Abstract
Context:
Today, the growth of excessive energy consumption and concerns about the conservation of natural resources has led to the identification and exploitation of new energy sources. One of the new energy sources is the production of energy from biomass, where sludge forms a significant source of biomass.Objectives:
This study aims to review novel methods of sludge processing, such as dark fermentation, photofermentation, anaerobic digestion, and bioelectrochemical processes, in addition to their advantages and disadvantages.Data Sources:
A literature search was conducted on PubMed, ScienceDirect, and Web of Science databases between 2000 - 2022 to find related articles. A total of 315 records were identified, of which 80 studies were retained that met the review’s inclusion criteria. Search engines were searched using some keywords, including biohydrogen, sludge processing, dark fermentation, photofermentation, bioelectrochemical system, microbial fuel cell, anaerobic digestion, microbial electrolysis cell, bioplastic, and polyhydroxyalkanoates.Results:
The reviewed studies have shown that dark fermentation alone cannot produce high levels of biohydrogen. However, the level of biohydrogen production increases significantly when dark fermentation is coupled with anaerobic digestion, optical fermentation, or bioelectrochemical processes. The advantages of integrating dark fermentation with other processes can be mentioned as an increase in biohydrogen production, the use of dark fermentation end products in electricity generation by bioelectrochemical systems, and bioplastics production.Conclusions:
With the development of biohydrogen production methods from renewable energy sources, dependence on fossil fuel systems will be reduced in the future. A review of various studies showed that combining the dark fermentation process with other processes also has disadvantages. Therefore, biohydrogen production strategies at an appropriate economic level need to be developed, and further research is required.Keywords
Sludge Processing Anaerobic Digestion Dark Fermentation Photofermentation Bioelectrochemical Biohydrogen Bioplastic
1. Context
Sewage sludge is a by-product of produced wastewater treatment worldwide. Due to its large production and disposal, it has recently become a subject of interest. However, there are numerous types of modern sewage sludge treatment methods. Nevertheless, with the low technology development, high investiture, and insufficient relevant laws, practical exploitation is still unsatisfactory and limited (1, 2).
Hydrogen is clean energy manufactured from renewable sources and fossil fuels, such as biomass, solar energy, and wind. To eliminate environmental pollution and its relationship with fossil fuels, renewable sources, according to the technologies of hydrogen production, have been noticed worldwide. Hydrogen produced from sewage sludge is known as one of these sources (3, 4). Hydrogen production by biological technology mostly mentions light and dark fermentation. In these methods, organic materials change to carbon dioxide, biohydrogen, and other products with the microorganisms’ action in various conditions (5, 6).
Hydrogen production by the dark fermentation method has attracted great attention because it can use cheaper and more abundant raw materials, which reduces production costs, and its practical application is more feasible (7-9). The maximum theoretical hydrogen yield using dark fermentation is only 4 mol H2/mol glucose. However, the energy yield is less than 3 mol H2/mol glucose in practical operation (10, 11). Among the disadvantages of traditional hydrogen production methods, such as the gasification of fossil fuels and water electrolysis, there is the need for high energy, cost, and instability (12-14).
The main gaseous fermentation products include hydrogen and carbon dioxide, and the main liquid products are ethanol or organic acids (most often acetic, formic, lactic, or butyric) (15). Studies show that to increase the profit of dark fermentation, parallel research on post-fermentation broth management should be carried out (15-17).
Photofermentation is the fermentative decomposition of organic substrates directly into hydrogen by various photosynthetic microorganisms, including purple and green anaerobic bacteria. Hydrogen produced in photofermentation is relatively pure (containing 20% carbon dioxide), which eliminates the need for energy and time purification process on the obtained gas. Under light conditions, purple non-sulfur bacteria, including Rhodobacter species, use organic acids, such as acetate, lactate, and butyrate, for this purpose. Moreover, purple sulfur bacteria use reduced sulfur compounds and are the product of the molecular hydrogen process (18, 19).
The concept of anaerobic digestion was introduced around 1870 through the development of the septic tank system (20). Anaerobic digestion technology is used not only for organic waste treatment but also for wastewater treatment (21). Anaerobic digestion is a biological process that breaks down complex organic matter into simpler chemical components in the absence of oxygen. During this process, the final products are in ideal biogas conditions, which mainly contain CH4 and CO2. A small amount of hydrogen sulfide (H2S), ammonia (NH3), and other gases are also formed during biogas production in the anaerobic digestion plant (22).
