Abstract
Keywords
1. Background
Neurodegenerative disorders, Alzheimer, Parkinson, and type II diabetes mellitus, are caused by the formation and extracellular deposition of fibrillar amyloids for certain proteins (1-3). There are increasing reports indicating the involvement of biometals, e.g. zinc and copper, in this process (4-6). The fibrillation process consists of three distinct steps: nucleation, elongation, and saturation (or precipitation) of amyloid fibrils. Kinetic studies of protein fibrillation show that in the first step of fibrillation, a preliminary nucleus of the partially unfolded monomer is derived from the native protein (7). This step expected to be facilitated by high temperatures, high concentrations of protein, acidic pH, vigorous agitation, detergents, and organic solvents exposure (8). In the next elongation step of fibrillation, a protofibril structure is formed by the association of the nucleus. In the last step, protofibrils are assembled to form highly ordered and thermodynamically stable structures rich in beta sheets with least water solubility (9, 10). The precise mechanism of nucleation underlying the pathogenesis of amyloidogenic diseases remains to be characterized.
Insulin is a small protein with 51 residues that make a reliable model to simulate protein misfolding experimentally. In acidic pH, high temperature, agitation, and/or in presence of organic co-solvent, insulin forms fibrillar structures in amyloidogenic diseases (11).
Insulin is composed of two chains, A-chain with 21 residues and B-chain with 30 residues. These two chains are cross-linked by two interchain disulfide bonds (CysA7 - CysB7 and CysA20 - CysB19). There is also a third intrachain disulfide bond bridge made between CysA6 and CysA11. Insulin in its secondary structure contains about 50% alpha-helical structures (including segments of A1 - A8, A12 - A19, and B9 - B19) and about 10% beta structures (segment B24 - B28). Two beta turns (residues B6-B9 and B20-B23) comprise 16% of insulin structure and there is about 24% estimated random coil structure with about 15 residues (7-12).
Ethanol as an organic solvent diminishes hydrogen bonds between water and protein backbone that decreases protein stability and pushes it toward denaturation and misfolding (13).
There are some reports showing that low concentrations of ethanol exert a preservative effect on beta-amyloid protein and prevent aggregation. However, there is increasing evidence showing that high concentrations (> 80%) of ethanol trigger protein aggregation especially at acidic pH (14-17). Accordingly, ethanol at an 80% v/v concentration could be used to induce protein fibrillation in short time experimentally.
The zinc ion is an essential trace element prescribed supplementary in certain conditions, night blindness, cataracts, asthma, diabetes, high blood pressure, acquired immunodeficiency syndrome (AIDS), and skin conditions such as psoriasis, eczema, acne, and Alzheimer’s disease (18-24).
It was shown that zinc at millimolar concentrations chelates A-beta protein against aggregation. However, NMR experiments show that micromolar concentrations of zinc ions by decreasing the lag phase of fibrillation process accelerate fibrils formation (6, 25-29)
It is hypothesized that zinc ions have two sets of binding sites on the amyloid protein made by His and Asp residues, one site with high affinity and another with low affinity for zinc binding. These binding sites thought to be responsible for different effects of zinc on amyloidosis at different concentrations of zinc ions (30, 31).
In the case of insulin, the hexameric form, soluble in water and stable against fibrillation, is the dominant species in millimolar concentrations of zinc ions even at acidic pH of up to four (11, 32-37). Upon decreasing pH to two, this form is converted to the lower oligomers state of di and monomeric forms (38-41). At micromolar concentrations, zinc binds to high affinity binding sites on monomeric forms of insulin and pushes them toward molten globule formation, making them susceptible to unfolding and aggregation, while at high concentrations, zinc binds to low-affinity sites of hexameric forms and prevents their dissociation to monomers and blocks their aggregation (30, 31, 40, 41).
Based on miscellaneous reports and published documents, there is a clear inconsistency regarding beneficial or deleterious effects of zinc ions on amyloidogenic disease (20-26). The main purpose of the current work was to simulate insulin fibrillation at different concentrations of zinc ion to make a new look on this process, find some new evidence for this inconsistency, and add some useful comments hopefully.
2. Methods
Human insulin and thioflavin T were purchased from Sigma-Aldrich (USA). The rest of the chemicals used in this work were of the analytical grade. Insulin samples of 2 mg/mL were freshly prepared in an appropriate buffer prior to each experiment and its concentration was assessed at 276 nm with an extinction coefficient of 1.08 for 1.0 mg/mL.
