Abstract
Keywords
Noise Pollution Aluminum Foam Gas Power Plant Sound Absorption Coefficient
1. Background
The noise pollution analysis of power plants shows that this pollution can cause serious concern for the workforce in these locations (1, 2). The noise pollution problem is not specific to developing countries; hence, it is also a major difficulty for the developed countries (3). Nowadays, further attention is paid to the accidents caused by noise pollution more than ever because of the issues such as the need for environments which are a more suitable and more desirable lifestyle. In the industrial sector, there are various sound pollution sources, specifically power plants which are the main centers of power generation (4). Electricity plays a vital role in the industrial and social development in developing countries. In addition to their valuable work, these centers have environmental problems including noise pollution (5). According to the National Institute for Occupational Safety and Health (NIOSH), the determination of main noise sources in a thermal power plant is of great importance (6). Thus, various materials including soft polymer foams, mineral fibers, and glass wool, were found to turn the sound waves into heat while hitting the fibers and the inter-filament air so that the sound is absorbed. However, the environmental hazards and harmful effects of these substances on health cannot be ignored (7). Therefore, the researchers seek to find materials with lower pollution, weight, and cost that can absorb sound waves at high frequencies (8). Metal foams, as one of the solutions proposed in this area (9), have sound absorption characteristics which are a good option to control noise pollution, especially in the industrial environments. Porosity, size, thickness, airflow resistance, pore morphology, closed/open pores, angle of impact of the acoustic waves and resistance of the airflow are important factors influencing sound absorption coefficient in metal foams (10). The simplest method used for making the foams is producing them directly by injecting the gas bubbles into the molten metal. By this method, the large parts are produced at a relatively low cost (11). Wang et al. (9) showed that the acoustic absorption coefficient of aluminum foam was equal to 0.4 at low frequencies. They found that the sound absorption coefficient was higher at higher frequencies. Besides, the aluminum foam with a pore size of 500 µm had the highest sound absorption coefficient.
Tao et al. (10) and Stanev et al. (11) synthesized the aluminum foam using solid-state production methods and showed that these foams are one of the most widely used foams at high melting temperatures. Previous studies showed that porous metals have reasonable effects on sound absorption due to the morphology of free pores with improved functional properties and mainly due to high resistance to mechanical and chemical materials (12). In a study using nickel foam showed that a 7.5 mm thick foam sample formed by a 5-layer foam panel (thickness: 1.5 mm; porosity: 96%; average diameter: 0.65 mm) could produce an excellent sound absorption effect at 4000 Hz with an absorption coefficient of about 0.8 (13). Studies showed that using the aluminum foam to reduce noise pollution could play a significant role in the power industry. On the other hand, using the aluminum foam to reduce the noise pollution should be evaluated due to the high resistance of aluminum foam to humidity and heat, high rigidity at a low weight, and energy absorption. Despite the studies on metal foam effect and its varieties on sound absorption, few studies were done on the effect of these types of foam on industrial environments and especially thermal power plants.
2. Objectives
Therefore, this study aims to synthesize the aluminum foam with nano surface topography and its application to reduce the noise pollution in a gas power plant under construction.
3. Methods
At first aluminum, the foam was made from the aluminum powder with a pore size of 300 – 500 µm and a porosity of 90%. Then, to predict the noise pollution in the Tarasht gas power plant (a power plant under construction (We measured noise pollution at a similar power plant in terms of the type of fuel, electricity production, and technical specifications (Figure 1), and also we used from the simulator software (ANSYS software model 2019 R3).
Measurement stations of similar gas power plant
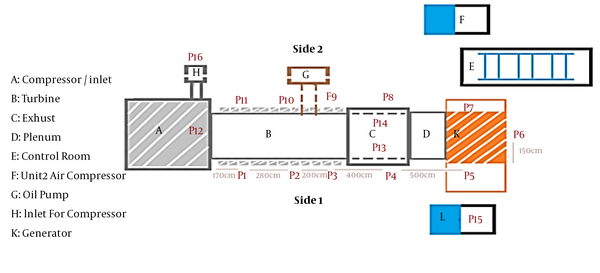
3.1. Synthesis of Aluminum Foam
For aluminum foam synthesis, the aluminum smelting process was carried out at a temperature range from 670°C - 690°C. Then, about 1.5 wt% of calcium was added to the aluminum melt at 680°C and mixed using a mixer at 900 rpm to concentrate the liquid metal. After the viscosity reached the desired level, 1% of TiH2 was added to the molten solution. TiH2 is the most common foam maker. It decomposes at 470°C and releases H2 gas, resulting in the formation of a large volume of bubbles, as well as the foam with closed cells. When the foam was formed, the chamber was cooled down below the aluminum melting point (Figure 2).
