Abstract
Background:
Many studies have assessed the effects of either low intensity pulsed ultrasound (LIPUS) or demineralized bone matrix (DBM) on bone repair; however, an evaluation of the combination of these modalities (LIPUS + DBM) has not yet been considered.Objectives:
This study aimed to investigate combined effects of DBM and LIPUS on fracture healing.Methods:
Bilateral 5-mm tibial defects were created in male Dutch rabbits (n = 30). Animals were divided to two groups of empty defect (A) and DBM group (B), in which commercial DBM putty was used in defects. In each animal left tibia was treated with LIPUS (intensity = 30 mW/cm2, I SATA, 1 MHz, 20 min/day, pulsed duty 1:4) and the contralateral limb was used as the control. Animals, after 14, 28 and 60 days, were submitted to radiographic or computerized tomography (CT) scanning analysis.Results:
At two weeks, LIPUS had no substantial effect on bone formation. Slight increase of average rates in LIPUS group (A2) were seen compared to the empty defect group (A1) at day 21 and 28. In the DBM–treated group compared with the sham LIPUS, bone-healing rate was reduced at the end of the period (60 days) after surgery. The average healing rate in group B at the end of the 60-day period was less than group A after 21 days.Conclusions:
The present study discusses systemic effect of LIPUS because of non-significant results between treated group and control group and is the first to demonstrate that LIPUS decreases bone formation induced by DBM.Keywords
1. Background
Millions of fractures occur every year worldwide. Fractures in bone result from two general causes, trauma or pathological conditions. In either case, the continuity of the bone has been disrupted so that the transmission of mechanical loads through the bone becomes impossible. Even though treatment methods have improved over the last few decades, 5 - 10% of fractures still show delayed healing. A significant subpopulation of these delayed healings did not heal by nine months and were thus termed non-unions (1). During the repair of bone, apart from the costs, postoperative care and complications of the internal and external fixation are problematic. On the other hand, patients with non-union fractures mostly need another operation. Retreatment is usually in the form of plates and other alternative methods, and this surgical approach may lead to more pain in patients (2). In this regard, there is urgent need to develop ways to speed up and strengthen the process of fracture healing.
Demineralized bone matrix (DBM) is an allograft derived from processed human bone and functions as an osteoinductive and osteoconductive biomaterial, delivering osteogenic growth factors in a bioresorbable form (3). The ability of DBM to provide an osteoconductive scaffold is important in maintaining the space within the defect to facilitate bone formation, whereas its bone morphogenetic protein (BMP) component, particularly BMP-2 and BMP-7, is thought to be responsible for its osteoinductive potential (4). Bone morphogenetic proteins, are members of a large family of transforming growth factors (TGF), and they allow mesenchymal cells differentiation to osteoblasts. Bone matrix, containing small amounts of morphogenetic protein that demineralizing process reveals these proteins and provides mesenchymal cells more access to them for inducing bone formation (5). Among the reasons that make this method ideal and proper bone graft substitute are tissue compatibility, the ability to change the structure, economic material, accessibility in large amounts, sterilization ability and acceptable storing time (4).
Another promising new therapeutic method is low-intensity pulsed ultrasound. Widespread clinical use of low intensity pulsed ultrasound (LIPUS), in the treatment of acute fractures and delayed union and nonunion, dates back to the 1990s (6). Low Intensity Pulsed Ultrasound is a form of mechanical energy that is transmitted through and into living tissue as acoustic pressure waves and absorbs according to the density of the tissues. In the literature, LIPUS generally entails a 20-minute treatment per day of 1-MHz waves repeating at 1 kHz, average intensity of 30 mW/cm2, and a pulse width of 200 ms. The micromechanical strains made by these pressure waves on body tissue can result in biochemical events at the cellular level and facilitate tissue healing (7). Osteoblasts are sensitive to mechanical force so LIPUS has direct mechanical stimulation on osteoblast proliferation, ossification of cartilage and increasing bone mineralization (8, 9). Other mechanisms for LIPUS are alteration of cellular membrane’s function and subsequently increase in cellular calcium levels, the stimulation of fibroblast activity, increase of protein synthesis, vascular permeability and angiogenesis (10). Intensity used in LIPUS, (30 mW/cm2) is in the range of therapeutic purposes (1 - 50 mW/cm2) so is considered as a non-thermal and non-invasive method (8). Using LIPUS for the treatment of bone fractures has been approved by the food and drug administration (FDA) (11).
