenomenon increases the bioavailability of hydrophobic contaminant; however, the characteristics of EPS including CMC, emulsifying index (E24) and surface tension lowering property of the solution are very poor compared to biosurfactants like surfactin or rhamnolipid. This conclusion has been verified by some other studies (28). Since EPS contains hydrophobic and hydrophilic moieties, they pose various surface characteristics and enhance the solubility of hydrocarbons. In the first step, hydrophobic forces move pyrene and EPS close together (31). Interactions of biosurfactant and PAHs are spontaneous and exothermic and are affected by hydrophobic interactions. On the other hand, intracellular enzymes of EPS contain oxidoreductase and hydrolase, which play important roles in pyrene degradation. Some toxic compounds can be degraded by oxidoreductases like laccase, polyphenol oxidase and catalase, which are related through PAHs degradation (32). These factors can be the possible reasons of pyrene removal in samples without any biosurfactant application. The control samples without bacterial consortium showed 14% pyrene removal in the same reaction time for initial pyrene concentration of 500 mg/kg. Removal can be referred to as the adsorption of contaminant to soil particles. The studied soil sample with 10.3% clay and 10.7% organic content can be considered as an adsorbent which removed some portion of pyrene. Adsorption of heavy four-to-six-ring PAHs to soil has been verified in other studies. As the PAH hydrophobic characteristic increases (Kow ≥ 5), adsorption to soil particles is more expected. Unfortunately, there is not any soil standard in term of PAHs and the comparison of findings of the current study with desired condition is not possible. Therefore, a higher efficiency would be more acceptable. Some of the European and North American countries like England, Canada and Holland use the phenanthrene guideline of 40 mg/kg as the PAH standard for soil (33). If this guideline is considered as the base of comparison, removal of pyrene to less than 40 mg/kg was not observed in any of the studied conditions of soil remediation till day 63, but a large portion of pyrene (84.6%) was removed, which cannot be argued due to lack of internal standards. However, it was an acceptable output because the majority of pyrene was removed.
We can conclude that application of produced rhamnolipid biosurfactant from Pseudomonas aeruginosa sp. can enhance bioremediation of soils artificially contaminated with pyrene significantly in lab scale studies. Evaluation of the process efficiency in pilot and full scale studies in the presence of real effective factors and cumulative concentrations of hydrocarbons in naturally contaminated soil can be considered to introduce a valid, viable, reliable and efficient remediation method.
Acknowledgements
References
-
1.
USEPA. Polycyclic Aromatic Hydrocarbons (PAHs). 2008. Available from: http://www.epa.gov/osw/hazard/wastemin/priority.htm.
-
2.
Gan S, Lau EV, Ng HK. Remediation of soils contaminated with polycyclic aromatic hydrocarbons (PAHs). J Hazard Mater. 2009;172(2-3):532-49. [PubMed ID: 19700241]. https://doi.org/10.1016/j.jhazmat.2009.07.118.
-
3.
Guo CL, Zhou HW, Wong YS, Tam NF. Isolation of PAH-degrading bacteria from mangrove sediments and their biodegradation potential. Mar Pollut Bull. 2005;51(8-12):1054-61. [PubMed ID: 16291206]. https://doi.org/10.1016/j.marpolbul.2005.02.012.
-
4.
Lai CC, Huang YC, Wei YH, Chang JS. Biosurfactant-enhanced removal of total petroleum hydrocarbons from contaminated soil. J Hazard Mater. 2009;167(1-3):609-14. [PubMed ID: 19217712]. https://doi.org/10.1016/j.jhazmat.2009.01.017.
-
5.
Ferrarese E, Andreottola G, Oprea IA. Remediation of PAH-contaminated sediments by chemical oxidation. J Hazard Mater. 2008;152(1):128-39. [PubMed ID: 17689010]. https://doi.org/10.1016/j.jhazmat.2007.06.080.
-
6.
Zhang L, Li P, Gong Z, Li X. Photocatalytic degradation of polycyclic aromatic hydrocarbons on soil surfaces using TiO(2) under UV light. J Hazard Mater. 2008;158(2-3):478-84. [PubMed ID: 18372106]. https://doi.org/10.1016/j.jhazmat.2008.01.119.