Manufacturing process of aluminum foam
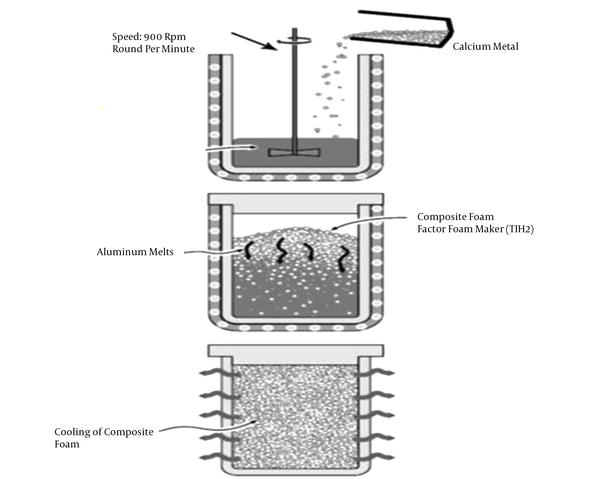
3.2. Determination of Sound Absorption Coefficient in Aluminum Foam
After aluminum foam synthesis, the acoustic absorption coefficient of aluminum foam was measured by the B & K model 4206 impedance tube (B & K, Denmark), equipped with two microphones with tube diameters of 30 and 100 mm. In this method, a speaker was used to create standing waves inside a tube, where at its backend, there was a specimen of the absorbent material. Each specimen was separately inserted into the tube, and the absorption coefficient was calculated by measuring the ratio between the maximum and minimum acoustic pressures by a cylindrical microphone moving along the tube. This method complies with the C384 standard introduced by the American Society for Testing and Materials (ASTM) (Figure 3).
Schematic diagram of impedance tube
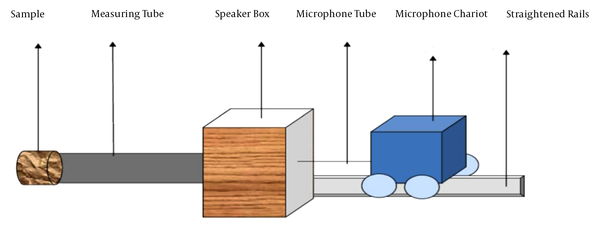
3.3. Predict of Noise Pollution at a Gas Power Plant Under Construction
Considering the plant was under construction and was not fully operational yet, the manufacturer’s data were used by measuring the noise level of a similar power plant and the sound absorption coefficient of aluminum foam in the ANSYS Products V. 2019R3 to acoustically analyze the aluminum foam exhaust duct in a simulated environment.
4. Results and Discussion
According to the measurements of noise pollution areas in the similar power plant, the highest noise level in the compressor and exhaust sections was equal to 93 dB (Figure 4).
A view of exhaust from gas power plant
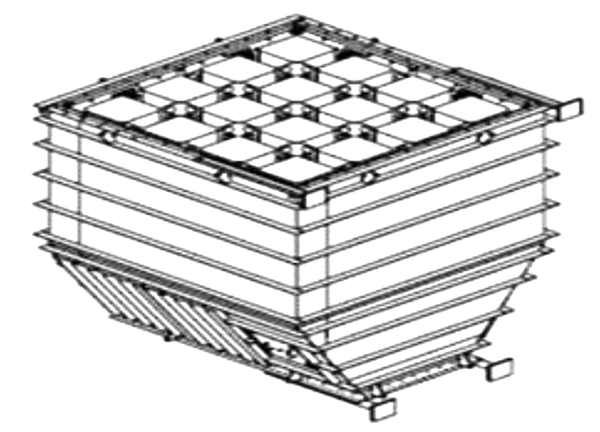
After aluminum foam synthesis, the sound absorption coefficient was determined for the foam produced in the laboratory. Figure 5 shows the results obtained from the measurement of sound absorption coefficient at different frequencies on the aluminum foam specimens. The highest sound absorption coefficient in the aluminum foam with a thickness of 1cm was equal to 0.777 and 0.840 at the frequencies of 1600 and 6300 Hz, respectively.