Microorganisms play an essential role in the anaerobic digestion process, and bacterial groups differ among the stages of hydrolysis, acidification, and methane production (23). There are countless benefits associated with the anaerobic digestion process, such as digestion for agricultural applications, reduction of greenhouse gas emissions, and production of high-quality renewable fuel (24). However, disadvantages of the anaerobic digestion process, such as long holding time, required control of some important parameters (e.g., pH, temperature, feed rate, and alkalinity), and relatively high capital costs, prevent its widespread implementation (24).
In addition, bioelectrochemical systems are used separately or in combination with other systems to produce electricity and hydrogen from sewage sludge (25, 26). With the increase in population and the advancement of technology, the consumption of all kinds of plastic has increased significantly. In recent years, public concern about the harmful effects of synthetic plastics on the environment has increased, and this issue has made researchers in various countries strive to develop biodegradable plastics. Non-degradable plastic pollution can be solved by producing environmentally friendly plastics, such as bioplastics (27).
2. Objectives
This study aims to review the new methods of sludge processing, for example, dark and light fermentation and bioelectrochemical processes, for energy production and bioplastics, and their advantages and disadvantages. Finally, the study gap in this field is determined, and the direction of future studies for sludge processing is stated.
3. Methods
First, the inclusion and exclusion criteria of the present study were determined. The inclusion criteria included only English articles published in the field of sludge processing, and articles published in other languages were excluded from the study. Books, presentations, theses, articles presented in conferences, seminars, and letters to the editor were also excluded from the study.
Then, according to the research title, suitable keywords were selected to search for scientific articles related to the title on Web of Science, PubMed, and ScienceDirect databases. The databases were searched for articles published between 2000 - 2022. The start and end dates of the search were recorded in each of the databases, and duplicate or unrelated studies were removed from the titles. To achieve the objectives of the study, the selected articles were reviewed by two reviewers. The process for selecting studies to be eligible for data analysis consisted of performing an advanced search using some terms, including (biohydrogen) AND (sludge processing) AND (dark fermentation) AND (photofermentation) AND (bioelectrochemical) AND (microbial fuel cell) AND (anaerobic digestion) AND (microbial electrolysis cell) AND (bioplastic) AND (polyhydroxyalkanoates).
Figure 1 depicts the flow diagram for the inclusion of the studies in the systematic review. From the total number of 315 papers identified first in different studied databases, 116, 83, and 124 papers were collected from Web of Science, PubMed, and ScienceDirect, respectively. After reviewing the titles and excluding duplicate and unrelated articles, 210 papers were selected. Then, 130 studies were selected after reviewing the abstracts and excluding 80 articles. Afterward, the full texts of the papers were read, and 50 papers were excluded from the study due to insufficient data. Finally, 80 articles were selected and further studied.
Flow diagram for inclusion of studies in the systematic review
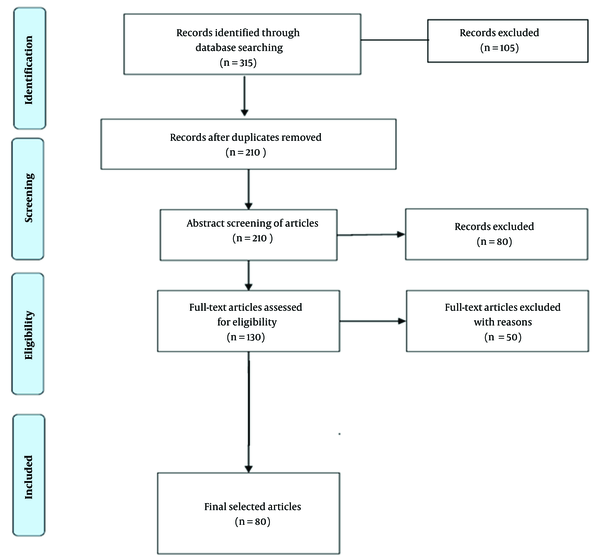
4. Results and Discussion
4.1. Dark Fermentation Process to Produce Biohydrogen
In the dark fermentation process, the acidogenesis fermentation of organic materials is performed in an environment without oxygen and light to produce biohydrogen, biogas, and carbon dioxide (28, 29). Additionally, in this process, effluent containing metabolites of final products (e.g., butyric acid, acetic acid, and ethanol) is generated. In dark fermentation, biohydrogen production occurs via a chain of biochemical reactions (28). Moreover, in this process, the biohydrogen yield production and the effluent composition largely depend on the microorganisms’ metabolic route. Most literature sources investigate the microorganism species of the Clostridium genus due to their high hydrogen production rates. In addition, these bacteria possess ideal properties for industrial application, including forming endospores and high growth rates (30).