-
7.
Priya DN, Modak JM, Trebse P, Zabar R, Raichur AM. Photocatalytic degradation of dimethoate using LbL fabricated TiO2/polymer hybrid films. J Hazard Mater. 2011;195:214-22. [PubMed ID: 21880417]. https://doi.org/10.1016/j.jhazmat.2011.08.030.
-
8.
Yu XZ, Wu SC, Wu FY, Wong MH. Enhanced dissipation of PAHs from soil using mycorrhizal ryegrass and PAH-degrading bacteria. J Hazard Mater. 2011;186(2-3):1206-17. [PubMed ID: 21176862]. https://doi.org/10.1016/j.jhazmat.2010.11.116.
-
9.
Gonzalez N, Simarro R, Molina MC, Bautista LF, Delgado L, Villa JA. Effect of surfactants on PAH biodegradation by a bacterial consortium and on the dynamics of the bacterial community during the process. Bioresour Technol. 2011;102(20):9438-46. [PubMed ID: 21862321]. https://doi.org/10.1016/j.biortech.2011.07.066.
-
10.
Ahn CK, Kim YM, Woo SH, Park JM. Soil washing using various nonionic surfactants and their recovery by selective adsorption with activated carbon. J Hazard Mater. 2008;154(1-3):153-60. [PubMed ID: 18006231]. https://doi.org/10.1016/j.jhazmat.2007.10.006.
-
11.
Nikolopoulou M, Kalogerakis N. Enhanced bioremediation of crude oil utilizing lipophilic fertilizers combined with biosurfactants and molasses. Mar Pollut Bull. 2008;56(11):1855-61. [PubMed ID: 18799169]. https://doi.org/10.1016/j.marpolbul.2008.07.021.
-
12.
Youssef NH, Duncan KE, Nagle DP, Savage KN, Knapp RM, McInerney MJ. Comparison of methods to detect biosurfactant production by diverse microorganisms. J Microbiol Methods. 2004;56(3):339-47. [PubMed ID: 14967225]. https://doi.org/10.1016/j.mimet.2003.11.001.
-
13.
Zhao HP, Wu QS, Wang L, Zhao XT, Gao HW. Degradation of phenanthrene by bacterial strain isolated from soil in oil refinery fields in Shanghai China. J Hazard Mater. 2009;164(2-3):863-9. [PubMed ID: 18930349]. https://doi.org/10.1016/j.jhazmat.2008.08.098.
-
14.
Paria S. Surfactant-enhanced remediation of organic contaminated soil and water. Adv Colloid Interface Sci. 2008;138(1):24-58. [PubMed ID: 18154747]. https://doi.org/10.1016/j.cis.2007.11.001.
-
15.
Zhao Z, Selvam A, Wong JW. Synergistic effect of thermophilic temperature and biosurfactant produced by Acinetobacter calcoaceticus BU03 on the biodegradation of phenanthrene in bioslurry system. J Hazard Mater. 2011;190(1-3):345-50. [PubMed ID: 21530078]. https://doi.org/10.1016/j.jhazmat.2011.03.042.
-
16.
Christofi N, Ivshina IB. Microbial surfactants and their use in field studies of soil remediation. J Appl Microbiol. 2002;93(6):915-29. [PubMed ID: 12452947].
-
17.
Lotfabad TB, Shourian M, Roostaazad R, Najafabadi AR, Adelzadeh MR, Noghabi KA. An efficient biosurfactant-producing bacterium Pseudomonas aeruginosa MR01, isolated from oil excavation areas in south of Iran. Colloids Surf B Biointerfaces. 2009;69(2):183-93. [PubMed ID: 19131218]. https://doi.org/10.1016/j.colsurfb.2008.11.018.
-
18.
Hasanshahian M, Emtiazi G. Investigation of alkane biodegradation using the microtiter plate method and correlation between biofilm formation, biosurfactant production and crude oil biodegradation. Inter j Bio Biodeg. 2008;62(2):170-8. https://doi.org/10.1016/j.ibiod.2008.01.004.
-
19.
