Abstract
Context:
For the prevention and management of thromboembolic complications, warfarin is the most extensively recommended anticoagulant. It is categorized as a drug with a narrow therapeutic window. Therefore, warfarin prescription requires special attention related to therapeutic drug monitoring.Evidence Acquisition:
By categorizing the clinical implications of warfarin, this manuscript aims to provide a comprehensive (albeit somewhat brief) conclusion associated with its pharmacotherapy. The key words relevant to the topic were searched. Consequently, articles relevant to the pharmacotherapeutic management of warfarin were selected and reviewed in their entirety.Results:
To obtain a reasonable level of stability between the required antithrombotic treatment and the risk of bleeding, an analysis of the literature revealed that the prothrombin time in terms of the international normalized ratio (INR) was found for each individual. The best model for stable warfarin dosage prediction was found to be based on multiple linear regression. Genotype-guided procedures were established to: 1, improve the time in the therapeutic range; 2, reduce time to the first therapeutic INR; and 3, reduce the time for the stable doses. Vitamin K epoxide reductase is an enzyme with an important role in vitamin K metabolism, and warfarin is metabolized in hepatocytes via a monooxygenase, cytochrome P450 2C9. In patients carrying 2C9*1/*2 and 2C9*2/*2 or 2C9*1/*3 alleles, the dose is recommended to be reduced by 18% - 40% and 21% - 49%, respectively.Conclusions:
Race, age, body surface area, chronic kidney disease, CYP2C9*3 level, and VKORC1 variants could affect the dose of warfarin. To administer the proper doses of warfarin, patients and physicians might achieve the best results with the pharmacologist proficient anticoagulation database and recommended continuation program. Owing to its’ unpredictability, caution must be taken when prescribing warfarin. More advanced warfarin pharmacotherapy studies are recommended based on a linear regression model specifically in the Iranian population.Keywords
1. Context
Warfarin, otherwise known as Coumadin, Jantoven, Marevan, or Uniwarfin, has a systemic international union of pure and applied chemistry name of (RS)-4-Hydroxy-3-(3-oxo-1-phenylbutyl)-2H-chromen-2-one. It is an anticoagulant usually administered via oral or intravenous routes for the prevention of: 1, thrombosis; 2, thromboembolism; and 3, the development of blood masses in the blood vessels. In 1948, the drug was presented as an insecticide against rats and mice, and then in 1950, its safety and efficacy as an anticoagulant was established and consequently it was used for such treatment in 1954 (1-3). More specifically, it is used for the prevention and treatment of thromboembolic events in patients with deep vein thrombosis, pulmonary embolism, atrial fibrillation, and prosthetic heart valves. However, regardless of its efficiency, treatment with warfarin comes with numerous problems (4). Due to the narrow therapeutic window, deciding upon a suitable dosage of warfarin is a challenging task (5). For instance, a body mass index of more than 30 has been connected to augmented time for achieving a therapeutic international normalized ratio (INR) on the starting dose of warfarin, as well as higher maintenance doses (6).
Table 1 shows the clinical data on warfarin. After oral administration, the drug has a bioavailability of 79% - 100%. Warfarin has a protein binding rate of 99%. With a mean of 40 hours, the minimum and maximum half-life of the drug has been reported as 20 to 60 hours, respectively. 92% of the drug is excreted through the kidneys. The main organ for metabolism of the drug is the liver through the activation of CYP3C9, 2C9, 2C8, 1A2, and 3A4. Indandione derivatives (fluindion) or coumarin derivatives (acenocoumarol, phenprocoumon, or warfarin) are involved in vitamin K antagonist metabolism. Concerning the latter, the shorter half-life acenocoumarol or longer half-life phenprocoumon are coumarins that are not completely interchangeable with warfarin.