The fibril formation was carried out in 25 mM glycine buffer (pH 2) by adding 80% v/v ethanol at 25°C temperature. Thioflavin T with a final concentration of 25 μM was used to detect fibril formation at Fluorescence emission spectra of 470-560 nm, excitation wavelength of 450nm, and scan-speed of 100 nm/min using a spectrofluorometer apparatus (JASCO Europe, Cremella, Italy, Great Dunmow, Essex, UK) with a maximum emission at 490 nm.
Circular Dichroism Measurements and spectral analysis were performed using AVIV (Lakewood, NJ) 60DS spectrophotometer and supplementary software within 250 to 190 nm range. The spectra were obtained at 25°C, 0.1 cm path length, the step size of 0.5 nm, the bandwidth of 1.5 nm, and protein concentration of 2 mg/mL in 50 mM glycine buffer pH 2.
Turbidity measurements were carried out at the 289 nm wavelength. To follow protein aggregation, a Biochrom WPA Bioware II UV/Visible spectrophotometer was used.
3. Results
In order to study the formation of insulin aggregates and its progression with time, we measured insulin turbidity at 289 nm wavelength, showing a linear behavior with turbidity. Based on previous reports, protein aggregation is a time-retarded phenomenon with a lag phase of about 5 - 10 hours that is accelerated at acidic pH, high temperatures, agitation, and co-solvent addition (8-10). Our study in accordance with previous reports indicated that a high concentration (80% v/v) of aliphatic alcohols, methanol, ethanol, and propanol, promoted insulin toward aggregation in such a way to reduce the lag phase of insulin aggregation to a couple of minutes instead of hours. Figure 1 shows the effect of methanol, ethanol, and propanol on protein turbidity at 289 nm with time. As observed, the increased R group from CH3 in methanol to C3H9 in propanol with more hydrophobic property could increase the insulin aggregation rate reasonably with a power order of propanol > ethanol > methanol. Among alcohols used, ethanol was chosen for our experiments as an aggregation inducer because human beings are naturally more vulnerable to ethanol exposure by subtle fermentations that take place in foods and beverages regularly.
The time course measurement of insulin turbidity at a 5 mg/mL concentration accelerated by 20% v/v concentration for aliphatic alcohols, methanol, ethanol, and propanol, in glycine buffer (50 mM and pH2) at 25°C.
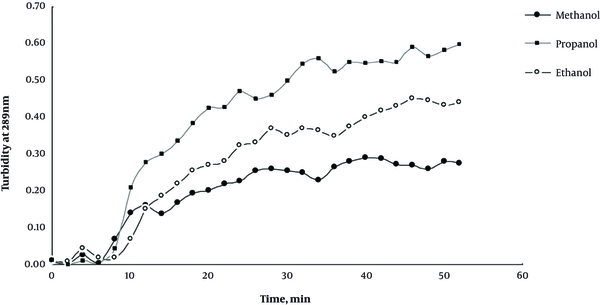
As mentioned earlier in the introduction section, there are serious inconsistencies regarding the zinc effect on protein aggregation. Does zinc induces protein aggregation or preserves it against this event when taken up as supplementary? To answer this important question, the samples of 0.2 mg/mL of insulin were first prepared in glycine buffer (50 mM, pH2) and then treated with 0, 0.05, 0.1, 1.0, and 5.0mM concentrations of zinc sulfate separately. Insulin aggregation then was triggered by adding ethanol at a final concentration of 80% v/v and the aggregation process was followed by scanning spectroscopic absorbance at 298 nm as the indicator of turbidity with time for up to 60 minutes. The results of these experiments are represented collectively in Figure 2. As shown, zinc ions at 0.05 and 0.1 mM (50 and 100°M) concentrations caused a more steep increase in absorbance and accelerated protein aggregations more efficiently when compared to the zero concentration of zinc. This is while at 1.0 and 5.0 mM concentrations, zinc ions represented a preservative effect on protein aggregation with lower absorbance compared to the zero concentration. These findings indicate that there should be a critical concentration of zinc effect in accelerating or inhibiting insulin aggregation. Based on this result, we decided to study the zinc effects on insulin aggregation at the micro (50 and 100 μM) and millimolar (1.0 and 5 mM) by different techniques to shed light on this dual effect of zinc on insulin aggregation and probably on amyloidogenic diseases when consumed clinically.