Clostridium spp. also allows mixed culture hydrogen production, in which the variety of species present facilitates the efficient decomposition and conversion of organic waste into hydrogen. The conversion process is generally accompanied by organic acid production (30). Other commonly used enteric bacteria, such as Escherichia coli, Enterobacter aerogenes, and Pseudomonas, can also be applied for hydrogen production via fermentation (31). Controlling the microbial community in the fermenter is necessary for obtaining sustainable industrial-scale hydrogen production because the used substrate might contain microorganisms that affect the microbial community within the fermenter, leading to unfavorable conditions and lower hydrogen production rates. Necessary procedures should be taken into consideration, for example, sterilization (32). Figure 2 shows two metabolic pathways for biohydrogen production by microorganisms in this process. The final products of the dark fermentation process include volatile fatty acids (i.e., acetate and butyrate) and alcohol (ethanol), which can be used as primary biomass in other processes, such as anaerobic digestion systems, photofermentation, and bioelectrochemical systems, and the production of bioplastics (28, 29). Table 1 shows some studies in which the dark fermentation process was used.
Dark fermentation process
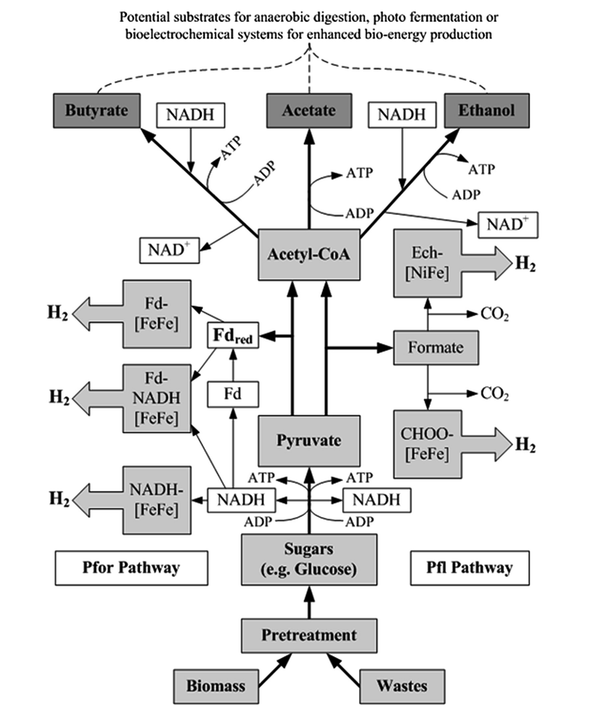
Studies Performed with Dark Fermentation Process
Substrate | Inoculating Liquid | Hydrogen Production Efficiency | Publication Year | Ref. |
---|---|---|---|---|
Glucose | Anaerobic sludge | 0.89 mL H2/g xylose | 2015 | (33) |
Glucose | Sewage treatment plant sludge | 0.38 mol H2/mol glucose | 2011 | (34) |
Glucose | Sewage treatment plant sludge | 0.7 mol H2/mol glucose | 2010 | (35) |
No substrate | Sewage treatment plant sludge | 0.00005 mol H2/g VS | 2009 | (36) |
Glucose | Sewage treatment plant sludge | 0.0106 mol H2/g carbohydrate | 2011 | (37) |
Bacteria | Sewage treatment plant sludge | 1.32 mol H2/mol glucose | 2010 | (38) |
Apple fruit | Sewage treatment plant sludge | 0.00423 mol H2/g TS | 2010 | (39) |
Corn | Sewage treatment plant sludge | 0.0058 mol H2/g corn stalk | 2012 | (40) |
Cheese | Anaerobic sludge | 111 mL H2/g sugar | 2012 | (41) |
Rice straw | Sewage treatment plant sludge | 24.8 mL H2/g sugar | 2012 | (42) |
Chicken meat | Anaerobic sludge | 6.9 mL H2/g sugar | 2014 | (43) |
Buffalo manure | Anaerobic sludge | 135.6 mL H2/g sugar | 2015 | (28) |
4.2. Disadvantages of the Dark Fermentation Process
Some of the disadvantages of the dark fermentation process obtained by reviewing the studies can be mentioned as follows:
(i) The low production efficiency of biohydrogen from biomass is, therefore, not economically desirable on a large scale. The researchers in the studies stated that the low biohydrogen production efficiency in the process of dark fermentation is the hydrogen consumers in the mixed microflora, for example, hematogenesis, hydrogenotrophic metagenesis, nitrate-reducing bacteria, sulfate-reducing bacteria, and propionate-producing bacteria. Therefore, placing a pre-treatment unit before the dark fermentation process is necessary, which leads to an increase in costs.