2. Objectives
This study was conducted to address the gap in knowledge regarding benefits of LIPUS and the effect of LIPUS on other treatment methods of fracture. The aim of this study was the evaluation of low intensity pulsed ultrasound effects on the osteogenesis potential of demineralized bone matrix by X-ray and CT scan.
3. Methods
3.1. Animals
This study was experimental in design, for which 30 white, male and adult Dutch rabbits (2 - 2.5 kg) were obtained from the department of animal experiment, Razi institute, Tehran. The animals were housed in an experimental animal room (22°C, and 12-h light/dark cycle) and fed a standard laboratory diet and water.
3.2. Study Design
Rabbits were randomly divided to two groups: without graft (group A) and with demineralized bone matrix (group B). To compare effects of LIPUS treatment under standard conditions, in all groups, the right side acted as “sham” and the left side was treated by LIPUS. On the other hand, each rabbit was its own control.
3.3. Surgery Procedure
In brief, general anesthesia was delivered by intramuscular injections of ketamine (100 mg/ kg) and xylazine (10 mg/kg). After shaving the skin and disinfecting the surgical site with 10% povidone iodine solution in each animal, the medial compartment of the tibia was exposed through a longitudinal incision on the shaved skin and muscle tissue. The periosteum was elevated to expose the bone. In the upper third of the tibia (1 cm below of tibial tuberosity) in medial part, a 5-mm circular and unicortical defect was created by low speed drill (200 rpm) with a trephine. Procedures were done under profuse irrigation with sterile saline. Defects in group A were left non-grafted (empty) and in group B, defects were filled with DBM putty (Ceno Bone, tissue regeneration corporation, bone putty) (n = 18). Then muscles and skin was sutured and the skin was disinfected with povidone-iodine. Postoperative care included intramuscular injections of cefazolin and gentamicin for seven days.
3.4. Treatment with Low Intensity Pulsed Ultrasound
The study design encompassed right tibiae as control group (A1, B1) and left tibiae as a treatment group (A2, B2) where low intensity pulsed ultrasound (LIPUS) was performed daily. low intensity pulsed ultrasound was started on the first postoperative day; Low-intensity pulsed ultrasound (Enraf nonius, Sonopuls 434, Holand) (1MHz, 1:4 duty cycle, intensity SATA 30 mW/cm2, 20 min/day, stationary mode application) was used. No sedative agent was given during the ultrasound treatment to avoid the negative effects of sedative agents, and for elimination of air between transducer and therapeutic area, ultrasonic gel was used on the skin. Groups of the study were classified as described below. Progress of healing was followed by weekly radiographic imagine (0, 7, 14, 21, 28, 40, 50 and 60 days) and additional analysis by CT was performed on 14, 28 and 60 days (n = 6). It is important to mention that group A analysis was done only in 14 and 28 days because most of them were treated in this period (n = 12).
Group description:
A1: Defects without graft and without LIPUS therapy
A2: Defects without graft under LIPUS therapy
B1: Defects with DBM putty without LIPUS therapy
B2: Defects with DBM putty and under LIPUS therapy.
Radiographic and CT images were evaluated blindly by radiologist and scored as seen in Table 1.
Description of Radiographic and Computerized-Tomography Image’s Scoring in Rabbit Tibia Defect
Radiologic Score’s Description | Score | CT score’s Description |
---|---|---|
Ability to view defect with defined margin | 0 | View the full defect |
Beginning of filling defect with serrated borders | 1 | Beginning of osteogenesis in defect margin |
Approximately one quarter of defect filled by new bone tissue | 2 | Reduction of defect’s area |
Space filling in defect is half | 3 | Defect filled by new bone and only a small space is left in the middle |
Nearly 75% of defect space is filled with new bone | 4 | Full defect filling, bone thickness is less than the adjacent bone |
Defect completely filled with new bone, but without similar density to adjacent bone tissue | 5 | Complete filling defect with the same or more thickness than adjacent bone |
Complete filling of the defect with similar density to adjacent bone | 6 |
Surgery Procedure
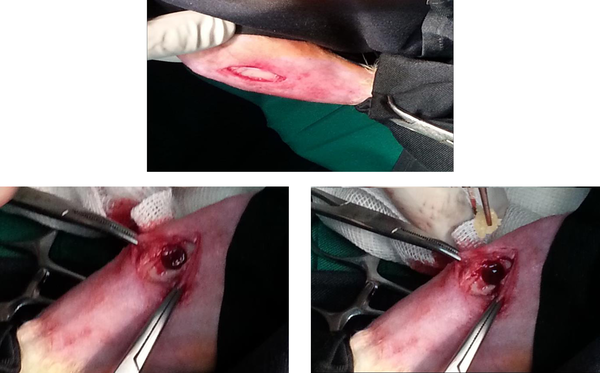
3.5. Statistical Analysis
The radiographic and CT scores were analyzed with non-parametric Mann-Whitney test and Kruskal-Wallis using the SPSS 23 software for windows (SPSS, 23) (ἀ < 0.05).
