Abstract
Background:
Salvia officinalis is a phytoestrogen, which is used to reduce clinical signs of pregnancy and improve the female infertility in herbal medicine treatment. The effect of S. officinalis on ovarian angiogenesis at the preimplantation time is unknown.Objectives:
This research was a histomorphometric and quantitative real-time study on angiogenic activity in the ovarian tissue in mice after exposure to S. officinalis at preimplantaion.Methods:
The extract of S. officinalis leaves, which was analyzed by phytochemical methods, administered at a dose of 100 mg/kg daily for 14 days to female mice. Then, pseudopregnancy was induced and ovarian tissue samples were removed for histological and molecular assessment.Results:
Data analysis on the space-solid phase micro extraction/gas chromatography-mass spectrometry demonstrated α-thujone (33.90%), camphor (19.34%), cineole (11.01%) and ß-thujone (9.48%) were the dominating compounds in the leaves of S. officinalis. Data analysis on histomorphometric parameters showed a significant difference in the vascular parameters between the test and control groups. Furthermore, gene expression analysis implied an increased level of the vascular endothelial growth factor (VEGF) (3.175) and was also associated with an increase in the level of Kinase insert domain protein receptor (KDR/Flk) gene expression (3.556) and FMS-like tyrosine kinase (Flt) gene expression (3.437) in the test group compared to the control group.Conclusions:
Increased ovarian angiogenesis after exposure to S. officinalis may induce vascular permeability and finally contribute to a variety of disorders including clinical signs similar to the ovarian hyperstimulation syndrome.Keywords
Salvia officinalis Phytoestrogen Ovary Angiogenesis Preimplantation Pregnancy
1. Background
Angiogenesis is the formation of new blood vessels (1). Two VEGF receptors, VEGF-R1 [Flt-1] and VEGFR-2 [KDR/Flk-1], were also identified in the ovarian tissue (2). The VEGF-R2 receptor appears to be mainly involved in regulation of angiogenesis and vasculogenesis (3). The expression of angiogenic factors in the reproduction system is hormone-dependent. Ovarian endothelium is a primary target of gonadotropins and locally synthetized steroids (4). Salvia is the largest and the most important genus of the family Lamiacea (5). Diverse biological activities of this plant were reported including anti-inflammatory, antioxidant, antibacterial, fungistatic, virustatic, antiprotease, and antimetastatic features (6). Salvia officinalis consists of steroid and isoflavonoids, which are considered as phytoestrogens (7). Researchers have shown an increase in the estrogen receptors expression in the mammarian glands of mice after the genistein treatment (8). It was demonstrated that luteolin as the common and putative estrogenic principle flavonoid in the S. officinalis or sage acts as an antihot flush during the menopause (9). It was reported that isoflavones reduced a risk of cancer and flavonoid component had the main effect on menopausal symptoms (10). The medicinal property of sage is valuable as a therapy in reducing menopausal symptoms (11) and for postmenopausal women who travailed from estrogen poorness (12). This plant is generally used among Palestinian women to ameliorate the clinical signs of pregnancy (13). The hydroalcoholic extract of sage stimulated the growth of the mammarian gland in adult female rats, which could be due to estrogenic compounds of sage (14). Phytoestrogens are nonsteroidal components of plants that bind to α and β estrogen receptors and act similar to 17-β estradiol (15). During recent years, more attention has been focused on an angiogenic effect of S. officinalis (16). An anti-angiogenic activity of S. officinalis has also been used for cancer treatment (17). It is true that female infertility could be improved a success rate of the IVF and pregnancy in the herbal medicine treatment (18, 19). However, the angiogenic effect of the ethanolic extract of medicinal plants such as S. officinalis on the ovarian tissue at the preimplantation time is unknown. To provide the best care to pregnant women who use herbal products, we assessed the angiogenic effect of S. officinalis on ovarian VEGF gene expression and its receptors at the pre-implantation time.
2. Objectives
This study was a histomorphometric and quantitative real-time study on angiogenic activity in the ovarian tissue in mice after exposure to S. officinalis at preimplantaion.