The aggregation phenomena of insulin at a 5 mg/mL concentration and 80% ethanol at different concentrations of zinc ions at 25°C in glycine buffer (50 mM and pH 2).
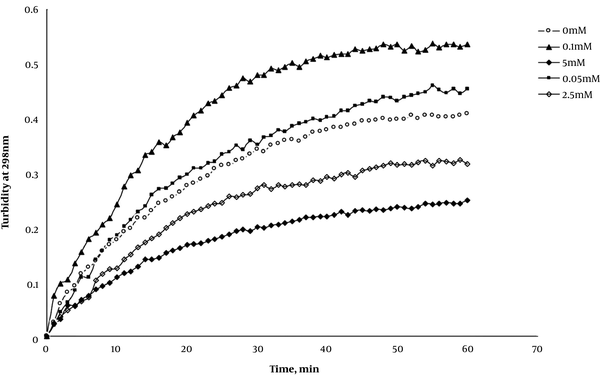
Thioflavin T is a fluorescent dye used routinely as an indicator chromophore to determine and quantify amyloid fibril formation during protein misfolding. This chromophore is hypothesized to intercalate within grooves in amyloid fibrils formed by solvent-exposed side chains with consequently increased fluorescence emission at 490 nm when excited at 440 nm with red-shifted characteristic peak. Figure 3 shows the fluorescence peaks of insulin, insulin + Zn (100 μM) + ethanol (80%) and insulin + Zn (5.0 mM) + ethanol (80%) samples within 450-600nm range. As it is obvious, insulin at micromolar concentrations of zinc ions (100 μM) and 80% ethanol shows a red-shifted peak with highly increased emission at 490 nm (~100%) that is characteristic for amyloid fibrils with increased beta structures.
ThT incorporation fluorescence spectra to human insulin in the presence and absence of 80% concentration of ethanol and 5 mM zinc ions incubated for 24 hours at 25°C.
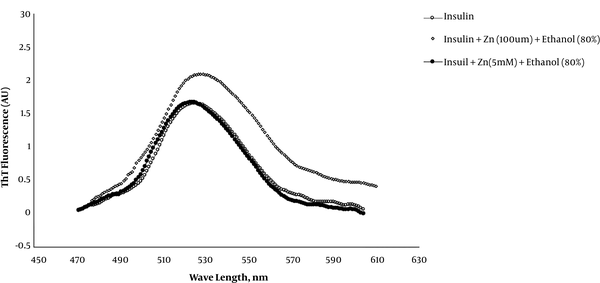
Nevertheless, this figure indicated that millimolar concentrations (5 mM) of zinc prevent insulin aggregation induced by 80% ethanol, with the least increase in the ThT emission (~6%) at 490 nm. This means that zinc exerts a preservative effect against insulin misfolding; this finding is consistent with our turbidity results.
It is well documented that protein aggregation is a process accompanied by an increase in beta structures at the expense of a decrease in helical structures. In order to follow and determine the secondary structure alteration in our samples, Far-UV CD spectroscopy was used in this context as a powerful method for this purpose. Figure 4 represents Far-UV CD spectra obtained for insulin, insulin + Zn (100 μM) + ethanol (80%) and insulin + Zn (5 mM) + ethanol (80%) in glycine buffer with 50mM concentration and pH 2 at 25°C. As observed, in the absence of ethanol and zinc ions, insulin spectrum contains two minima at 208 and 222 nm showing the prevalence of alpha-helical structure that is consistent with the native structure for insulin sample. In amyloidogenic conditions, these two minima disappeared gradually indicating alpha helix disruption followed by a peak appearance at 216 nm due to the beta structure formation.
Far-UV CD spectra of human insulin (2 mg/mL) in the presence and absence of 80% v/v ethanol and 5 mM concentration of zinc ions obtained at 25°C and incubated for one hour in glycine buffer (50 mM and pH 2).