4. Results
During the present study, no adverse reactions or deaths were observed and the results were on the basis of X-ray and CT observations (Tables 2 and 3).
Mean ± Standard Deviation of Radiology Scoring of the Rabbits
Group | 0 day | 7 day | 14 day | 21 day | 28 day | 40 day | 50 day | 60 day |
---|---|---|---|---|---|---|---|---|
US control (A1) | 0.00 ± 0.00 | 0.00 ± 0.00 | 1.20 ± 0.42 | 5.00 ± 0.70 | 5.20 ± 0.44 | |||
US test (A2) | 0.00 ± 0.00 | 0.00 ± 0.00 | 1.20 ± 0.42 | 5.20 ± 0.83 | 5.40 ± 0.54 | |||
DBM control (B1) | 0.00 ± 0.00 | 0.22 ± 0.42 | 1.55 ± 0.61 | 2.33 ± 0.99 | 3.08 ± 0.99 | 3.833 ± 0.40 | 4.16 ± 0.75 | 4.66 ± 1.03 |
DBM + US (B2) | 0.00 ± 0.00 | 0.16 ± 0.51 | 1.66 ± 0.59 | 2.41 ± 0.88 | 2.66 ± 0.77 | 3.00 ± 1.09 | 3.50 ± 1.37 | 4.16 ± 1.72 |
Mean ± Standard Deviation of Computerized Tomography Scoring in Rabbits
Group | 0 day | 14 day | 28 day | 60 day |
---|---|---|---|---|
US control (A1) | 0.00 ± 0.00 | 0.00 ± 0.00 | 3.2 ± 1.09 | |
US test (A2) | 0.00 ± 0.00 | 0.00 ± 0.00 | 5.2 ± 1.09 | |
DBM control (B1) | 0.00 ± 0.00 | 0.83 ± 0.40 | 4 ± 1.26 | 4.83 ± 1.60 |
DBM + US (B2) | 0.00 ± 0.00 | 0.66 ± 0.51 | 4.60 ± 1.03 | 4.80 ± 0.98 |
4.1. Radiographic anAlysis
Between A1 and A2 groups, there was no significant difference in terms of healing on different days (P > 0.05). However, sharp increase was seen in bone healing and filling of defect between days 14 and 21 in both groups (Figures 2 - 4). More average rates in group A2 were seen compared to group A1 at day 21 (5.2 vs. 5) and 28 (5.4 vs. 5.2), which indicates more regenerative response in the treatment group. However, this difference was not significant in statistical analysis (P > 0.05) (Table 2, Figure 2). In the B1 and B2 groups, an increase in average bone healing was seen at the different times, which shows the progress of bone healing (Figure 2). However, at all time points, mean bone healing rate, between B1 and B2 groups showed no significant difference (P > 0.05). Bone healing rate in group B, showed a slight decline in B2 at day 7 (B1: 0.22 - B2: 0.16). The bone healing rate was increased between B1 and B2 group on days 14 yet reduced from day 21 to the end of the study period (60 days) after surgery (Table 2) (Figures 2, 5 and 6). According to Figure 2, the average healing rate in group B at the end of the 60-day period was less than group A after 21 days.
Mean ± Standard Deviation of Radiology Scoring in Different Groups.