(ii) Effluent production with a high concentration of volatile fatty acids.
(iii) The dark fermentation process requires mixed microflora and pure hydrogen production environments, which is not practical for producing pure hydrogen on a large scale.
(iv) Biohydrogen production in the dark fermentation process is affected by various parameters, including inoculation, temperature, pH, partial pressure of hydrogen, hydraulic retention time, type of bioreactor, type and concentration of growth medium, and type and concentration of nutrients.
(v) One of the main problems in biohydrogen production in the dark fermentation process is the cost of raw materials.
(vi) The dark fermentation process is very complicated due to the presence of anaerobic conditions and the simultaneous production of several products (e.g., acids and alcohols with a mixture of gases).
(vii) Various inhibitors, such as the presence of other microorganisms in the medium of mixed cultures, metal ions (light and heavy), materials from the initial pretreatment of the growth medium (e.g., phenolic compounds and furan derivatives), ammonia, and soluble metabolites concentrations, are effective in the amount and speed of biohydrogen production in the dark fermentation process (36, 44-47). To improve the performance of dark fermentation, it is essential to use several microorganisms capable of high hydrogen yields, such as Bacillus amyloliquefaciens and Clostridium pasteurianum, and to adopt some advanced techniques of co-culture fermentation (48).
4.3. Advantages of the Dark Fermentation Process
Some of the advantages of the dark fermentation process obtained by reviewing the studies can be mentioned as follows:
(i) The dark fermentation process does not require light.
(ii) Energy consumption is less in the dark fermentation process.
(iii) Compared to other processes, the yield of biohydrogen production is higher in the dark fermentation process.
(iv) Dark fermentation is easier to control than other processes.
(v) Compared to other processes, the dark fermentation process has lower operating costs.
(vi) The dark fermentation process has a broader possibility for industrialization.
(vii) In the dark fermentation process, different growth types of yeasts can be used (5, 36, 49, 50).
As mentioned in the previous sections, the dark fermentation process has disadvantages. Based on the reviewed studies, these disadvantages can be solved by combining the process of dark fermentation with other processes, such as anaerobic digestion, light fermentation, and bioelectrochemical systems. Nevertheless, combining the fermentation process with other processes has its advantages and disadvantages, which will be presented below.
4.4. Advantages of Combining Dark Fermentation Process with Other Processes
Combining the process of dark fermentation with other processes leads to an enhancement in bioenergy production from the dark fermentation process and the possibility of its implementation on a large scale (29, 51, 52).
4.5. Disadvantages of Combining Dark Fermentation Process with Other Processes
Some of the disadvantages of combining dark fermentation with other processes obtained by reviewing the studies can be mentioned as follows:
(i) In combining the process of dark fermentation with other processes, the number of required reactors increases, which results in an enhancement in the cost of maintenance and operation of the entire system.
(ii) The effluent of the dark fermentation process contains high amounts of volatile fatty acids. Therefore, a neutralization step is needed to combine it with other processes, which increases costs due to the high consumption of alkali.
(iii) High levels of some compounds, such as metal ions and ammonium, in the dark fermentation effluent, might prevent the methanogen stage in anaerobic digestion (51, 52).