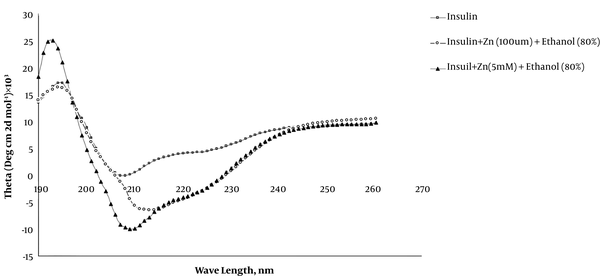
Detailed secondary structure alterations in our samples were followed by deconvolutions of CD spectra using CD Spectra deconvolution software. The results of insulin secondary structure analysis are summarized in Table 1. Our data indicate that in glycine buffer, pH 2, the amount of random coil structure was about 60% that is more than two-fold that for native insulin at pH 7 which contained about 24% of insulin residues. This finding conveys that in pH 2, such increment in insulin random coils makes insulin sensitive to misfolding by ethanol experimentally and makes insulin to become a suitable model for protein misfolding studies. Table 1 indicates that upon adding ethanol to the samples previously incubated with 100 μM zinc ions, the alpha structures decrease by about 7% at the expense of about 10% increase in the beta structure. This is while in the case of samples incubated with a 5 mM concentration of zinc ions, there is a negligible change in an alpha helix (1% increase) and beta structures (2% increase) at the expense of 3% decrease in the random coil structure. These findings confirm the protective effects of zinc ions at millimolar concentrations.
CD Values at 208 nm, 222 nm, and Secondary Structure Percentage for Insulin in Various Conditions
[θ]208 nm | [θ]222 nm | Helix, % | Beta, % | Random Coil, % | |
---|---|---|---|---|---|
Insulin | -0.058 | 4.21 | 12 | 23 | 65 |
Insulin + Zn (100 μM) + Ethanol (80%) | -2.556 | -3.85 | 5 | 32 | 63 |
Insuil + Zn (5 mM) + Ethanol (80%) | -9.72 | -3.52 | 13 | 25 | 62 |
4. Discussion
Undoubtedly, zinc as an essential trace element plays a critical role in biological processes by taking part in certain protein structures and helps to maintain their structural integrity and functionality. There are increasing reports advising zinc as medication in the management of old and newly emerged diseases (19-23). A dose-dependent or dual effect of the action is a well-known behavior in all drugs; it means each drug has an effective dose for its beneficial effect for treatment and a toxic dose for their unwanted side effects; thus, the latter is normally higher than the former.
Our findings in conjunction with previous reports indicated that the dual effect seen for zinc as supplementary medication is different so as to say that zinc at low concentrations (100 μM) exerts its side effects (misfolding induction), while at high concentrations (5 mM), zinc binds to its high-affinity binding sites and induces its therapeutic effects (misfolding inhibition) and protects proteins against aggregation (30, 31, 40, 41).
In this context, our ThT fluorescence experiments confirm that the 100 μM concentration of zinc ions caused ethanol (80%) to induce two fold aggregation with 100% increase in the ThT emission at 490 nm (Figure 3). This finding is interpreted as increased beta structure formation (32-42). When zinc concentration is increased to 5mM, the aggregation process seems to be inhibited completely because there is only 6% increase in the ThT emission, indicating the protective effect of zinc ions (Figure 3).
Our Far-UV CD experiments as a gold standard confirmed a significant increase in the beta structure occurred at low concentrations of zinc ions, with negligible changes at 5mM concentrations of zinc ions.
4.1. Conclusions
Unless neutral pH in which zinc preserves the natural hexameric form of insulin with native structure and function, in acidic pH or probably in destabilizing conditions, zinc affects the insulin structure integrity differently. Depending on its concentration, zinc at micromolar concentrations triggers and at millimolar concentrations inhibits insulin misfolding. Therefore, we can conclude that in normal cellular conditions, zinc seems to be a useful trace element, especially at millimolar concentrations. Due to effective absorption in the alimentary tract, ease of transportation, and successful targeting, zinc preparations at millimolar concentrations will guarantee the zinc beneficial effects in amyloidogenic organs.
References
-
1.
Huang X, Cuajungco MP, Atwood CS, Moir RD, Tanzi RE, Bush AI. Alzheimer's disease, beta-amyloid protein and zinc. J Nutr. 2000;130(5S Suppl):1488S-92S. [PubMed ID: 10801964].
-
2.
Liu R, Su R, Qi W, He Z. Photo-induced inhibition of insulin amyloid fibrillation on online laser measurement. Biochem Biophys Res Commun. 2011;409(2):229-34. [PubMed ID: 21570949]. https://doi.org/10.1016/j.bbrc.2011.04.132.