3. Methods
3.1. Plant Material
In this study, S. officinalis (Herbarium Code MPH-826) leaves were extracted by an ultrasonic bath method and then the samples were concentrated by a rotary evaporator.
3.2. Gas Chromatography-Mass Spectrometry
To perform gas chromatography-mass spectrometry, a gas chromatograph was equipped with an agilent 6890N coupled to an agilent 5973 mass detector, a manual injection port opening in a spiltless mode and an HP-5 Column30m (length) × 0.25 mm (ID) × 0.25 µm. Working statements were injector: 250°C; gas carrier: He (99.999%), pressure of column head, 5 psi. Wiley 7n was selected as a library and an injector temperature was defined at 250°C. The flow rate of GC was 0.9 mL/min. Temperature programming of column was from 50°C to 255°C; heating rate: 5°C/min at 170°C and 10°C/min at 255°C. Samples (0.5 g) of the fresh leaves of S. officinalis were placed in a glass vial, which were then closed with the Viton septa and aluminium seals provided by Supelco (Sigma-Aldrich, US). The vials containing the samples were sonicated in a thermostatic water bath (Euronda sonication instrument, Italy) at 60°C for 10 minutes). After 10 minutes, the solid-phase microextraction (SPME) tool was entered into the locked vial by the manually pervasive septum, and SPME staple: PDMS 100 µm thickness (Supelco, Sigma-Aldrich) was exposed to the sample headspace for 15 minutes. The needle on the SPME hand-operated preservative was put in the GC injector and the staple was directly subjected to the hot injector at 250°C for 3 minutes in the splitless method.
3.3. Animals
The adult female mice with an average 6 - 8 weeks old were allocated into two groups (control and test groups). First, pseudopregnancy was induced in the mice of the control group. Then, the adult female mice in the test group received the extract of the S. officinalis leaves at a dose of 100 mg/kg daily for 14 days. Finally, pseudopregnancy was induced in this group.
3.4. Tissue Preparation
Mice were sacrificed by cervical dislocation at the preimplantation time. Ovarian tissue samples were collected for histological and molecular assessment. After staining with hematoxylin and eosin for evaluation of vascular density and intravascular area, the Motic Image Plus2 software was served to measure the quantitative vascular parameters. Sections were selected and analyzed with magnification × 400.
3.5. RNA Isolation and cDNA Synthesis
To determine the VEGF, FLK and FLT expression, RNA extraction was performed by the RNeasy Mini Kit (Qiagen Cat. No. 19306). The cDNA synthesis was accomplished by the unique quanti tect reverse transcription kit (QIAGEN Cat. NO. 205311 thermo scientific K 1621 Lot 00124084). A list of mouse primers utilized in this study was depicted in Table 1.
Mouse Primers Used in Real-Time Polymerase Chain Reaction Studies
Gene | Sequence 5’ 3’ | Lenght | Annealing Temperature | Gene Bank Code |
---|---|---|---|---|
Real-time RT-PCR primers | ||||
Glyceraldehyde-3-phosphate dehydrogenase Gapdh | F: ATCACTGCCACCCAGAAGAC | 20 | 59.67 | NM-001289726.1 |
R: AGATCCACGACGGACACATT | 20 | 59.10 | ||
Vascular endothelial growth factor A(VEGF) | F: GGAGACTCTTCGAGGAGCACTT | 22 | 61.46 | NM-001110268.1 |
R: GGCGATTTAGCAGCAGATATAAGAA | 25 | 59.36 | ||
FMS-like tyrosine kinase 1 (FLT1) | F: GAGGAGGATGAGGGTGTCTA | 20 | 57.24 | NM-010228.3 |
R: GTGATCAGCTCCAGGTTTGA | 20 | 57.52 | ||
Kinase insert domain protein receptor (KDR) | F: GGCGGTGGTGACAGTATCTT | 20 | 59.75 | NM-010612.2 |
R: GAGGCGATGAATGGTGATCT | 20 | 57.46 |
3.6. Real-Time Polymerase Chain Reaction
To perform the gene expression analysis, 40 reaction amplification cycles (each reaction contains 15 seconds at 95°C and 60 seconds at 60°C) were performed using the Rotor-Gene Q by SYBR Green detection. The GAPDH gene was used as an internal control.