Sound absorption coefficient of aluminum foam
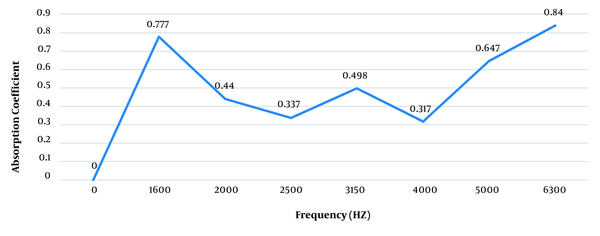
The aluminum foam was used in the simulation environment to reduce the noise pollution. The analysis results using the ANSYS software for the walls with or without aluminum foam indicated that SPL distribution reached 74 dB at the frequencies of 1600 and 60 dB at the frequency of 6300 Hz in the wall with aluminum foam located at a 1.5 m distance from the ground level and 1m from the equipment (standard ISO 3741). Meanwhile, SPL distribution reached 77 dB at the frequencies of 1600 and 68 dB at the frequency of 6300 Hz on the wall without aluminum foam (Figure 6).
Distribution of SPL in a wall with or without aluminum foam at the frequencies of 1600 - 6300 Hz
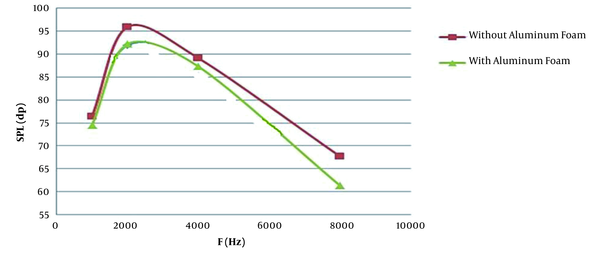
The model designed to predict the sound pressure level (before and after the installation of aluminum foam) was assessed at a frequency of 1000 Hz. It can be concluded that the minimum and maximum SPL in the wall without aluminum foam were equal to 24 dB and 99 dB, respectively (Figure 7).
Distribution of SPL in the wall without aluminum foam at the frequency of 1000 Hz
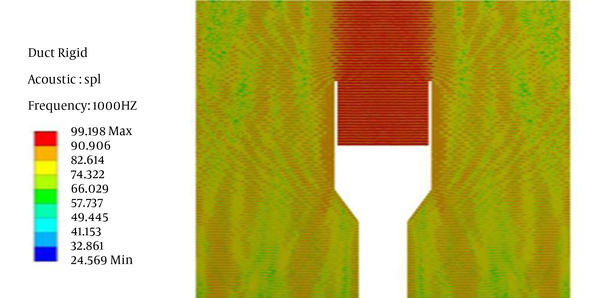
In the model of aluminum foam wall, sound pressure level distribution reached at -30 dB (minimum) to 99 dB (maximum) at the frequency of 1000 Hz (Figure 8).
Distribution of SPL in a multilayer wall environment at the frequency of 1000 Hz
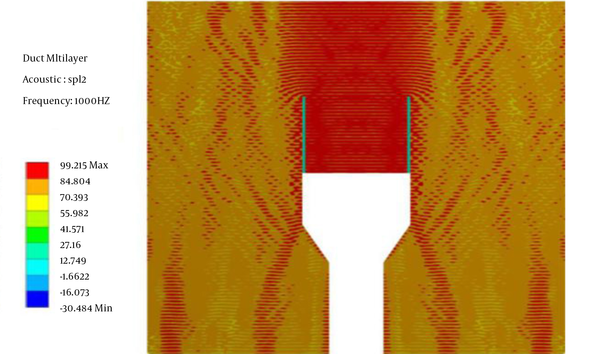
The highest noise pollution areas (turbine exhaust and air compressor) were predicted in the mentioned power plant using the noise pressure level at a similar power plant (Figure 9). The equivalent level was equal to 93 dB which was high compared to the permissible acoustic comfort level. In Iran, the standard range of industrial environments is equal to 70 dB, as recommended by the National Occupational Health Committee (14). A study on the noise pollution reduction in all power plant equipment showed that the highest noise level could be attributed to the exhaust. Hence, they applied a silencer to reduce exhaust noise (15). Therefore, it seems that the exhaust is the most important source of noise, confirming our results regarding the noise pressure level in the power plant.