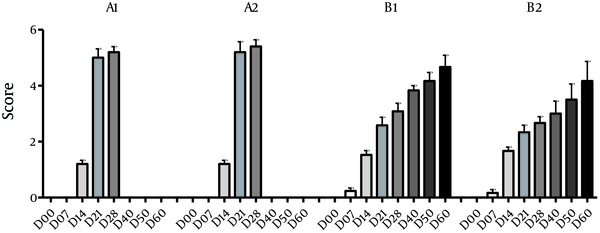
X-ray Observation in the Non-Grafted Group
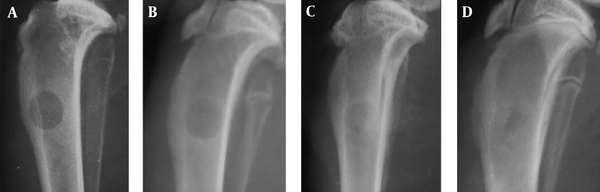
X-ray Observation in Non-Grafted Group Under Treatment of Low Intensity Pulsed Ultrasound
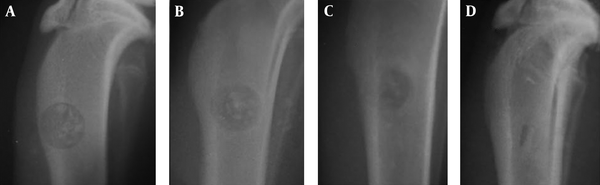
X-ray Observation in the Demineralized Bone Matrix Group
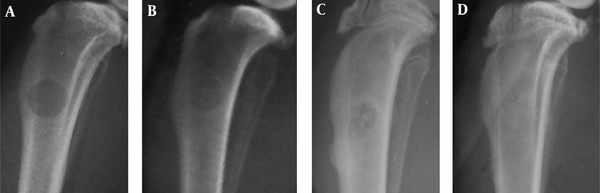
X-ray Observation in Demineralized Bone Matrix + Low Intensity Pulsed Ultrasound Group
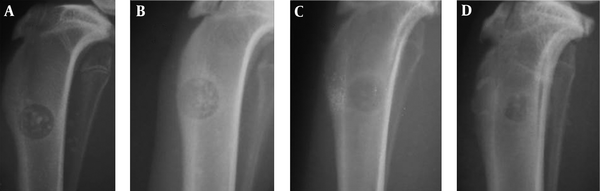
4.2. Computerized Tomography Analysis
Computerized Tomography images showed no significant difference between groups A and B on the 14th day (P > 0.05). On the 28th day, significant difference was observed between groups A1 and A2, which confirmed the effectiveness of LIPUS on fracture healing (P ≤ 0.05). At the same time, despite the higher mean scores in group B2, no significant difference was observed between B1 - B2 (P > 0.05). On day 60 the mean scores did not show significant difference between B1 and B2 (P > 0.05) (Table 3) (Figures 7 and 8).
Mean ± Standard Deviation of Computerized Tomography Images Scoring Based on Density of New Bone Tissue in Different Groups
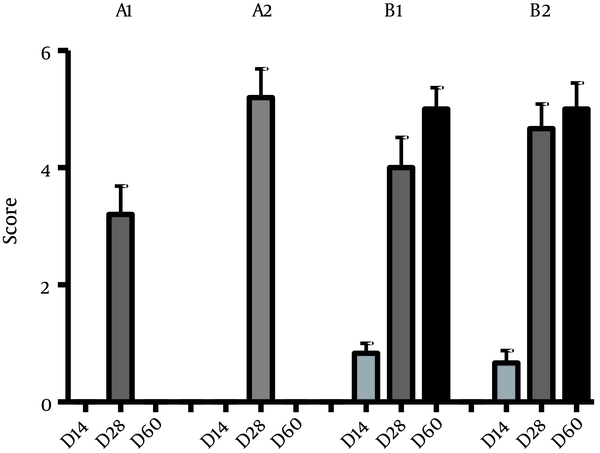
Computed Tomogram of Bony Defect
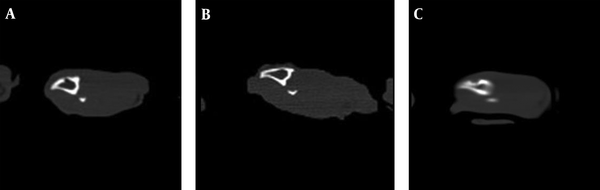
5. Discussion
In this study the effect of DBM in combination with LIPUS treatment was investigated on tibial fracture healing in a rabbit model. Use of LIPUS for treatment of diseases was investigated in the last two decades and defined as an effective method in several studies (12-14). Due to hazardous effect of high intensity ultrasound (US) on osseous tissue and fracture healing, low intensity US was used in the present study (15). The mechanism of LIPUS on fracture healing has not clearly been defined but the induced metabolic alteration causes a decrease in the treatment period, brings more comfort to patients and also increases volume of new bone formation. One of the possible mechanisms is the effect of LIPUS on BMP. Huang et al., in 2015 investigated the effect of LIPUS (30 mW/cm2, 1.5 MHz, 1:4 duty cycle) on osteoblastic cell culture and showed an increase in expression of BMP2 (16). Wijdicks et al. (2009) studied ultrasound effect on recombinant human BMP-2 in a rat model. They used absorbable collagen sponges loaded with 0-, 1-, 2.5- or 5-mg doses of rhBMP-2, which were implanted subcutaneously. Finally they found that, at two weeks, LIPUS had no effect on rhBMP-2-induced bone formation, but at four weeks, LIPUS increased bone volume in the 1-mg and in the 5-mg dose rhBMP-2–treated implants. Tissue density was reduced at both two and four weeks (17). Other studies have confirmed US effect on BMP (18, 19). Another explained mechanism is the effect of US on fracture healing by increasing TGF-β1, IGF, VEGF and FGF (20-22). Here we hypothesized that ultrasound therapy can affect the potency of osteogenesis with DBM. In this regards, we used an experimental rabbit model due to the similarity of rabbit bone structure to the human bone tissue (23).