3.7. Statistical Analysis
The data obtained from the histological study were analyzed using the Mann-Whitney test by the SPSS software. Results obtained from real-time polymerase chain reaction (PCR) were analyzed using the Lin Reg PCR and Rest-RG softwares.
4. Results
4.1. The Solid-Phase Microextraction Analysis
The results obtained from SPME/GC- MS indicated the most percentage of volatile components of leaves of S. officinalis were α-thujone (33.90%) as a monoterpene, camphor (19.34%) as a terpenoid, cineole (11.01%) as monoterpenoid and ß-thujone (9.48%) as a monoterpene, respectively. Our SPME/GC- MS composition data of leaves of S. officinalis were collected in Table 2 and the SPME/GC- MS chromatogram of the leaves of S. officinalis were also depicted in Figure 1.
Composition of the Solid-Phase Microextraction Analysis in the Leaves of the Salvia officinalis
Retention Time | Compound | Area | % |
---|---|---|---|
5.817 | Tricyclene | 1123574 | 0.17 |
6.103 | α-Pinene | 691716 | 0.11 |
6.463 | Camphene | 17243888 | 2.63 |
6.6 | Verbenene | 7645886 | 1.17 |
7.074 | Sabinene | 273991 | 0.04 |
7.16 | β-pinene | 2631161 | 0.40 |
7.406 | 1-Octen-3-ol | 5554296 | 0.85 |
7.503 | β-Myrcene | 2528123 | 0.39 |
8.017 | 3-Carene | 1834837 | 0.28 |
8.212 | α-Terpinene | 323102 | 0.05 |
8.446 | P-Cymene | 4737420 | 0.72 |
8.772 | 1,8-Cineole | 72144659 | 11.01 |
8.749 | cis-Ocimene | 8459877 | 1.29 |
9.035 | trans-.beta.-Ocimene | 1624794 | 0.25 |
9.36 | γ-Terpinene | 689294 | 0.11 |
9.72 | trans-Sabinene hydrate | 1773354 | 0.27 |
10.166 | Terpinolen | 1464675 | 0.22 |
10.641 | Linalool | 8138116 | 1.24 |
10.892 | α-Thujone | 222121547 | 33.90 |
11.621 | β-Thujone | 62144659 | 9.48 |
11.944 | Camphor | 126723451 | 19.34 |
12.069 | Isomenthone | 6908140 | 1.05 |
12.327 | Menthofuran | 5947438 | 0.91 |
12.715 | Borneol | 35870525 | 5.47 |
12.881 | 4-Terpineol | 1150700 | 0.18 |
13.738 | Eucarvone | 1320306 | 0.20 |
14.15 | Isobornyl acetate | 4610527 | 0.70 |
14.584 | Cuminic aldehyde | 757869 | 0.12 |
14.67 | Carvone | 766506 | 0.12 |
14.83 | Linalyl acetate | 8715699 | 1.33 |
15.699 | Bornyl acetate | 6793171 | 1.04 |
15.887 | Menthyl acetate | 8488008 | 1.30 |
18.528 | β-elemene | 1861521 | 0.28 |
18.956 | α-Gurjunene | 463254 | 0.07 |
19.248 | trans-Caryophyllene | 6052134 | 0.92 |
20.071 | Trans-beta-Farnesene | 5246325 | 0.80 |
20.808 | Germacrene D | 3747971 | 0.57 |
21.368 | Geranyl acetate | 1523409 | 0.23 |
23.357 | Caryophyllene oxide | 3231570 | 0.49 |
24.74 | α-Cadinol | 1923542 | 0.29 |
The SPME Chromatogram of Salvia officinalis (leaves) From the Fars Province Sample.