-
3.
Bekard IB, Dunstan DE. Tyrosine autofluorescence as a measure of bovine insulin fibrillation. Biophys J. 2009;97(9):2521-31. [PubMed ID: 19883595]. [PubMed Central ID: PMC2770625]. https://doi.org/10.1016/j.bpj.2009.07.064.
-
4.
Rezaei-Ghaleh N, Giller K, Becker S, Zweckstetter M. Effect of zinc binding on beta-amyloid structure and dynamics: implications for Abeta aggregation. Biophys J. 2011;101(5):1202-11. [PubMed ID: 21889458]. [PubMed Central ID: PMC3164126]. https://doi.org/10.1016/j.bpj.2011.06.062.
-
5.
Faller P, Hureau C. Bioinorganic chemistry of copper and zinc ions coordinated to amyloid-beta peptide. Dalton Trans. 2009;(7):1080-94. [PubMed ID: 19322475]. https://doi.org/10.1039/b813398k.
-
6.
Hane F, Leonenko Z. Effect of metals on kinetic pathways of amyloid-beta aggregation. Biomolecules. 2014;4(1):101-16. [PubMed ID: 24970207]. [PubMed Central ID: PMC4030978]. https://doi.org/10.3390/biom4010101.
-
7.
Tougu V, Tiiman A, Palumaa P. Interactions of Zn(II) and Cu(II) ions with Alzheimer's amyloid-beta peptide. Metal ion binding, contribution to fibrillization and toxicity. Metallomics. 2011;3(3):250-61. [PubMed ID: 21359283]. https://doi.org/10.1039/c0mt00073f.
-
8.
Hua QX, Weiss MA. Mechanism of insulin fibrillation: the structure of insulin under amyloidogenic conditions resembles a protein-folding intermediate. J Biol Chem. 2004;279(20):21449-60. [PubMed ID: 14988398]. https://doi.org/10.1074/jbc.M314141200.
-
9.
Bouchard M, Zurdo J, Nettleton EJ, Dobson CM, Robinson CV. Formation of insulin amyloid fibrils followed by FTIR simultaneously with CD and electron microscopy. Protein Sci. 2000;9(10):1960-7. [PubMed ID: 11106169]. [PubMed Central ID: PMC2144465]. https://doi.org/10.1110/ps.9.10.1960.
-
10.
Whittingham JL, Scott DJ, Chance K, Wilson A, Finch J, Brange J, et al. Insulin at pH 2: structural analysis of the conditions promoting insulin fibre formation. J Mol Biol. 2002;318(2):479-90. [PubMed ID: 12051853]. https://doi.org/10.1016/S0022-2836(02)00021-9.
-
11.
Nettleton EJ, Tito P, Sunde M, Bouchard M, Dobson CM, Robinson CV. Characterization of the oligomeric states of insulin in self-assembly and amyloid fibril formation by mass spectrometry. Biophys J. 2000;79(2):1053-65. [PubMed ID: 10920035]. [PubMed Central ID: PMC1301001]. https://doi.org/10.1016/S0006-3495(00)76359-4.
-
12.
Dong J, Wan Z, Popov M, Carey PR, Weiss MA. Insulin assembly damps conformational fluctuations: Raman analysis of amide I linewidths in native states and fibrils. J Mol Biol. 2003;330(2):431-42. [PubMed ID: 12823980].
-
13.
Ahmad A, Millett IS, Doniach S, Uversky VN, Fink AL. Partially folded intermediates in insulin fibrillation. Biochemistry. 2003;42(39):11404-16. [PubMed ID: 14516191]. https://doi.org/10.1021/bi034868o.
-
14.
Parodi J, Ormeno D, Ochoa-de la Paz LD. Amyloid pore-channel hypothesis: effect of ethanol on aggregation state using frog oocytes for an Alzheimer's disease study. BMB Rep. 2015;48(1):13-8. [PubMed ID: 25047445]. [PubMed Central ID: PMC4345636].
-
15.
Peters R, Peters J, Warner J, Beckett N, Bulpitt C. Alcohol, dementia and cognitive decline in the elderly: a systematic review. Age Ageing. 2008;37(5):505-12. [PubMed ID: 18487267]. https://doi.org/10.1093/ageing/afn095.
-
16.