Results of the healing process in non-grafted group indicated the effectiveness of ultrasound on bone repair, which is in line with previous studies (24, 25). Furthermore, the use of ultrasound in the DBM group with the profile of 1 MHz and intensity of 30 mW/cm2 and 20 minutes/day scheme, caused a slight (non-significant) delay in the healing process. This finding is in contrast with studies that showed a positive effect of ultrasound on fracture healing and osseous implants (12, 26). However, our results are similar with the findings of other researchers, who showed no effect for ultrasound on fracture healing such as Lubbert et al. (27), Emami et al. (28) and Handolin et al. (29). The systemic effect of ultrasound can be counted as a possible explanation for the non-significant results in the treatment and control groups. In this regard, Tsai et al. studied osteotomy operation on fibular bone in rabbits and showed ultrasound therapy improves bone growth on the opposite side of the treated fracture. Chung et al. reported new bone formation on contralateral side (sham group) by using ultrasound therapy in osteotomy (30-32). On the other hand, many researchers have tried to consider the systemic effect of ultrasound on osteoporosis disease, yet their efforts have not been successful. This raises the question of why US has a different influence on fractured and intact bone. The possible explanation is different effect of ultrasound on bone fractures and intact bones in osteoporosis. In intact bones, US energy rapidly attenuates at the soft tissue–bone interface. Thus, the ultrasonic effect might only influence cells on the periosteal surface and in the outermost layers of the bone cortex. The integrity of the bone cortex is lost in fractured bone and the attenuated effect at the soft tissue-bone interface is reduced in these areas. Therefore, ultrasonic effects might have a greater opportunity to influence bone formation through the fracture gap of the bone (33). For enhancing contralateral bone formation, growth factors may play an important role. Growth factors such as bone morphogenetic proteins (BMPs) and transforming growth factor (TGF)-β can regulate and stimulate bone formation. To investigate this hypothesis, further studies and measuring the BMP and TGF plasma level are required after treatment with ultrasound (31).
In this study the DBM putty was used due to its ability in better filling of defects. A study by Kiely et al. showed no differences in bone strength between putty and powder forms of DBM (34). In radiographic and CT examinations, it has been shown that particles of DBM have remained in defect for a long time, which means a reduction in DBM absorption. Decreased osteoclast activity by low intensity pulsed ultrasound can be a reason for this observation (35).
On the other hand, radiology results revealed that the rate of fracture healing in non-grafted group is higher than the DBM group on day 28. Pietrzak showed that BMP concentration could significantly effect osteogenesis potential of DBM (36). In this study, BMP concentration was not defined in DBM, which can be counted as a limitation of the present study. After formulation and processing, osteoinduction potential of DBM was assessed by using standard intra-muscular implants to induce bone formation in a non-bony structure that had already been proven as an indicator for the presence of biologically active BMP (37). Some studies have shown that a different concentration of BMP depends on the donor bone, such as age, gender and other factors (38). However, Trainedes claimed that the processing method might cause different results from the DBM products (39). Currently, there is no standard way to control power of induction of bone formation. There is no standard test to investigate the biological activity of these substances, which could assist us in explaining the differences of observations (37).