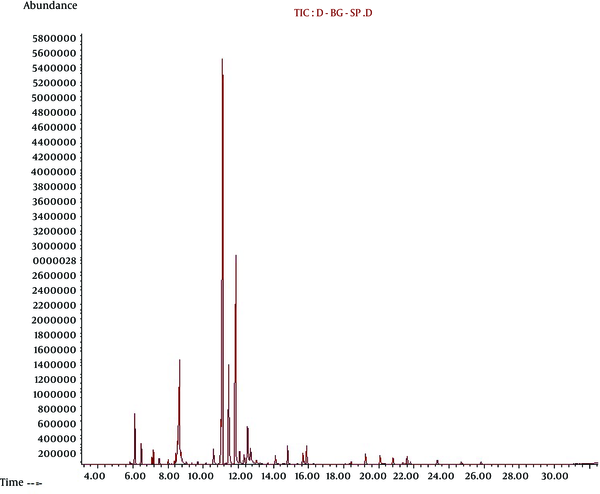
4.2. Histological Findings
The results of the histological parameters of ovarian angiogenesis revealed a significant difference in the vascular parameters between the two groups at the preimplantation time. Comparison of the data of the quantitative vascular parameters are stated as P < 0.05, significantly different from the control group in the ovarian tissue of the mice at the preimplantation time. Vascular density of the test group (11.42 ± 1.08) was significantly different from the control group (3.83 ± 0.36). Also, an intravascular area of the test group (132.80 ± 3.55) was significantly different from the control group (18.07 ± 1.27). Histological morphology was also indicative of increased vascular sections in the test group compared to the control group. Vascular sections were specially seen around and inside the corpus luteum and also were seen around the primary and secondary follicles. Histological data were depicted in Table 3 and Figure 2.
Representative comparison of vascular density and intravascular area of the ovarian tissue between the control and test groups after treatment of S. officinalis with a light microscopy, magnification was × 400. There was a significant difference in vascular density between the control and test groups, (P ≤ 0.05). A significant difference was also observed in the intravascular area between the control and test groups at the preimplantation time. Arrows show the vascular section in each group. A, Vascular density and intravascular area in the control group. B, Vascular density and intravascular area in the test group.
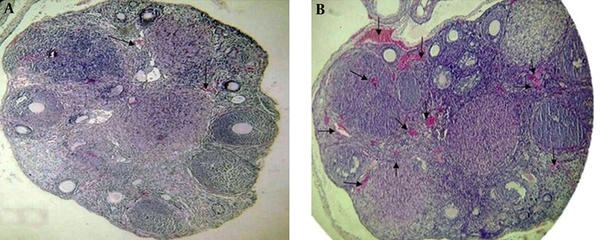
4.3. Real-Time Polymerase Chain Reaction
Comparison of VEGF, KDR, and FLT gene expressions between the two groups showed an increased VEGF gene expression level (3.175), increased KDR gene expression level (3.556) and FLT gene expression level (3.437). Results of gene expression analysis of these genes were shown in Figure 3.
Quantitative expression analysis of the VEGF, KDR and FLT in the ovary obtained from the control and test groups. The rest-RG software was used to generate the graph.
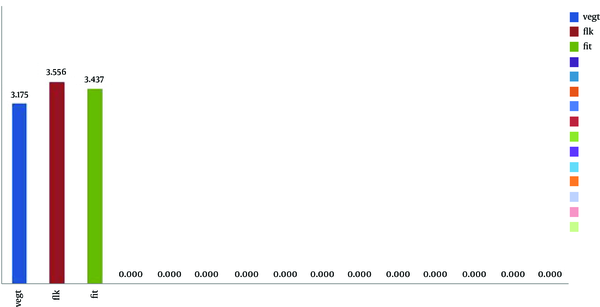
5. Discussion
Histological findings of our study demonstrated a significant difference in the vascular density and also intravascular area of an ovarian tissue between the two groups. It was concluded that the ethanolic extract of the leaves of S. officinalis was resulted in elevated angiogenesis in the ovarian tissue at the preimplantation time.
Researchers showed that the ethanolic extract of S. officinalis and its hexan fraction had a therapeutic role against angiogenesis on a three-dimensional culture of the aorta model of rat and human umblical vein endothelial cells (20). Also, other researchers indicated an antiangiogenic activity of S. officinalis related to ursolic acid component (21). Over the recent studies, more attention has been focused on the antiangiogenic and antimetastatic effects of compounds from S. officinalis. However, the results of the present study showed that the ethanolic extract of S. officinalis resulted in induction of angiogenesis in the ovarian tissue via increased VEGF gene expression and its receptors. These reports are in contradiction with the results of other studies, which have shown the anti-angiogenic activity of S. officinalis (22).