Clinical Data of Warfarin
Clinical Issues | Information |
---|---|
IUPAC name | (RS)-4-Hydroxy-3-(3-oxo-1-phenylbutyl)- 2H-chromen-2-one |
Trade names | Coumadin, Jantoven, Marevan |
Legal status | By prescription only |
Pregnancy category | D (AU), X (US) |
Route of administration | Oral or intravenous |
Bioavailability | 70% - 100% |
Protein binding | 99% |
Half-Life | 20 - 60 hours (mean 40 hours) |
Metabolism | Hepatic (CYP2C9, 2C19, 2C8, 2C18, 1A2, and 3A4) |
Administration | By prescription only, route of: oral or intravenous, available as: 0.5 and 1 mg |
Effectiveness | 1, Prevention and treatment of thromboembolic events in patients with deep vein thrombosis, pulmonary embolism, atrial fibrillation and prosthetic heart valves; (4) and 2, more effective in patients with high blood pressure, or left ventricular dysfunction particularly individuals aged > 75 years (7). |
Dose | Differs by race, age, body surface area, chronic kidney disease, amiodarone, CYP2C9*3, and VKORC1 variants. In those carrying 2C9*1/*2 and 2C9*2/*2 or 2C9*1/*3 alleles, the dose is recommended to be reduced by 18% - 40% and 21% - 49%, respectively (8). |
Drug-Drug interaction | 1, Oseltamivir (9); 2, Simvastatin; 3, Metronidazole; 4, other macrolides; 5, broad-spectrum antibiotics; 6, food that contains large quantities of vitamin K1; 7, banana flakes (10); 8, hypothyroidism; 9, excessive use of alcohol; 10, many herbs and spices such as ginseng and Ginkgo biloba, borage (starflower) oil, fish oils; and 11,cranberry juice. |
Reported adverse effects | 1, The higher risk of bleeding in patients obese as BMI > 30 could increase time to achieve a therapeutic INR (starting dose and maintenance dose) (6); 2, spontaneous spinal subdural hematoma at a rare level (11); 3, bleeding, particularly intracranial hemorrhage with multiple cerebral microbleeds (12); 4, bleeding events and life-threatening hemorrhage (13); 5, Warfarin embryopathy connected with maternal warfarin ingestion, warfarin resistance, extreme anticoagulation and warfarin reversal (14). |
Figure 1 shows the mechanism of action for warfarin. In the coagulation process, the inactive factors II, VII, IX, and X should be converted to active forms by the cofactors K1H2 for γ-glutamyl carboxylase (GGCX). In the blood stream, vitamin K1 is carried by apolipoprotein E, and then taken up by receptors. It is then reduced to vitamin K1H2 by vitamin K reductase. Vitamin K epoxide reductase complex 1 (VKORC1) and possibly epoxide hydrolase 1 (EPHX1) catalyze the reduction of vitamin K1O. Warfarin, after being metabolized by cytochrome P450 isoforms to R-warfarin and S-warfarin, inhibits VKORC1, decreases the amount of vitamin K1H2 and thus interferes with the activation of clotting factors (1-6, 14, 15). The aim of this review is to briefly provide updated information related to the pharmacotherapy of warfarin in different clinical practices.
Mechanism of Action of Warfarin
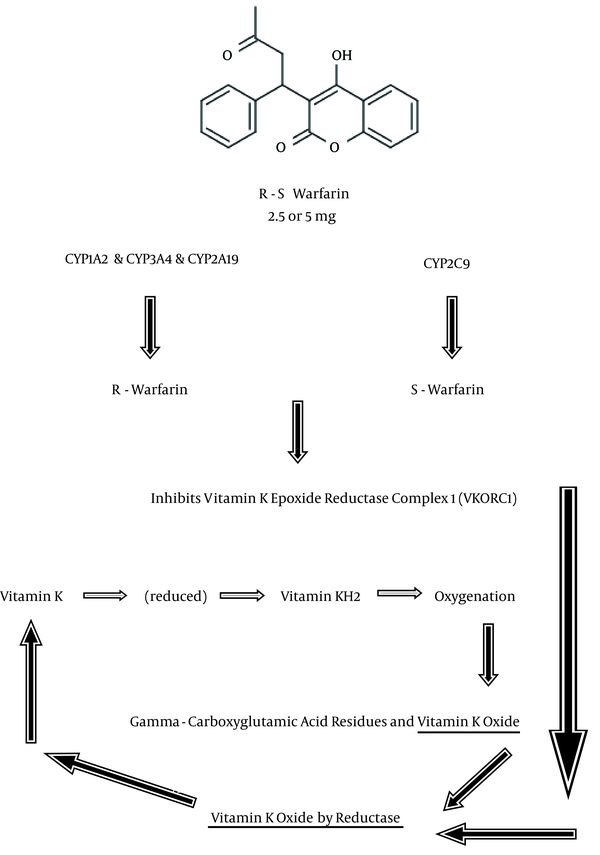
Ball-and-Stick Model of Warfarin
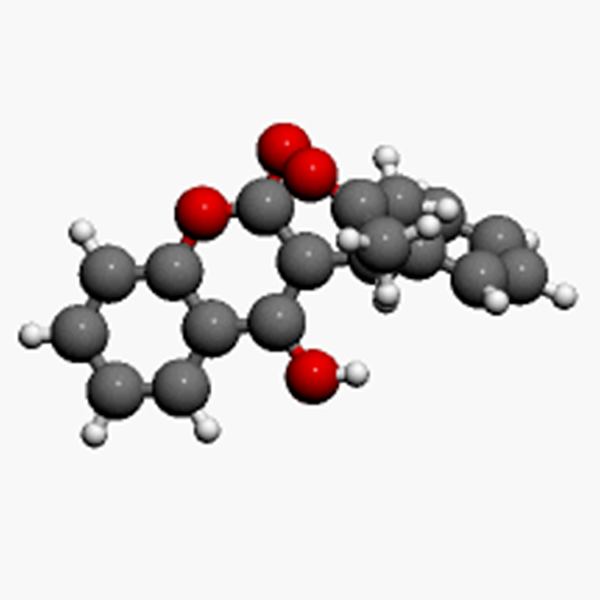
2. Evidence Acquisition