This study also had some limitations including lack of biomechanical method to evaluate the power tolerance in fracture spot. Another issue was the lack of inactive ultrasound in contralateral side; in the ideal situation this group should be used as a placebo group.
5.1. Conclusion
Ultrasound waves with low intensity is effective in bone healing process and it can be used as a non-invasive and cheap method for fracture healing but we propose using bone implants with ultrasound due to delay in bone formation.
Acknowledgements
References
-
1.
Gauthier O, Muller R, von Stechow D, Lamy B, Weiss P, Bouler JM, et al. In vivo bone regeneration with injectable calcium phosphate biomaterial: a three-dimensional micro-computed tomographic, biomechanical and SEM study. Biomaterials. 2005;26(27):5444-53. [PubMed ID: 15860201]. https://doi.org/10.1016/j.biomaterials.2005.01.072.
-
2.
Haug RH, Schwimmer A. Fibrous union of the mandible: a review of 27 patients. J Oral Maxillofac Surg. 1994;52(8):832-9. [PubMed ID: 8040737].
-
3.
Jahangir AANRM, Mehta S, Sharan A. Bone- graft substitutes in orthopaedic surgery. Am Acad Orthop Surg; 2009. Available from: http://www.aaos.org/news/aaosnow/jon08/reimbursement2.asp.
-
4.
Wol finbarger L, Eisenlohr LM, Ruth K. Maximizing new bone formation for successful bone implantation. Musculoskeletel Tissue Regeneration Biological Materials and Methods. Humana press. 2006:93-119.
-
5.
Pietrzak WS, Perns SV, Keyes J, Woodell-May J, McDonald NM. Demineralized bone matrix graft: a scientific and clinical case study assessment. J Foot Ankle Surg. 2005;44(5):345-53. [PubMed ID: 16210154]. https://doi.org/10.1053/j.jfas.2005.07.006.
-
6.
Renno AC, Favaro-Pipi E, Fernandes K, Tim C, Ribeiro DA. Ultrasound therapy modulates osteocalcin expression during bone repair in rats. Ultrasonics. 2012;52(1):111-6. [PubMed ID: 21820693]. https://doi.org/10.1016/j.ultras.2011.07.002.
-
7.
Claes L, Willie B. The enhancement of bone regeneration by ultrasound. Prog Biophys Mol Biol. 2007;93(1 - 3):384-98. [PubMed ID: 16934857]. https://doi.org/10.1016/j.pbiomolbio.2006.07.021.
-
8.
Nolte PA, Klein-Nulend J, Albers GH, Marti RK, Semeins CM, Goei SW, et al. Low-intensity ultrasound stimulates endochondral ossification in vitro. J Orthop Res. 2001;19(2):301-7. [PubMed ID: 11347705]. https://doi.org/10.1016/S0736-0266(00)00027-9.
-
9.
Skerry TM. The response of bone to mechanical loading and disuse: fundamental principles and influences on osteoblast/osteocyte homeostasis. Arch Biochem Biophys. 2008;473(2):117-23. [PubMed ID: 18334226]. https://doi.org/10.1016/j.abb.2008.02.028.
-
10.
Dyson M. Mechanisms involved in therapeutic ultrasound. Physiotherapy. 1987;73(3):116-20.
-
11.
Xie LK, Wangrangsimakul K, Suttapreyasri S, Cheung LK, Nuntanaranont T. A preliminary study of the effect of low intensity pulsed ultrasound on new bone formation during mandibular distraction osteogenesis in rabbits. Int J Oral Maxillofac Surg. 2011;40(7):730-6. [PubMed ID: 21550207]. https://doi.org/10.1016/j.ijom.2011.03.016.
-
12.
Akiyama H, Hachiya Y, Otsuka H, Kurisuno M, Kawanabe K, Katayama N, et al. Low-intensity pulsed ultrasound therapy stimulates callus formation between host femur and cortical onlay strut allograft. Ultrasound Med Biol. 2014;40(6):1197-203. [PubMed ID: 24556560]. https://doi.org/10.1016/j.ultrasmedbio.2013.12.030.
-
13.