Our molecular data on the VEGF gene expression and more importantly its receptors, FLK and Flt receptors, confirmed our histological findings. Quantitative comparison of the ovarian VEGF gene expression and its receptors after S. officinalis treatment indicated a positive expression in the test group contrasted to the control group.
Antiangiogenic components of the S. officinalis were known to inhibit protease, suppress receptor phosphorylation or disrupt endothelial tube formation related to interference of cytoskeleton organization (23). The current study showed that the gene expression following S. officinalis treatment caused a decrease in the gene expression and an increase in the Flk and Flt receptors in the endometrium of the pseudopregnant mice at the preimplantation time (24). It was also revealed that progesterone treatment after ovarian induction led to an increase in the VEGF gene expression and its receptors at the preimplantation time (25).
Considered together, it may be a biological activity of S. officinalis on the vascular changes related to anti-inflammatory compounds (26) of this plant done through prostaglandin inhibition by phenolic diterpenes such as carnosol and carnosic acids (27), triterpenes such as ursolic acid (28) and other terpenoid components (29). Phenolic diterpenes such as carnosol and carnosic acids were determined in the HPLC analysis of the ethanolic extract of S. officinalis in our recent published data (24). Moreover, the SPME analysis in the present study showed other major components of the leaves of S. officinalis, which have an anti-inflammatory property such as α-thujone (33.90%), ß-thujone (9.48%), camphor (19.34%) and cineole (11.01%). The mechanism of anti-inflammatory compounds mentioned above is as same as nonsteroidal anti-inflammatory drugs (NSAIDS) causing prostaglandin inhibition and resulting in increased corpus luteum, which leads to maintenance of pregnancy (30).
Therefore, it seems that some phytoestrogenic agents of S. officinalis may affect the hormonal levels such as human chorionic gonadotropin (HCG), which is the prime mediator involved in increased VEGF gene expression. There are several mechanisms involved in the upregulation of the VEGF gene expression and its receptors. It is mentioned that hypoxia via hypoxia inducing factor, cytokine and growth factors such as interleukin-6 and the presence of some bioactive molecules in the ascite fluid upregulate VEGF gene expression (4). Other researchers indicated that HCG is as the prime mediator causing an increase in the VEGF gene expression containing some hypophyseal hormones involved in ovarian angiogenesis (31).
The results of the present study showed that administration of the ethanolic extract of S. officinalis resulted in an increased angiogenesis. It may be related to ovarian hormone changes after S. officinalis treatment. The presence of the HCG receptor on microvascular endothelial cells as the primary target of HCG resulting in an acute release of VEGF, consequently can affect the KDR expression and finally a significant increase will happen in cytokines in an autocrine-paracrine outcome, which may increase vascular permeability (32). It was declared that increased VEGF may induce vascular permeability but it may not be directly involved in the massive fluid transport as in severe OHSS (2). In the present study, we did not observe a massive fluid transport although increased VEGF gene expression in the ovarian tissue may be associated with vascular permeability. Another study demonstrated that granulosa lutein cells and endothelial cells, which are sources of the VEGF production, may be involved in OHSS development (33). Therefore, changes in ovarian angiogenesis can be considered as one of the adverse effects of using ethanolic extract of S.officinalis on ovarian tissue at the preimplantation time because VEGF and VPF are correlative with each other for normal fertile. An adverse effect of the sage oil reported in a newborn and a child causing seizures after even a single ingested dose of S. officinalis can be proposed as an alarming report (34). In addition, it is well-known that LH is an activator of adenylate cyclase and acts as a stimulator on ovarian angiogenesis in the granulosa cells of primate (35). The VEGF mRNA is expressed in several sites of ovarian tissue at multiple stages. More importantly, luteinized granulosa and thecal cells express VEGF at early pregnancy. Thus, overincitement of the VEGF expression is created by an increased level of gonadotropin and ovarian hormones (36, 37). So, regarding the effect of the ethanolic extract of S. officinalis on a luteinizing hormone, it can be proposed as one of the mechanism involving increased angiogenesis in the ovarian tissue.