Results of SPL measurements at all locations around turbine of the similar power plant at the frequencies of 20 - 8000 Hz
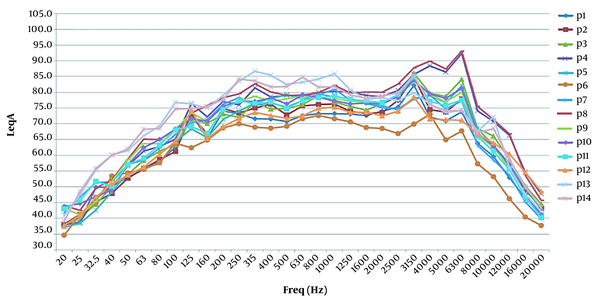
The studies showed that exhaust, booster pumps, fans, and compressors are the most important noise sources in the power plant, which, in some cases, the noise was reported to exceed recommended limits (16). The noise generation in power plant equipment is associated to a number of factors. From the industrial point of view, these factors include the mechanical and structural nature of device, the degradation of mechanical components, malfunctioning of the machines’ moving parts, and weak foundations (17, 18). After specifying noise pollution areas and synthesizing the aluminum foam, the sound absorption coefficient was measured in these areas. The results stated that the aluminum foam could be a good adsorbent in the power plant. Considering the high demand for aluminum foams, there is a need in the industries to develop the methods to measure the aluminum foams with a low cost and high quality (19). Tao et al. (10), using solid-state production methods (using powder technology, gas-producing materials such as TiH2, and spacers such as NaCl, together with the metal powder), showed that the aluminum foams with high melting temperature can be good acoustic absorbers to reduce noise pollution. In another study, Stanev et al. (11) showed that aluminum foam is suitable for the acoustic absorption. Wang et al. (9), found that with higher frequencies, the absorption coefficient of aluminum foam increases. In another study, Navacerrada et al. (20) identified aluminum foam with a pore diameter of 500 µm as the best sound absorbent. In a study entitled “The investigation of the effect of aluminum alloy foam holes on sound absorption coefficient”, the sound absorption behavior of aluminum foams was studied. The sound absorption behavior of aluminum alloy foams with pores less than 250 microns was much weaker at low frequencies than alloy foams with pore sizes in the range of 1000 microns. While, emphasizing the patterns of aluminum alloy foam on the sound absorption coefficient, the cavity and its size on the sound absorption were studied (21). In previous studies, compared to a study conducted at the Tarasht power plant, all confirmed that compressors and chimneys have the highest noise pollution in the gas plant, and closed-cell aluminum foam with a pore size of 500 microns has the greatest impact on reducing noise pollution. Finally, similar to previous studies, it was found that the aluminum foam could be a suitable adsorbent for noise pollution reduction in power plants.
4.1. Conclusions
Results related to this study revealed that the aluminum foam could reduce sound pressure levels in the duct wall of chimney output. If 10 cm thick insulation layer includes reticulated stainless steel plates, refractory fabric, and closed-cell aluminum foam (thickness of 1cm and porosity of 90%); then, we will have at least an 8 dB reduction in the noise pollution. As aluminum foam is much more resistant than rock wool and has a higher strength against thermal stress and less environmental pollution; thus it can be a very good alternative to rock wool.
Acknowledgements
References
-
1.
Eluozo SN, Onwuka GM, Nwaobakata C. Noise pollution effect from generating power plant and electronics device on human and the environment a case of port harcourt. World J Civil Mech Electr Eng. 2014:1-5.
-
2.
Aliabadi M, Golmohammadi R, Khotanlou H, Mansoorizadeh M, Salarpour A. Development of a noise prediction model based on advanced fuzzy approaches in typical industrial workrooms. J Res Health Sci. 2014;14(2):157-62. [PubMed ID: 24728753].
-
3.