4.6. Combining Dark Fermentation Process with Anaerobic Digestion
In the anaerobic digestion process, organic materials, such as carbohydrates, proteins, and fats, are decomposed in an oxygen-free environment by a microbial consortium, which produces biogas, mainly carbon dioxide and methane. Biogas has a high content of energy that can be applied to produce heat and electricity. The anaerobic digestion process includes four stages, hydrolysis, acidogenesis, acetogenesis, and methanogenesis, as shown in Figure 3. Since the final yields of the dark fermentation process are volatile fatty acids, these products can be used as primary biomass in the anaerobic digestion process. According to the studies, the biohydrogen production efficiency increases when the dark fermentation process is combined with the anaerobic digestion process (53-56). Table 2 shows some studies in which the dark fermentation process was combined with the anaerobic digestion process.
Anaerobic digestion process (29)
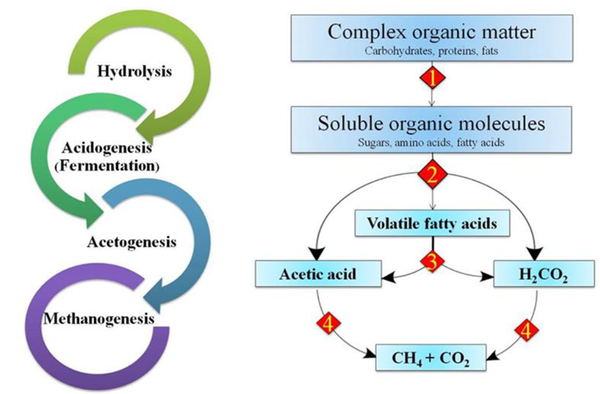
Studies Conducted with Dark Fermentation Process Combined with Anaerobic Digestion Process
Substrate | Inoculating Liquid | Hydrogen Production Efficiency | Publication Year | Ref. |
---|---|---|---|---|
Xylose | Anaerobic activated sludge | Dark fermentation: 0.191 mL H2/g xylose; dark fermentation + anaerobic digestion: 0.191 L H2/g xylose + 0.217 L CH4/g xylose | 2012 | (53) |
Cassava residues | Anaerobic digester sludge | Dark fermentation: 0.106 L H2/g VS; dark fermentation + anaerobic digestion: 0.106 L H2/g VS + 0.075 L CH4/g VS | 2015 | (57) |
Cassava residues | Anaerobic digester sludge | Dark fermentation: 0.102 L H2/g VS; dark fermentation + anaerobic digestion: 0.102 L H2/g VS + 0.093 L CH4/g VS | 2015 | (57) |
Food waste | Anaerobic digester sludge | Dark fermentation: 0.074 L H2/g VS; dark fermentation + anaerobic digestion: 0.074 L H2/g VS + 139.0 L CH4/g VS | 2016 | (54) |
Sewage sludge | Anaerobic digester sludge | Dark fermentation: 0.175 L H2/g VS; dark fermentation + anaerobic digestion: 0.175 L H2/g VS + 0.264 L CH4/g VS | 2016 | (54) |
Food waste + sewage sludge | Anaerobic digester sludge | Dark fermentation: 0.039 L H2/g VS; dark fermentation + anaerobic digestion: 0.039 L H2/g VS + 0.097 L CH4/g VS | 2016 | (49) |
Cassava residues | Anaerobic digester sludge | Dark fermentation: 0.092 L H2/g VS; dark fermentation + anaerobic digestion: 0.092 L H2/g VS + 0.079 L CH4/g VS | 2017 | (58) |
Laminaria digitata + Chlorella pyrenoidosa | Anaerobic digester sludge | Dark fermentation: 0.097 L H2/g VS; dark fermentation + anaerobic digestion: 0.097 L H2/g VS + 0.224 L CH4/g VS | 2016 | (55) |
Laminaria digitata + Nannochloropsis oceanica | Anaerobic digester sludge | Dark fermentation: 0.095 L H2/g VS; dark fermentation + anaerobic digestion: 0.095 L H2/g VS + 0.296 L CH4/g VS | 2016 | (55) |
Laminaria digitata + Saccharinalatissima | Anaerobic digester | Dark fermentation: 0.067 L H2/g VS; dark fermentation + anaerobic digestion: 0.067 L H2/g VS + 0.234 L CH4/g VS | 2016 | (55) |
Spartina anglica biomass | Anaerobic digester | Dark fermentation: 0.136 L H2/g VS; dark fermentation + anaerobic digestion: 0.136 L H2/g VS + 0.269 L CH4/g VS | 2016 | (56) |
Wheat straw hydrolysate | Anaerobic environment (dark fermentation), metagenic granules, and digested manure (anaerobic digestion) | Dark fermentation: 0.089 L H2/g VS; dark fermentation + anaerobic digestion: 0.089 L H2/g VS + 0.307 L CH4/g VS | 2011 | (59) |
Sugarcane bagasse | Cow manure | Dark fermentation: 0.093 L H2/g VS; dark fermentation + anaerobic digestion: 0.093 L H2/g VS + 0.222 L CH4/g VS | 2015 | (47) |