In conclusion, herbs may have an unrecognized effect on pregnancy and serious complication on the fetus. Natural plants products have been used since ancient times and are now increasing. In fact, different tissues have diverse responses of stromal cells in each tissue depending on the number of cell surface receptors on these cells. Ovarian tissue is no exception. Each of these stromal cells could directly or indirectly affect the microenviroment tissue, which itself could change the vascular gene expression. Increased ovarian angiogenesis after the S. officinalis administration may induce vascular permeability and involve in developing some disorders such are anovulation, infertility, miscarriage and clinical signs similar to the ovarian hyperstimulation syndrome. To provide the best care to a pregnant woman who uses herbal products, we recommend that scientists stay up to date with their safety in pregnancy. Further research is needed to elucidate the potential and beneficial role of the responsible component of S. officinalis involved in the ovarian angiogenesis at the preimplantation.
Acknowledgements
References
-
1.
Zihlif M, Afifi F, Abu-Dahab R, Abdul Majid AM, Somrain H, Saleh MM, et al. The antiangiogenic activities of ethanolic crude extracts of four Salvia species. BMC Complement Altern Med. 2013;13:358. [PubMed ID: 24330494]. https://doi.org/10.1186/1472-6882-13-358.
-
2.
Ajonuma LC. Is vascular endothelial growth factor (VEGF) the main mediator in ovarian hyperstimulation syndrome (OHSS)? Med Hypotheses. 2008;70(6):1174-8. [PubMed ID: 18164141]. https://doi.org/10.1016/j.mehy.2007.11.004.
-
3.
Gomez R, Simon C, Remohi J, Pellicer A. Vascular endothelial growth factor receptor-2 activation induces vascular permeability in hyperstimulated rats, and this effect is prevented by receptor blockade. Endocrinology. 2002;143(11):4339-48. [PubMed ID: 12399430]. https://doi.org/10.1210/en.2002-220204.
-
4.
Geva E, Jaffe RB. Role of vascular endothelial growth factor in ovarian physiology and pathology. Fertil Steril. 2000;74(3):429-38. [PubMed ID: 10973633].
-
5.
Ciesla L, Hajnos M, Staszek D, Wojtal L, Kowalska T, Waksmundzka-Hajnos M. Validated binary high-performance thin-layer chromatographic fingerprints of polyphenolics for distinguishing different Salvia species. J Chromatogr Sci. 2010;48(6):421-7. [PubMed ID: 20822654].
-
6.
Schwager J, Richard N, Fowler A, Seifert N, Raederstorff D. Carnosol and Related Substances Modulate Chemokine and Cytokine Production in Macrophages and Chondrocytes. Molecules. 2016;21(4):465. [PubMed ID: 27070563]. https://doi.org/10.3390/molecules21040465.
-
7.
Vitale DC, Piazza C, Melilli B, Drago F, Salomone S. Isoflavones: estrogenic activity, biological effect and bioavailability. Eur J Drug Metab Pharmacokinet. 2013;38(1):15-25. [PubMed ID: 23161396]. https://doi.org/10.1007/s13318-012-0112-y.
-
8.
Padilla-Banks E, Jefferson WN, Newbold RR. Neonatal exposure to the phytoestrogen genistein alters mammary gland growth and developmental programming of hormone receptor levels. Endocrinology. 2006;147(10):4871-82. [PubMed ID: 16857750]. https://doi.org/10.1210/en.2006-0389.
-
9.
Rahte S, Evans R, Eugster PJ, Marcourt L, Wolfender JL, Kortenkamp A, et al. Salvia officinalis for hot flushes: towards determination of mechanism of activity and active principles. Planta Med. 2013;79(9):753-60. [PubMed ID: 23670626]. https://doi.org/10.1055/s-0032-1328552.
-
10.