Saeki T, Fujii T, Yamaguchi S, Harima S. Effects of acoustical noise on annoyance, performance and fatigue during mental memory task. Appl Acoust. 2004;65(9):913-21. https://doi.org/10.1016/j.apacoust.2003.12.005.
-
4.
Golmohammadi R, Aliabadi M, Motlagh MS, Goodarzi R. Efficiency assessment of acoustic cabin for providing acoustic comfort in turbine unit of a thermal power plant. J Occup Hyg Eng. 2019;6(1):1-7.
-
5.
Byakova A, Gnyloskurenko S, Bezimyanniy Y, Nakamura T. Closed-Cell Aluminum Foam of Improved Sound Absorption Ability: Manufacture and Properties. Metals. 2014;4(3):445-54. https://doi.org/10.3390/met4030445.
-
6.
Banhart J. Manufacture, characterisation and application of cellular metals and metal foams. Progress Mater Sci. 2001;46(6):559-632. https://doi.org/10.1016/s0079-6425(00)00002-5.
-
7.
Guan D, Wu JH, Wu J, Li J, Zhao W. Acoustic performance of aluminum foams with semiopen cells. Appl Acoust. 2015;87:103-8. https://doi.org/10.1016/j.apacoust.2014.06.016.
-
8.
Alhusseny ANM, Nasser AG, Al-zurf NM. High-Porosity Metal Foams: Potentials, Applications, and Formulations. Porosity-Process, Technologies and Applications. 2018. p. 181-200. https://doi.org/10.5772/intechopen.70451.
-
9.
Wang F, Wang LC, Wu JG, You XH. Sound absorption property of open-pore aluminum foams. China Foundry Res Dev. 2007;4:31-3.
-
10.
Tao YB, You Y, He YL. Lattice Boltzmann simulation on phase change heat transfer in metal foams/paraffin composite phase change material. Appl Therm Eng. 2016;93:476-85. https://doi.org/10.1016/j.applthermaleng.2015.10.016.
-
11.
Stanev L, Kolev M, Drenchev B, Drenchev L. Open-Cell Metallic Porous Materials Obtained Through Space Holders-Part II: Structure and Properties. A Review. J Manuf Sci Eng. 2017;139(5). https://doi.org/10.1115/1.4034440.
-
12.
Erdeniz D, Schaedler TA, Dunand DC. Deposition-based synthesis of nickel-based superalloy microlattices. Scripta Mat. 2017;138:28-31. https://doi.org/10.1016/j.scriptamat.2017.05.027.
-
13.
Liu P, Xu X, Cheng W, Chen J. Sound absorption of several various nickel foam multilayer structures at aural frequencies sensitive for human ears. Trans Nonferrous Met Soc China. 2018;28(7):1334-41. https://doi.org/10.1016/s1003-6326(18)64771-5.
-
14.
Education IMoHAM. Occupational exposure limits (OEL). Tehran: Environmental and Occupational Health Center; 2016.
-
15.
Tupov VB. A package of measures to reduce the noise from thermal power plants. Power Technol Eng. 2013;47(3):217-21. https://doi.org/10.1007/s10749-013-0426-1.
-
16.
Kisku GC, Bhargava SK. Assessment of noise level of a medium scale thermal power plant. Indian J Occup Environ Med. 2006;10(3). https://doi.org/10.4103/0019-5278.29575.
-
17.
Barron RF. Industrial Noise Control and Acoustics. 2002. p. 43-6. https://doi.org/10.1201/9780203910085.
-
18.
R G. Noise & Vibration engineering. 4th ed. Hamadan: Daneshjo; 2010.
-
19.
Rakow JF, Waas AM. Size effects and the shear response of aluminum foam. Mech Mat. 2005;37(1):69-82. https://doi.org/10.1016/j.mechmat.2003.12.002.
-
20.
Navacerrada MA, Fernández P, Díaz C, Pedrero A. Thermal and acoustic properties of aluminium foams manufactured by the infiltration process. Appl Acoust. 2013;74(4):496-501. https://doi.org/10.1016/j.apacoust.2012.10.006.
-
21.
Bahreini A, Alizadeh A, Baharvandi HR, Ramezani Ghaemi A. Investigation of the effect of aluminum alloy foam pore size on the sound absorption coefficient. J Acoust Eng Soc Iran. 2015;2(2):34-40.