Orgogozo JM, Dartigues JF, Lafont S, Letenneur L, Commenges D, Salamon R, et al. Wine consumption and dementia in the elderly: a prospective community study in the Bordeaux area. Rev Neurol (Paris). 1997;153(3):185-92. [PubMed ID: 9296132].
-
17.
Goda S, Takano K, Yamagata Y, Nagata R, Akutsu H, Maki S, et al. Amyloid protofilament formation of hen egg lysozyme in highly concentrated ethanol solution. Protein Sci. 2000;9(2):369-75. [PubMed ID: 10716189]. [PubMed Central ID: PMC2144556]. https://doi.org/10.1110/ps.9.2.369.
-
18.
Prasad AS. Discovery of human zinc deficiency: 50 years later. J Trace Elem Med Biol. 2012;26(2-3):66-9. [PubMed ID: 22664333]. https://doi.org/10.1016/j.jtemb.2012.04.004.
-
19.
Walker BR, Colledge NR. Davidson's Principles and Practice of Medicine E-Book. Elsevier Health Sciences; 2013.
-
20.
Gurvits GE, Tan A. Burning mouth syndrome. World J Gastroenterol. 2013;19(5):665-72. [PubMed ID: 23429751]. [PubMed Central ID: PMC3574592]. https://doi.org/10.3748/wjg.v19.i5.665.
-
21.
Foster M, Samman S. Zinc and regulation of inflammatory cytokines: implications for cardiometabolic disease. Nutrients. 2012;4(7):676-94. [PubMed ID: 22852057]. [PubMed Central ID: PMC3407988]. https://doi.org/10.3390/nu4070676.
-
22.
Suzuki H, Asakawa A, Li JB, Tsai M, Amitani H, Ohinata K, et al. Zinc as an appetite stimulator - the possible role of zinc in the progression of diseases such as cachexia and sarcopenia. Recent Pat Food Nutr Agric. 2011;3(3):226-31. [PubMed ID: 21846317].
-
23.
Chasapis CT, Loutsidou AC, Spiliopoulou CA, Stefanidou ME. Zinc and human health: an update. Arch Toxicol. 2012;86(4):521-34. [PubMed ID: 22071549]. https://doi.org/10.1007/s00204-011-0775-1.
-
24.
Millichap JG, Yee MM. The diet factor in attention-deficit/hyperactivity disorder. Pediatrics. 2012;129(2):330-7. [PubMed ID: 22232312]. https://doi.org/10.1542/peds.2011-2199.
-
25.
Bush AI, Tanzi RE. Therapeutics for Alzheimer's disease based on the metal hypothesis. Neurotherapeutics. 2008;5(3):421-32. [PubMed ID: 18625454]. [PubMed Central ID: PMC2518205]. https://doi.org/10.1016/j.nurt.2008.05.001.
-
26.
Bonda DJ, Lee HG, Blair JA, Zhu X, Perry G, Smith MA. Role of metal dyshomeostasis in Alzheimer's disease. Metallomics. 2011;3(3):267-70. [PubMed ID: 21298161]. [PubMed Central ID: PMC3117398]. https://doi.org/10.1039/c0mt00074d.
-
27.
Ha C, Ryu J, Park CB. Metal ions differentially influence the aggregation and deposition of Alzheimer's beta-amyloid on a solid template. Biochemistry. 2007;46(20):6118-25. [PubMed ID: 17455909]. https://doi.org/10.1021/bi7000032.
-
28.
Pedersen JT, Ostergaard J, Rozlosnik N, Gammelgaard B, Heegaard NH. Cu(II) mediates kinetically distinct, non-amyloidogenic aggregation of amyloid-beta peptides. J Biol Chem. 2011;286(30):26952-63. [PubMed ID: 21642429]. [PubMed Central ID: PMC3143654]. https://doi.org/10.1074/jbc.M111.220863.
-
29.
Sarell CJ, Wilkinson SR, Viles JH. Substoichiometric levels of Cu2+ ions accelerate the kinetics of fiber formation and promote cell toxicity of amyloid-{beta} from Alzheimer disease. J Biol Chem. 2010;285(53):41533-40. [PubMed ID: 20974842]. [PubMed Central ID: PMC3009880]. https://doi.org/10.1074/jbc.M110.171355.
-
30.