Microalgae biomass | Granular anaerobic sludge | Dark fermentation: 0.045 L H2/g VS; dark fermentation + anaerobic digestion: 0.045 L H2/g VS + 0.431 L CH4/g VS | 2016 | (60) |
Water hyacinth leaves | Anaerobic sludge | Dark fermentation: 0.0368 L H2/g VS; dark fermentation + anaerobic digestion: 0.0368 L H2/g VS + 0.15 L CH4/g VS | 2010 | (61) |
4.7. Photofermentation
Fermentation processes that use light as an energy source for photosynthesis are called photofermentation. One of the advantages of photofermentation is that light can replace sugars as an energy source, thereby reducing competition for land use with food crops. Photosynthetic bacteria, such as Rhodobacter sphaeroides and Rhodospirillum, are used in fermentation, and they use small molecular organic acids as a source of carbon (62). Another advantage of photofermentation is the higher theoretical yield of H2 than dark fermentation. In addition, purple non-sulfur microorganisms can absorb and utilize a wide spectrum of light (400 - 900 nm) and can utilize organic substrates derived from various wastes (19, 63).
The industrialization of natural photofermentation is limited by the availability and distribution of light, as self-shadowing must be minimized in an industrial-scale photofermenter. The second limitation imposes a significant surface-to-volume ratio for an externally exposed photobioreactor. In turn, this leads to the expensive construction of the photobioreactor (31). In addition, photofermentation requires specific substrates, namely mainly smaller fatty acids, such as acetate, propionate, and butyrate (48).
4.8. Combining Dark Fermentation Process with Photofermentation
In the photofermentation process, photosynthetic bacteria produce hydrogen and carbon dioxide from organic compounds, such as purple non-sulfate bacteria, in the light presence, without oxygen and nitrogen deficiency. The requirement for light penetration is the major photofermentation disadvantage that leads to the complex design of these processes. Combining the process of dark fermentation and photofermentation does not solve the problem of light penetration. When combining these processes, the dark fermentation effluent must be pre-treated before entering the photofermentation process to increase light penetration. Therefore, an intermediary step is needed, which leads to an increase in costs in the entire system (46, 51, 64).
On the other hand, because the effluent of the dark fermentation process contains high concentrations of volatile fatty acid and ammonium, this might prevent the light fermentation process. In some studies, to solve this problem, the dark fermentation effluent was diluted before entering the light fermentation process. Some studies used natural zeolite to remove ammonium (46, 51, 52, 64-68). There are studies on the combination of dark fermentation and photofermentation, and these studies consider this method to be the most promising method for industrial hydrogen fermentation due to its economy and reduction in the number of side products. The main advantage of using mixed fermentation is due to its ability to reuse non-useful organic acids in the photosynthesis stage. However, hybrid fermentation also faces problems in optimizing reactor design and minimizing energy consumption, both of which are major drawbacks of photofermentation. These efforts showed that photofermentation is effective in the further valorization of dark fermentation effluent (31, 50, 69). Table 3 shows some studies in which the dark fermentation process was combined with the photofermentation process.