Stojanovi JB, Veljkovi VB. Extraction of flavonoids from garden (Salvia officinalis L.) and glutinous (Salvia glutinosa L.) sage by ultrasonic and classical maceration. J Serb Chem Soc. 2007;72(1):73-80.
-
11.
Denham A, Green J, Hawkey S. What's in the bottle? Prescriptions formulated by medical herbalists in a clinical trial of treatment during the menopause. J Herbal Med. 2011;1(3):95-101.
-
12.
Limopasmanee W, Chansakaow S, Rojanasthien N, Manorot M, Sangdee C, Teekachunhatean S. Effects of the chinese herbal formulation (liu wei di huang wan) on the pharmacokinetics of isoflavones in postmenopausal women. Bio Res Inter. 2015.
-
13.
Al-Ramahi R, Jaradat N, Adawi D. Use of herbal medicines during pregnancy in a group of Palestinian women. J Ethnopharmacol. 2013;150(1):79-84. [PubMed ID: 23933314]. https://doi.org/10.1016/j.jep.2013.07.041.
-
14.
Monsefi M, Abedian M, Azarbahram Z, Ashraf MJ. Salvia officinalis L. induces alveolar bud growing in adult female rat mammary glands. Avicenna J Phytomed. 2015;5(6):560-7. [PubMed ID: 26693413].
-
15.
Ososki AL, Kennelly EJ. Phytoestrogens: a review of the present state of research. Phytother Res. 2003;17(8):845-69. [PubMed ID: 13680814]. https://doi.org/10.1002/ptr.1364.
-
16.
Kayashima T, Matsubara K. Antiangiogenic effect of carnosic acid and carnosol, neuroprotective compounds in rosemary leaves. Biosci Biotechnol Biochem. 2012;76(1):115-9. [PubMed ID: 22232247]. https://doi.org/10.1271/bbb.110584.
-
17.
Kim GD, Park YS, Jin YH, Park CS. Production and applications of rosmarinic acid and structurally related compounds. Appl Microbiol Biotechnol. 2015;99(5):2083-92. [PubMed ID: 25620368]. https://doi.org/10.1007/s00253-015-6395-6.
-
18.
Ried K. Chinese herbal medicine for female infertility: an updated meta-analysis. Complement Ther Med. 2015;23(1):116-28. [PubMed ID: 25637159]. https://doi.org/10.1016/j.ctim.2014.12.004.
-
19.
Guo J, Li D, Liu C, Ji X, Li R, Du X. Effects of Chinese herbs combined with in vitro fertilization and embryo transplantation on infertility: a clinical randomized controlled trial. J Tradit Chin Med. 2014;34(3):267-73. [PubMed ID: 24992752].
-
20.
Keshavarz M, Mostafaie A, Mansouri K, Bidmeshkipour A, Motlagh HR, Parvaneh S. In vitro and ex vivo antiangiogenic activity of Salvia officinalis. Phytother Res. 2010;24(10):1526-31. [PubMed ID: 20878705]. https://doi.org/10.1002/ptr.3168.
-
21.
Kashyap D, Tuli HS, Sharma AK. Ursolic acid (UA): A metabolite with promising therapeutic potential. Life Sci. 2016;146:201-13. [PubMed ID: 26775565]. https://doi.org/10.1016/j.lfs.2016.01.017.
-
22.
Haas J, Ophir L, Barzilay E, Yerushalmi GM, Yung Y, Kedem A, et al. GnRH agonist vs. hCG for triggering of ovulation--differential effects on gene expression in human granulosa cells. PLoS One. 2014;9(3):90359. [PubMed ID: 24603682]. https://doi.org/10.1371/journal.pone.0090359.
-
23.
Keshavarz M, Bidmeshkipour A, Mostafaie A, Mansouri K, Mohammadi-Motlagh HR. Anti tumor activity of Salvia officinalis is due to its anti-angiogenic, anti-migratory and anti-proliferative effects. Cell J. 2011;12(4):477-82.
-
24.
Boroujeni NB, Boroujeni MB, Gholami M, Shafiei A. Ethanolic extract of Salvia officinalis alter vascular endothelial growth factor gene expression and its receptors in the mouse endometrium at preimplantation. Comparative Clin Pathol. 2016;25(1):125-9.