Danielsson J, Pierattelli R, Banci L, Graslund A. High-resolution NMR studies of the zinc-binding site of the Alzheimer's amyloid beta-peptide. FEBS J. 2007;274(1):46-59. [PubMed ID: 17222176]. https://doi.org/10.1111/j.1742-4658.2006.05563.x.
-
31.
Cardoso SM, Rego AC, Pereira C, Oliveira CR. Protective effect of zinc on amyloid-beta 25-35 and 1-40 mediated toxicity. Neurotox Res. 2005;7(4):273-81. [PubMed ID: 16179264].
-
32.
Brange J, Owens DR, Kang S, Volund A. Monomeric insulins and their experimental and clinical implications. Diabetes Care. 1990;13(9):923-54. [PubMed ID: 2226110].
-
33.
Baker EN, Blundell TL, Cutfield JF, Cutfield SM, Dodson EJ, Dodson GG, et al. The structure of 2Zn pig insulin crystals at 1.5 A resolution. Philos Trans R Soc Lond B Biol Sci. 1988;319(1195):369-456. [PubMed ID: 2905485].
-
34.
De Meyts P. Insulin and its receptor: structure, function and evolution. Bioessays. 2004;26(12):1351-62. [PubMed ID: 15551269]. https://doi.org/10.1002/bies.20151.
-
35.
Attri AK, Fernandez C, Minton AP. pH-dependent self-association of zinc-free insulin characterized by concentration-gradient static light scattering. Biophys Chem. 2010;148(1-3):28-33. [PubMed ID: 20202737]. [PubMed Central ID: PMC2856799]. https://doi.org/10.1016/j.bpc.2010.02.002.
-
36.
Dunn MF. Zinc-ligand interactions modulate assembly and stability of the insulin hexamer -- a review. Biometals. 2005;18(4):295-303. [PubMed ID: 16158220]. https://doi.org/10.1007/s10534-005-3685-y.
-
37.
Correia M, Neves-Petersen MT, Jeppesen PB, Gregersen S, Petersen SB. UV-light exposure of insulin: pharmaceutical implications upon covalent insulin dityrosine dimerization and disulphide bond photolysis. PLoS One. 2012;7(12). e50733. [PubMed ID: 23227203]. [PubMed Central ID: PMC3515625]. https://doi.org/10.1371/journal.pone.0050733.
-
38.
Nedumpully-Govindan P, Yang Y, Andorfer R, Cao W, Ding F. Promotion or Inhibition of Islet Amyloid Polypeptide Aggregation by Zinc Coordination Depends on Its Relative Concentration. Biochemistry. 2015;54(50):7335-44. [PubMed ID: 26603575]. https://doi.org/10.1021/acs.biochem.5b00891.
-
39.
Nicolson TJ, Bellomo EA, Wijesekara N, Loder MK, Baldwin JM, Gyulkhandanyan AV, et al. Insulin storage and glucose homeostasis in mice null for the granule zinc transporter ZnT8 and studies of the type 2 diabetes-associated variants. Diabetes. 2009;58(9):2070-83. [PubMed ID: 19542200]. [PubMed Central ID: PMC2731533]. https://doi.org/10.2337/db09-0551.
-
40.
Brender JR, Hartman K, Nanga RP, Popovych N, de la Salud Bea R, Vivekanandan S, et al. Role of zinc in human islet amyloid polypeptide aggregation. J Am Chem Soc. 2010;132(26):8973-83. [PubMed ID: 20536124]. [PubMed Central ID: PMC2904811]. https://doi.org/10.1021/ja1007867.
-
41.
Salamekh S, Brender JR, Hyung SJ, Nanga RP, Vivekanandan S, Ruotolo BT, et al. A two-site mechanism for the inhibition of IAPP amyloidogenesis by zinc. J Mol Biol. 2011;410(2):294-306. [PubMed ID: 21616080]. [PubMed Central ID: PMC3115507]. https://doi.org/10.1016/j.jmb.2011.05.015.
-
42.
Tougu V, Karafin A, Zovo K, Chung RS, Howells C, West AK, et al. Zn(II)- and Cu(II)-induced non-fibrillar aggregates of amyloid-beta (1-42) peptide are transformed to amyloid fibrils, both spontaneously and under the influence of metal chelators. J Neurochem. 2009;110(6):1784-95. [PubMed ID: 19619132]. https://doi.org/10.1111/j.1471-4159.2009.06269.x.