Maeda T, Masaki C, Kanao M, Kondo Y, Ohta A, Nakamoto T, et al. Low-intensity pulsed ultrasound enhances palatal mucosa wound healing in rats. J Prosthodont Res. 2013;57(2):93-8. [PubMed ID: 23270778]. https://doi.org/10.1016/j.jpor.2012.11.001.
-
14.
Shi M, Liu B, Liu G, Wang P, Yang M, Li Y, et al. Low intensity-pulsed ultrasound induced apoptosis of human hepatocellular carcinoma cells in vitro. Ultrasonics. 2016;64:43-53. [PubMed ID: 26231998]. https://doi.org/10.1016/j.ultras.2015.07.011.
-
15.
Lyon R, Liu XC, Meier J. The effects of therapeutic vs. high-intensity ultrasound on the rabbit growth plate. J Orthop Res. 2003;21(5):865-71. [PubMed ID: 12919875]. https://doi.org/10.1016/S0736-0266(03)00047-0.
-
16.
Huang W, Hasegawa T, Imai Y, Takeda D, Akashi M, Komori T. Low-intensity pulsed ultrasound enhances bone morphogenetic protein expression of human mandibular fracture haematoma-derived cells. Int J Oral Maxillofac Surg. 2015;44(7):929-35. [PubMed ID: 25835758]. https://doi.org/10.1016/j.ijom.2015.03.001.
-
17.
Wijdicks CA, Virdi AS, Sena K, Sumner DR, Leven RM. Ultrasound enhances recombinant human BMP-2 induced ectopic bone formation in a rat model. Ultrasound Med Biol. 2009;35(10):1629-37. [PubMed ID: 19632764]. https://doi.org/10.1016/j.ultrasmedbio.2009.04.017.
-
18.
Hou CH, Hou SM, Tang CH. Ultrasound increased BMP-2 expression via PI3K, Akt, c-Fos/c-Jun, and AP-1 pathways in cultured osteoblasts. J Cell Biochem. 2009;106(1):7-15. [PubMed ID: 19009553]. https://doi.org/10.1002/jcb.21934.
-
19.
Xue H, Zheng J, Cui Z, Bai X, Li G, Zhang C, et al. Low-intensity pulsed ultrasound accelerates tooth movement via activation of the BMP-2 signaling pathway. PLoS One. 2013;8(7):68926. [PubMed ID: 23894376]. https://doi.org/10.1371/journal.pone.0068926.
-
20.
Lu H, Qin L, Cheung W, Lee K, Wong W, Leung K. Low-intensity pulsed ultrasound accelerated bone-tendon junction healing through regulation of vascular endothelial growth factor expression and cartilage formation. Ultrasound Med Biol. 2008;34(8):1248-60. [PubMed ID: 18378382]. https://doi.org/10.1016/j.ultrasmedbio.2008.01.009.
-
21.
Mukai S, Ito H, Nakagawa Y, Akiyama H, Miyamoto M, Nakamura T. Transforming growth factor-beta1 mediates the effects of low-intensity pulsed ultrasound in chondrocytes. Ultrasound Med Biol. 2005;31(12):1713-21. [PubMed ID: 16344134]. https://doi.org/10.1016/j.ultrasmedbio.2005.07.012.
-
22.
Marrerol A, Enizl D. Low intensity pulsed ultrasound produces and increase of IGF-I gene expression during fibular bone repair in rats. Growth Horm IGF res. 2012;22:S33-88.
-
23.
Li Y, Chen SK, Li L, Qin L, Wang XL, Lai YX. Bone defect animal models for testing efficacy of bone substitute biomaterials. J Orthopaedic Translation. 2015;3(3):95-104.
-
24.
Babuccu C, Keklikoglu N, Baydogan M, Kaynar A. Cumulative effect of low-level laser therapy and low-intensity pulsed ultrasound on bone repair in rats. Int J Oral Maxillofac Surg. 2014;43(6):769-76. [PubMed ID: 24467933]. https://doi.org/10.1016/j.ijom.2013.12.002.
-
25.
Zura R, Della Rocca GJ, Mehta S, Harrison A, Brodie C, Jones J, et al. Treatment of chronic (>1 year) fracture nonunion: heal rate in a cohort of 767 patients treated with low-intensity pulsed ultrasound (LIPUS). Injury. 2015;46(10):2036-41. [PubMed ID: 26052056]. https://doi.org/10.1016/j.injury.2015.05.042.