Inter- and intra- individual variation of pharmacotherapy using warfarin needs to be well-designed and then documented individually for each prescription. As a result, clinical trials and review articles on obtaining efficiency of warfarin use in clinical practice were collected and evaluated. In this article, we provide a summary of the warfarin mechanisms of action that contribute to the beneficial effects of the drug and the conditions facilitating ideal effectiveness of prescription-based therapies in clinical practice.
2.1. Survey Method
The United States national library of medicine (PubMed, NLM) database was searched. The key words relevant to warfarin were investigated, including the following: 1, warfarin; 2, warfarin efficacy and safety; 3, warfarin efficacy and safety in clinical practice; 4, warfarin dosing; 5, warfarin in neurology; and 6, warfarin dosing in neurology. The total numbers of articles published were as follows: (1) 22,768 (24 November 1950 to 25 June 2015); (2) 1,005 (June 1975 to 29 May 2015); (3) 155 (April 1994 to 17 May 2015); (4) 976 (February 1973 to June 2015); (5) 744 (July 1968 to 26 June 2015); and (6) 15 (July 2005 to May 2015), respectively. Subsequently, items applicable to the pharmacotherapeutic management of warfarin were nominated and evaluated in their entirety.
3. Results
As the most widely prescribed anticoagulant for the prevention and treatment of thromboembolic events, warfarin blocks the synthesis of the specific vitamin-K dependent coagulation factors II, VII, IX, and X, as well as two vitamin K-dependent plasma proteins C and S (16). Even though the drug is greatly effective for these purposes, its use is restricted by a narrow therapeutic window with a more than ten-fold alteration in the dose necessary for satisfactory anticoagulation in different adults. Furthermore, in spite of the absence of an ideal dosing approach in overweight patients, warfarin remains the most commonly used anticoagulant (17). An optimum dose that secures appropriate stability among both the required antithrombotic outcome and the risk of bleeding as measured by the INR prothrombin time must be planned based on inter- and intra- individual variability. A priori dose prediction and a posteriori dose revision seem to be needed in order to obtain the most effective outcomes (17-19).
Warfarin is more effective in patients with high blood pressure or left ventricular dysfunction, particularly individuals aged > 75 years (7). A study of dosing algorithms comparing inpatients with outpatients showed similarities irrespective of whether dosing was pharmacogenetically or clinically guided (20). Studies confirm the need for more systematic patient instruction at the time of target-specific administration of oral anticoagulants (TSOACs) and further maintenance therapy (19). In a study performed in 2015, the target INR for patients with atrial fibrillation was reported between 2.0 and 3.0, but the designated goal INR for mechanical heart valves was reported to be more unstable. Commencement and continued dosing schedules were a main cause of unpredictability with doubt resulting from specific patient issues such as age, ethnicity, and body mass index (18).