Studies Conducted with Dark Fermentation Process Combined with Photofermentation Process
Substrate | Inoculating Liquid | Hydrogen Production Efficiency | Publication Year | Ref. |
---|---|---|---|---|
Synthetic wastewater | Dark fermentation: Anaerobic consortium; photofermentation: Dominant Chlorella culture medium | Dark fermentation: 1.21 mmol; dark fermentation + photofermentation: 6.43 mmol | 2011 | (46) |
Food waste | Dark fermentation: Anaerobic consortium; photofermentation: Dominant Chlorella culture medium | Dark fermentation: 0.16 mmol; dark fermentation + photofermentation: 1.40 mmol | 2011 | (46) |
Sucrose | Dark fermentation: Clostridium pasteurianum; photofermentation: Rhodopseudomonas palustris | Dark fermentation: 1.80 mol/mol sucrose; dark fermentation + photofermentation: 10.02 mol/mol sucrose | 2008 | (70) |
Rice stem | Dark fermentation: Anaerobic digester sludge; photofermentation: Rhodopseudomonas palustris | Dark fermentation: 0.135 L/g VS; dark fermentation + photofermentation: 0.463 L/g VS | 2011 | (71) |
Arthrospira platensis wet biomass | Dark fermentation: Anaerobic digester sludge; photofermentation: Rhodopseudomonas palustris | Dark fermentation: 0.097 L/g TS; dark fermentation + photofermentation: 0.337 L/g TS | 2012 | (52) |
Water hyacinth | Dark fermentation: Anaerobic digester sludge; photofermentation: Rhodopseudomonas palustris | Dark fermentation: 0.112 L/g VS; dark fermentation + photofermentation: 0.752 L/g VS | 2013 | (72) |
Glycerol | Dark fermentation: Klebsiella spp. Photofermentation: Rhodopseudomonas palustris | Dark fermentation: 5.74 mmol/g COD consumed; dark fermentation + photofermentation: 6.42 mmol/g COD consumed | 2015 | (73) |
Hydrolyzed starch | Dark fermentation: Clostridium butyricum; photofermentation: Rhodopseudomonas palustris | Dark fermentation: 5.40 mmol/g COD; dark fermentation + photofermentation: 12.16 mmol/g COD | 2008 | (65) |
Effluent from palm oil factory | Dark fermentation: Clostridium butyricum; photofermentation: Rhodopseudomonas palustris | Dark fermentation: 0.784 L/L POME; dark fermentation + photofermentation: 3.064 L/L POME | 2016 | (66) |
Beet molasses | Dark fermentation: Caldicellulosiruptor saccharolyticus; photofermentation: Mixed photosynthetic bacteria | Dark fermentation: 2.4 mol/mol sucrose; dark fermentation + photofermentation: 13.7 mol/mol sucrose | 2010 | (67) |
Sugarcane bagasse hydrolyzed with acid | Dark fermentation: Enterobacter aerogenes MTCC 2822; photofermentation: Rhodopseudomonas BHU 01 | Dark fermentation: 1.0 L/L; dark fermentation + photofermentation: 1.755 L/L | 2014 | (68) |
Cassava starch | Dark fermentation: Activated sludge; photofermentation: Rhodopseudomonas palustris | Dark fermentation: 0.240 L/g starch; dark fermentation + photofermentation: 0.402 L/g starch | 2009 | (45) |
Glucose | Dark fermentation: Clostridium buryticum; photofermentation: Rhodopseudomonas palustris | Dark fermentation: 1.59 mol/mol glucose; dark fermentation + photofermentation: 5.48 mol/mol glucose | 2009 | (51) |
Water hyacinth | Dark fermentation: Anaerobic activated sludge; photofermentation: Rhodopseudomonas palustris | Dark fermentation: 0.074 L/g VS; dark fermentation + photofermentation: 0.596 L/g VS | 2010 | (44) |
Sucrose | Dark fermentation: Sludge and cow dung; photofermentation: Rhodobacter sphaeroides | Dark fermentation: 3.67 mol/mol sucrose; dark fermentation + photofermentation: 6.63 mol/mol sucrose | 2007 | (50) |
4.9. Dark Fermentation Process for Bioplastic Production
Bioplastics are plastic materials produced from renewable biomass sources, for example, starch, vegetable oils and fats, cellulose, lactic acid, corn, straw, wood chips, recycled food waste, and sawdust (74, 75). In addition, microorganisms can produce bioplastics from plastic bottles and other containers and by-products of agriculture. Usual plastics, for example, fossil-fuel plastics (i.e., petroleum-based polymers), are made from natural gas or petroleum. According to statistics in 2014, bioplastics accounted for nearly 300 million tons of the global polymer market (76).