-
26.
Wang J, Li JW, Chen L. Effect of low-intensity pulsed ultrasound on posterolateral lumbar fusion of rabbit. Asian Pac J Trop Med. 2015;8(1):68-72. [PubMed ID: 25901928]. https://doi.org/10.1016/S1995-7645(14)60190-5.
-
27.
Lubbert PH, van der Rijt RH, Hoorntje LE, van der Werken C. Low-intensity pulsed ultrasound (LIPUS) in fresh clavicle fractures: a multi-centre double blind randomised controlled trial. Injury. 2008;39(12):1444-52. [PubMed ID: 18656872]. https://doi.org/10.1016/j.injury.2008.04.004.
-
28.
Emami A, Petren-Mallmin M, Larsson S. No effect of low-intensity ultrasound on healing time of intramedullary fixed tibial fractures. J Orthop Trauma. 1999;13(4):252-7. [PubMed ID: 10342350].
-
29.
Handolin L, Kiljunen V, Arnala I, Kiuru MJ, Pajarinen J, Partio EK, et al. No long-term effects of ultrasound therapy on bioabsorbable screw-fixed lateral malleolar fracture. Scand J Surg. 2005;94(3):239-42. [PubMed ID: 16259175].
-
30.
Chang WH, Sun JS, Chang SP, Lin JC. Study of thermal effects of ultrasound stimulation on fracture healing. Bioelectromagnetics. 2002;23(4):256-63. [PubMed ID: 11948604].
-
31.
Lai CH, Chuang CC, Li JK, Chen SC, Chang WH. Effects of ultrasound on osteotomy healing in a rabbit fracture model. Ultrasound Med Biol. 2011;37(10):1635-43. [PubMed ID: 21821345]. https://doi.org/10.1016/j.ultrasmedbio.2011.06.014.
-
32.
Tsai CL, Chang WH, Liu TK, Song GM. Ultrasound can affect bone healing both locally and systemically. Chin J Physiol. 1991;34(2):213-22. [PubMed ID: 1959440].
-
33.
Warden SJ, Bennell KL, Matthews B, Brown DJ, McMeeken JM, Wark JD. Efficacy of low-intensity pulsed ultrasound in the prevention of osteoporosis following spinal cord injury. Bone. 2001;29(5):431-6. [PubMed ID: 11704494].
-
34.
Kiely PD, Brecevich AT, Taher F, Nguyen JT, Cammisa FP, Abjornson C. Evaluation of a new formulation of demineralized bone matrix putty in a rabbit posterolateral spinal fusion model. Spine J. 2014;14(9):2155-63. [PubMed ID: 24512696]. https://doi.org/10.1016/j.spinee.2014.01.053.
-
35.
Hu B, Cai XZ, Shi ZL, Chen YL, Zhao X, Zhu HX, et al. Microbubble injection enhances inhibition of low-intensity pulsed ultrasound on debris-induced periprosthetic osteolysis in rabbit model. Ultrasound Med Biol. 2015;41(1):177-86. [PubMed ID: 25438844]. https://doi.org/10.1016/j.ultrasmedbio.2014.08.016.
-
36.
Pietrzak WS, Woodell-May J, McDonald N. Assay of bone morphogenetic protein-2, -4, and -7 in human demineralized bone matrix. J Craniofac Surg. 2006;17(1):84-90. [PubMed ID: 16432413]. https://doi.org/10.1097/01.scs.0000179745.91165.73.
-
37.
Edwards JT, Diegmann MH, Scarborough NL. Osteoinduction of human demineralized bone: characterization in a rat model. Clin Orthop Relat Res. 1998;(357):219-28. [PubMed ID: 9917720].
-
38.
Wang JC, Alanay A, Mark D, Kanim LE, Campbell PA, Dawson EG, et al. A comparison of commercially available demineralized bone matrix for spinal fusion. Eur Spine J. 2007;16(8):1233-40. [PubMed ID: 17205237]. https://doi.org/10.1007/s00586-006-0282-x.
-
39.
Traianedes K, Russell JL, Edwards JT, Stubbs HA, Shanahan IR, Knaack D. Donor age and gender effects on osteoinductivity of demineralized bone matrix. J Biomed Mater Res B Appl Biomater. 2004;70(1):21-9. [PubMed ID: 15199579]. https://doi.org/10.1002/jbm.b.30015.