Warfarin dose differs by race, age, body surface area, chronic kidney disease, amiodarone, CYP2C9*3, and VKORC1 variants. In patients with acute stroke, genotype-guided dosing is recommended to be more useful by decreasing the time essential for stabilization when compared with fixed dosing. A priori and posteriori dosing could be estimated based on data about body weight, age, baseline, and target INR, with the option of considering the CYP2C9 and VKORC1 genotypes (a priori) and other information about previous doses and the INR (a posteriori), respectively (21, 22).
4. Conclusions
The warfarin dosing algorithms were similar for individuals who started warfarin as inpatients and outpatients, irrespective of whether dosing was pharmacogenetically or clinically conducted (20). The risk of hemorrhage is higher in overweight as compared to normal weight patients who are on warfarin. Therefore, BMI plays an important role in predicting the occurrence of hemorrhage events in patients on warfarin (6). Unsuitable recommendations, monitoring, and management of target-specific oral anticoagulants occurs often in patients not officially registered in an anticoagulation monitoring program. There is a need for more thorough patient education at the time of target-specific oral anticoagulant initiation, as well as improved prescriber education regarding recommended target-specific oral anticoagulant dosing and monitoring (19).
By promoting vitamin K1 recycling and reducing active vitamin K1, warfarin inhibits vitamin K epoxide reductase. This enzyme reuses oxidized vitamin K1 in its reduced form after it has contributed to the carboxylation of numerous blood clotting proteins, mostly prothrombin and factor VII. The action of warfarin may constantly be inverted by vitamin K1, as the blood cannot be coagulated directly. After prescription, warfarin necessitates two to three days for onset of effective treatment in which the duration of the effect of a single dose is about two to five days (1-7, 14-22)
Spontaneous spinal subdural hematoma is reported at a rare level in those receiving anticoagulant or antiplatelet agents (11). However, a great frequency of intracerebral hemorrhages with multiple cerebral microbleeds has been reported in warfarin-treated patients (12). A drug-drug interaction has also been reported between oseltamivir for influenza B and warfarin in an 8-year-old boy with hypoplastic left heart syndrome with a previous history of thrombosis (9). Warfarin embryopathy connected with maternal warfarin ingestion, warfarin resistance, extreme anticoagulation, and warfarin reversal are also reported side-effects that could be accompanied with warfarin therapy (14). Multiple linear regression analysis revealed itself to be the best model for stable warfarin dosage prediction in the Chinese population, as the average predicted achievement ratio and mean of absolute error among the algorithms ranged from 52.31% to 58.08% and 4.25 to 4.84 mg/week in the validation samples, respectively (23). Another study of 2677 patients showed that compared with a fixed dose or clinically adjusted warfarin dosing schedules, genotype-guided algorithms profoundly increased the time in the therapeutic range, reduced the time to first therapeutic INR and the time to stable doses, but did not demonstrate any obvious developments in excessive anticoagulation, bleeding events, thromboembolism, or all-cause mortality (24).
In those carrying 2C9*1/*2 and 2C9*2/*2 or 2C9*1/*3 alleles, the dose was recommended to be reduced by 18% - 40% and 21% - 49%, respectively (25). Bariatric operation can also cause immanent distress for those on warfarin medication (8). In the first month of genotype-guided warfarin therapy, associated with standard dosing, there were no improvements in the percentage of time in the therapeutic range INR > 4.0, major or minor bleeding, thromboembolism, or all-cause mortality. There was shorter time to the maintenance dose, and after one month, there was an improved percentage time in the therapeutic range and reduction of major bleeding incidences, making this a cost-effective strategy in patients requiring longer anticoagulation (13, 26).
Regarding the interactions of warfarin with other food and drugs, caution is required to achieve the appropriate warfarin treatment for each individual. One study revealed that co-administration of warfarin with lenalidomide, an oral immunomodulatory drug that is used for hematological malignancies, indicated no required dose adjustment of either drug (27). However, warfarin users who are prescribed high-risk antibiotics are at an increased risk for serious bleeding events. Early INR evaluation may mitigate this risk (28). Blood levels of S-warfarin have been reported to be augmented by simultaneous administration of metronidazole, an antiprotozoal imidazole derivative (29). Nosebleeding with an INR of 8.0 and intraparenchymal hemorrhage of the left occipital lobe was reported in a 78-year-old Caucasian woman who had started treatment with metronidazole (250 mg every 8 hours for 5 days) and levofloxacin (500 mg QD for 6 days) for an upper respiratory tract infection (30). Interactions between warfarin and nutritional supplements were also represented by an interaction between warfarin and banana flakes that was reported in a 30-year-old male. In such cases, decreased warfarin efficacy and the advent of diarrhea may be connected with a reduced rate and subtherapeutic INR (10).