Saeki H, Sasaki M, Komatsu K, Miura A, Matsuda H. Oil spill remediation by using the remediation agent JE1058BS that contains a biosurfactant produced by Gordonia sp. strain JE-1058. Bioresour Technol. 2009;100(2):572-7. [PubMed ID: 18692393]. https://doi.org/10.1016/j.biortech.2008.06.046.
-
20.
Reddy SM, Naresh B, Leela T, Prashanthi M, Madhusudhan N, Dhanasri GD. Biodegradation of phenanthrene with biosurfactant production by a new strain of Brevibacillus. 21. 21 ed ed. Washington, DC: American Public Health Association; 1996. p. 7980-3.
-
21.
Płaza GA, Zjawiony I, Banat IM. Use of different methods for detection of thermophilic biosurfactant-producing bacteria from hydrocarbon-contaminated and bioremediated soils. J Petroleum Sci Engin. 2006;50(1):71-7. https://doi.org/10.1016/j.petrol.2005.10.005.
-
22.
Yap CL, Gan S, Ng HK. Fenton based remediation of polycyclic aromatic hydrocarbons-contaminated soils. Chemosphere. 2011;83(11):1414-30. [PubMed ID: 21316731]. https://doi.org/10.1016/j.chemosphere.2011.01.026.
-
23.
Nayak AS, Vijaykumar MH, Karegoudar TB. Characterization of biosurfactant produced by Pseudoxanthomonas sp. PNK-04 and its application in bioremediation. Inter Bio Biodeg. 2009;63(1):73-9. https://doi.org/10.1016/j.ibiod.2008.07.003.
-
24.
Jorfi S, Rezaee A, Mobeh-Ali G, Jaafarzadeh N. Application of Biosurfactants Produced byPseudomonas aeruginosa SP4for Bioremediation of Soils Contaminated by Pyrene. Soil Sedim Contamin. 2013;22(8):890-911. https://doi.org/10.1080/15320383.2013.770439.
-
25.
American Public Health Association. Standard methods for the examination of water and wastewater. 21 ed. Washington: APHA; 2005.
-
26.
Rezaei Kalantary R, Jorfi S, Dehghani fard E. Modification of PAHs biodegradation with humic compounds. Soil Sed Contamin. 2012;21.
-
27.
USEPA. Ultrasonic extraction. envir res info j. 1996.
-
28.
Jia C, Li P, Li X, Tai P, Liu W, Gong Z. Degradation of pyrene in soils by extracellular polymeric substances (EPS) extracted from liquid cultures. Proc Biochem j. 2011;46(8):1627-31. https://doi.org/10.1016/j.procbio.2011.05.005.
-
29.
Pei X, Zhan X, Wang S, Lin Y, Zhou L. Effects of a Biosurfactant and a Synthetic Surfactant on Phenanthrene Degradation by a Sphingomonas Strain. Pedosphere. 2010;20(6):771-9. https://doi.org/10.1016/s1002-0160(10)60067-7.
-
30.
Lu M, Zhang Z, Qiao W, Wei X, Guan Y, Ma Q, et al. Remediation of petroleum-contaminated soil after composting by sequential treatment with Fenton-like oxidation and biodegradation. Bioresour Technol. 2010;101(7):2106-13. [PubMed ID: 19942431]. https://doi.org/10.1016/j.biortech.2009.11.002.
-
31.
Hilliou L, Freitas F, Oliveira R, Reis MAM, Lespineux D, Grandfils C, et al. Solution properties of an exopolysaccharide from a Pseudomonas strain obtained using glycerol as sole carbon source. Carbohyd Poly j. 2009;78(3):526-32. https://doi.org/10.1016/j.carbpol.2009.05.011.
-
32.
Gianfreda L, Rao MA. Potential of extra cellular enzymes in remediation of polluted soils: a review. Enz Microbial Tech j. 2004;35(4):339-54. https://doi.org/10.1016/j.enzmictec.2004.05.006.
-
33.
Sun HW, Yan QS. Influence of pyrene combination state in soils on its treatment efficiency by Fenton oxidation. J Environ Manage. 2008;88(3):556-63. [PubMed ID: 17517464]. https://doi.org/10.1016/j.jenvman.2007.03.031.