-
25.
Boroujeni MB, Boroujeni NB, Gholami M. The effect of progesterone treatment after ovarian induction on endometrial VEGF gene expression and its receptors in mice at pre-implantation time. Iran J Basic Med Sci. 2016;19(3):252-7. [PubMed ID: 27114794].
-
26.
Albano SM, Miguel MG. Biological activities of extracts of plants grown in Portugal. Indust Crops Products. 2011;33(2):338-43.
-
27.
Bauer J, Kuehnl S, Rollinger JM, Scherer O, Northoff H, Stuppner H, et al. Carnosol and carnosic acids from Salvia officinalis inhibit microsomal prostaglandin E2 synthase-1. J Pharmacol Exp Ther. 2012;342(1):169-76. [PubMed ID: 22511203]. https://doi.org/10.1124/jpet.112.193847.
-
28.
Baricevic D, Sosa S, Della Loggia R, Tubaro A, Simonovska B, Krasna A, et al. Topical anti-inflammatory activity of Salvia officinalis L. leaves: the relevance of ursolic acid. J Ethnopharmacol. 2001;75(2-3):125-32. [PubMed ID: 11297842].
-
29.
Tosun A, Khan S, Kim YS, Calin-Sanchez A, Hysenaj X, Carbonell-Barrachina A. Essential oil composition and anti-inflammatory activity of salvia officinalis l (lamiaceae) in murin macrophages. Tropical J Pharmaceutical Res. 2014;13(6):937-42.
-
30.
Schisterman EF, Gaskins AJ, Whitcomb BW. Effects of low-dose aspirin in in-vitro fertilization. Curr Opin Obstet Gynecol. 2009;21(3):275-8. [PubMed ID: 19300253]. https://doi.org/10.1097/GCO.0b013e32832a0673.
-
31.
Vardhana PA, Julius MA, Pollak SV, Lustbader EG, Trousdale RK, Lustbader JW. A unique human chorionic gonadotropin antagonist suppresses ovarian hyperstimulation syndrome in rats. Endocrinology. 2009;150(8):3807-14. [PubMed ID: 19443574]. https://doi.org/10.1210/en.2009-0107.
-
32.
Albert C, Garrido N, Mercader A, Rao CV, Remohi J, Simon C, et al. The role of endothelial cells in the pathogenesis of ovarian hyperstimulation syndrome. Mol Hum Reprod. 2002;8(5):409-18. [PubMed ID: 11994537].
-
33.
Villasante A, Pacheco A, Ruiz A, Pellicer A, Garcia-Velasco JA. Vascular endothelial cadherin regulates vascular permeability: Implications for ovarian hyperstimulation syndrome. J Clin Endocrinol Metab. 2007;92(1):314-21. [PubMed ID: 17032719]. https://doi.org/10.1210/jc.2006-1231.
-
34.
Halicioglu O, Astarcioglu G, Yaprak I, Aydinlioglu H. Toxicity of Salvia officinalis in a newborn and a child: an alarming report. Pediatr Neurol. 2011;45(4):259-60. [PubMed ID: 21907890]. https://doi.org/10.1016/j.pediatrneurol.2011.05.012.
-
35.
Hazzard TM, Molskness TA, Chaffin CL, Stouffer RL. Vascular endothelial growth factor (VEGF) and angiopoietin regulation by gonadotrophin and steroids in macaque granulosa cells during the peri-ovulatory interval. Mol Hum Reprod. 1999;5(12):1115-21. [PubMed ID: 10587365].
-
36.
Wu L, Zhang Z, Pan X, Wang Z. Expression and contribution of the HIF‑1α/VEGF signaling pathway to luteal development and function in pregnant rats. Mol Med Rep. 2015;12(5):7153-9.
-
37.
Berisha B, Schams D, Rodler D, Pfaffl MW. Angiogenesis in The Ovary - The Most Important Regulatory Event for Follicle and Corpus Luteum Development and Function in Cow - An Overview. Anat Histol Embryol. 2016;45(2):124-30. [PubMed ID: 25951313]. https://doi.org/10.1111/ahe.12180.