In conclusion, experienced pharmacotherapy in relation to drugs with narrow therapeutic windows and in terms of recommendations based on detailed benefit-risk assessment requires thorough understanding and the establishment of well-balanced pharmacokinetic parameters in the Iranian population (31-36). Finally, the cytochrome P450 2C9 (CYP2C9) and vitamin K epoxide reductase complex 1 (VKORC1) genotypes have been confirmed to be associated with warfarin dose requirements. Accurate genotyping results are of particular importance for the obtaining of reliable genotype-guided warfarin dosing information (37).
Finally, in spite of the standard of care related to warfarin therapy all over of the world and specifically in Iran, there is still a requirement for the improvement of pharmacotherapeutic knowledge in relation to investigations on the adverse effects through the use of different equations that represent the pharmacokinetics and pharmacodynamics of warfarin. This category of a mechanism-based conclusion, if implemented, could be valuable for initiating and upholding successful warfarin treatment in the hospitals. It will confirm more reliable dose alteration between doctors, and offer well-organized and accurately personalized warfarin medication in both children and adults.
Acknowledgements
References
-
1.
Holford NH. Clinical pharmacokinetics and pharmacodynamics of warfarin. Understanding the dose-effect relationship. Clin Pharmacokinet. 1986;11(6):483-504. [PubMed ID: 3542339]. https://doi.org/10.2165/00003088-198611060-00005.
-
2.
Holbrook AM, Pereira JA, Labiris R, McDonald H, Douketis JD, Crowther M, et al. Systematic overview of warfarin and its drug and food interactions. Arch Intern Med. 2005;165(10):1095-106. [PubMed ID: 15911722]. https://doi.org/10.1001/archinte.165.10.1095.
-
3.
Ansell J, Hirsh J, Hylek E, Jacobson A, Crowther M, Palareti G, et al. Pharmacology and management of the vitamin K antagonists: American College of Chest Physicians Evidence-Based Clinical Practice Guidelines (8th Edition). Chest. 2008;133(6 Suppl):160S-98S. [PubMed ID: 18574265]. https://doi.org/10.1378/chest.08-0670.
-
4.
Lam MP, Cheung BM. The pharmacogenetics of the response to warfarin in Chinese. Br J Clin Pharmacol. 2012;73(3):340-7. [PubMed ID: 22023024]. https://doi.org/10.1111/j.1365-2125.2011.04097.x.
-
5.
Sharabiani A, Bress A, Douzali E, Darabi H. Revisiting Warfarin Dosing Using Machine Learning Techniques. Comput Math Methods Med. 2015;2015:560108. [PubMed ID: 26146514]. https://doi.org/10.1155/2015/560108.
-
6.
Ogunsua AA, Touray S, Lui JK, Ip T, Escobar JV, Gore J. Body mass index predicts major bleeding risks in patients on warfarin. J Thromb Thrombolysis. 2015;40(4):494-8. [PubMed ID: 26076985]. https://doi.org/10.1007/s11239-015-1226-2.
-
7.
Leys D, Balucani C, Cordonnier C. Antiplatelet drugs for ischemic stroke prevention. Cerebrovasc Dis. 2009;27 Suppl 1:120-5. [PubMed ID: 19342841]. https://doi.org/10.1159/000200449.
-
8.
Schullo-Feulner AM, Stoecker Z, Brown GA, Schneider J, Jones TA, Burnett B. Warfarin dosing after bariatric surgery: a retrospective study of 10 patients previously stable on chronic warfarin therapy. Clin Obes. 2014;4(2):108-15. [PubMed ID: 25826733]. https://doi.org/10.1111/cob.12046.
-
9.
Wagner J, Abdel-Rahman SM. Oseltamivir-warfarin interaction in hypoplastic left heart syndrome: case report and review. Pediatrics. 2015;135(5):e1333-6. [PubMed ID: 25917992]. https://doi.org/10.1542/peds.2014-2578.
-
10.
King AE, Strnad K. Probable Interaction Between Warfarin and Banana Flakes Supplement. Nutr Clin Pract. 2016;31(1):125-31. [PubMed ID: 26137942]. https://doi.org/10.1177/0884533615591056.
-
11.
Jung HS, Jeon I, Kim SW. Spontaneous Spinal Subdural Hematoma with Simultaneous Cranial Subarachnoid Hemorrhage. J Korean Neurosurg Soc. 2015;57(5):371-5. [PubMed ID: 26113966]. https://doi.org/10.3340/jkns.2015.57.5.371.
-
12.
Takizawa S, Tanaka F, Nishiyama K, Hasegawa Y, Nagata E, Mizuma A, et al. Protocol for cerebral microbleeds during the non–vitamin k antagonist oral anticoagulants or warfarin therapy in stroke patients with nonvalvular atrial fibrillation (cmb-now) study: Multisite pilot trial. J Stroke Cerebrovasc Dis. 2015;24(9):2143-8.
-
13.
Barton CA, Johnson NB, Case J, Warden B, Hughes D, Zimmerman J, et al. Risk of thromboembolic events after protocolized warfarin reversal with 3-factor PCC and factor VIIa. Am J Emerg Med. 2015;33(11):1562-6. [PubMed ID: 26143317]. https://doi.org/10.1016/j.ajem.2015.06.010.
-
14.
Yuan SM. Warfarin use and dose adjustment in a patient with mitral valve replacement. Pak J Pharm Sci. 2015;28(4):1351-5. [PubMed ID: 26142525].
-
15.
Rubboli A, Becattini C, Verheugt FW. Incidence, clinical impact and risk of bleeding during oral anticoagulation therapy. World J Cardiol. 2011;3(11):351-8. [PubMed ID: 22125670]. https://doi.org/10.4330/wjc.v3.i11.351.
-
16.
Hamberg AK, Hellman J, Dahlberg J, Jonsson EN, Wadelius M. A Bayesian decision support tool for efficient dose individualization of warfarin in adults and children. BMC Med Inform Decis Mak. 2015;15:7. [PubMed ID: 25889768]. https://doi.org/10.1186/s12911-014-0128-0.
-
17.
Quick JA, Meyer JM, Coughenour JP, Barnes SL. Less Is More: Low-dose Prothrombin Complex Concentrate Effective in Acute Care Surgery Patients. Am Surg. 2015;81(6):646-50. [PubMed ID: 26031281].
-
18.
Stewart A, Ganguli A, FitzGerald R, Pirmohamed M. Variation in warfarin prescribing and dosing in the UK: a national survey of anticoagulation clinics. J Clin Pharm Ther. 2015;40(4):466-71. [PubMed ID: 26032753]. https://doi.org/10.1111/jcpt.12291.
-
19.
Simon J, Hawes E, Deyo Z, Bryant Shilliday B. Evaluation of prescribing and patient use of target-specific oral anticoagulants in the outpatient setting. J Clin Pharm Ther. 2015. [PubMed ID: 26032876]. https://doi.org/10.1111/jcpt.12296.
-
20.
Kasner SE, Wang L, French B, Messe SR, Horenstein R, Mohler E3, et al. The Impact of Inpatient Versus Outpatient Initiation on Early Warfarin Dosing. Am J Cardiovasc Drugs. 2015;15(4):267-74. [PubMed ID: 26037731]. https://doi.org/10.1007/s40256-015-0126-3.
-
21.
Limdi NA, Brown TM, Yan Q, Thigpen JL, Shendre A, Liu N, et al. Race influences warfarin dose changes associated with genetic factors. Blood. 2015;126(4):539-45. [PubMed ID: 26024874]. https://doi.org/10.1182/blood-2015-02-627042.
-
22.
Supe S, Poljakovic Z, Bozina T, Ljevak J, Šarinić VM, Bozina N. Clinical application of genotype-guided dosing of warfarin in patients with acute stroke. Arch med res. 2015;46(4):265-73.
-
23.
Li X, Liu R, Luo ZY, Yan H, Huang WH, Yin JY, et al. Comparison of the predictive abilities of pharmacogenetics-based warfarin dosing algorithms using seven mathematical models in Chinese patients. Pharmacogenomics. 2015;16(6):583-90. [PubMed ID: 25872772]. https://doi.org/10.2217/pgs.15.26.
-
24.
Tang HL, Shi WL, Li XG, Zhang T, Zhai SD, Xie HG. Limited clinical utility of genotype-guided warfarin initiation dosing algorithms versus standard therapy: a meta-analysis and trial sequential analysis of 11 randomized controlled trials. Pharmacogenomics J. 2015;15(6):496-504. [PubMed ID: 25869011]. https://doi.org/10.1038/tpj.2015.16.
-
25.
Militaru FC, Vesa SC, Pop TR, Buzoianu AD. Pharmacogenetics aspects of oral anticoagulants therapy. J Med Life. 2015;8(2):171-5. [PubMed ID: 25866574].
-
26.
Dahal K, Sharma S, Fung E, Lee J, Moore JH, Unterborn JN, et al. Meta-analysis of randomized controlled trials of genotype-guided vs standard dosing of warfarin. Chest J. 2015;148(3):701-10.
-
27.
Weiss D, Knight R, Zhou S, Palmisano M, Chen N. Evaluation of pharmacokinetic and pharmacodynamic interactions when lenalidomide is co-administered with warfarin in a randomized clinical trial setting. Clin Drug Investig. 2015;35(7):455-61. [PubMed ID: 26024727]. https://doi.org/10.1007/s40261-015-0299-1.
-
28.
Lane MA, Zeringue A, McDonald JR. Serious bleeding events due to warfarin and antibiotic co-prescription in a cohort of veterans. Am J Med. 2014;127(7):657-663 e2. [PubMed ID: 24657899]. https://doi.org/10.1016/j.amjmed.2014.01.044.
-
29.
Kudo T, Endo Y, Taguchi R, Yatsu M, Ito K. Metronidazole reduces the expression of cytochrome P450 enzymes in HepaRG cells and cryopreserved human hepatocytes. Xenobiotica. 2015;45(5):413-9. [PubMed ID: 25470432]. https://doi.org/10.3109/00498254.2014.990948.
-
30.
Howard-Thompson A, Hurdle AC, Arnold LB, Finch CK, Sands C, Self TH. Intracerebral hemorrhage secondary to a warfarin-metronidazole interaction. Am J Geriatr Pharmacother. 2008;6(1):33-6. [PubMed ID: 18396247]. https://doi.org/10.1016/j.amjopharm.2008.03.003.
-
31.
Tolou Ghamari Z, Mehavari Habibabadi J, Palizban AA. Evidence-based pharmacotherapy of epilepsy. Arch Neurosci. 2014;2(2). https://doi.org/10.5812/archneurosci.18468.
-
32.
Tolou-Ghamari Z. Antiepileptic drugs (AEDs) polypharmacy could lead to buried pharmacokinetic interactions due to CYP450. Drug Metab Lett. 2012;6(3):207-12. [PubMed ID: 23140557].
-
33.
Tolou-Ghamari Z, Zare M, Habibabadi JM, Najafi MR. A quick review of carbamazepine pharmacokinetics in epilepsy from 1953 to 2012. J Res Med Sci. 2013;18(Suppl 1):S81-5. [PubMed ID: 23961295].
-
34.
Tolou-Ghamari Z, Zare M, Habibabadi JM, Najafi MR. Antiepileptic drugs: a consideration of clinical and biochemical outcome in patients with epilepsy. Int J Prev Med. 2013;4(Suppl 2):S330-7. [PubMed ID: 23776747].
-
35.
Tolou-Ghamari Z, Najafi MR, Habibabadi JM, Zare M. Preliminarily Analysis of Carbamazepine (CBZ) C0 in Patients Visited Isfahan Epileptic Clinics. Int J Prev Med. 2013;4(Suppl 2):S343-6. [PubMed ID: 23776749].
-
36.
Kashipazha D, Nejad SEM, Sadr F, Tarahomi S, Sadr S. Comparison of Levetiracetam With Sodium Valproate in Controlling Seizure in Patients Suffering From Juvenile Myoclonic Epilepsy. Jentashapir Journal of Health Research. 2014;5(4).
-
37.
Lin G, Yi L, Zhang K, Sun Y, Wang L, Zhang R, et al. Improvements in CYP2C9 Genotyping Accuracy Are Needed: A Report of the First Proficiency Testing for Warfarin-related CYP2C9 and VKORC1 Genotyping in China. J Cardiovasc Pharmacol. 2015;66(2):129-34. [PubMed ID: 25815675]. https://doi.org/10.1097/FJC.0000000000000254.