The degradability of bioplastics is a crucial feature. In addition, bioplastics are not only compatible with the environment but also compatible with the body. Therefore, bioplastics are expected to be used to produce medical products (e.g., surgical sutures). Among the degradable plastics prepared to date, a group called polyhydroxyalkanoates (PHAs) and their copolymers have more than 40 compounds due to their complete degradability and low production cost. Furthermore, the simplicity of the process compared to other biodegradable polymers has been considered. The PHA types are polyesters of various hydroxy acids that are produced by a large number of microorganisms as energy storage. There are more than 300 different types of PHA-producing microorganisms (77, 78).
Bacteria produce various types of PHAs as an energy and carbon source in the absence of essential nutrients, such as nitrogen and phosphorus, in the existence of an additional carbon source. However, PHA types are the best substitutes for petroleum-derived polymers. However, the high commercial production cost (more than 10 times that of synthetic plastics) is the main obstacle to their use in industry. The carbon source is a crucial parameter in enhancing the PHA production cost. To reduce the PHAs’ price, the new approach of using a mixture of sludge (i.e., the cultivation of a mixture of bacteria) has been considered in the last two decades (79, 80).
The economic evaluation shows that if activated sludge is used to produce PHAs, the cost of bioplastic production can be reduced by more than 50%. The PHAs can be produced by a bacterial consortium and volatile fatty acids from waste sludge. Using sludge to produce PHAs can turn waste into valuable products. Then, bioplastic production costs are reduced, and the sludge is also purified and recycled (81). The volatile fatty acids are the final yields of the dark fermentation process. On the other hand, the raw material for bioplastic production is volatile fatty acids. Therefore, dark fermentation with sludge substrate can be used to produce bioplastics. Table 4 shows some studies in which the dark fermentation process was used for bioplastic production.
Studies Conducted with Dark Fermentation Process for Bioplastic Production
Species of Microorganism | Type of Bioplastic Produced | Substrate Type | Bioplastic Production (%) | Publication Year | Ref. |
---|---|---|---|---|---|
Aeromonas hydrophila | P(3HB-co-3HV) | Glucose/lauric acid | 50 | 2001 | (82) |
Pseudomonas putida | PHASMCL | Oleic acid | 51 | 2000 | (83) |
Cupriavidus necator | P(3HB-co-4HB) | Glycerol | 36.1 | 2012 | (84) |
C. necator | P(3HB-4HB-3HV) | Glycerol | 36.9 | 2012 | (84) |
P. putida | PHASMCL | Glucose/nonanoic acid | 66.9 | 2007 | (85) |
C. necator | P(3HB) | Glucose/fructose | 68 | 2011 | (86) |
Burkholderia sacchari | P(3HB) | Sucrose | 42 | 2010 | (87) |
C. necator | P(3HB) | Glucose | 67 | 2003 | (88) |
Bacillus megaterium | P(3HB) | Sugarcane molasses | 42.1 | 2009 | (89) |
B. sacchari | P(3HB-co-3HV) | Sucrose | 45 | 2008 | (90) |
Mixed microbial culture | P(3HB/3HV) | Fermented sewage | 40 | 2007 | (91) |
Mixed microbial culture | P(3HB/3HV) | Primary sludge | 32 | 2007 | (92) |
Defluviicoccus vanus | PHA | Sugarcane molasses | 37.3 | 2010 | (93) |
Burkholderia sacchari | P(3HB) | wheat straw | 70 | 2014 | (94) |
Cupriavidus necator | P(3HB-co-3HV) | Glycerol | 78.9 | 2016 | (95) |
Mixed microbial culture | PHA | Brewery wastewater | 39 | 2016 | (96) |
Mixed microbial culture | PHA | Palm oil effluent | 70 | 2012 | (97) |
Mixed microbial culture | PHA | Fermented cheese | 58 | 2016 | (98) |
4.10. Bioelectrochemical Processes to Produce Biohydrogen
Research on bioelectrochemical processes can be a multi-disciplinary science, which includes microbiology, environmental engineering, biotechnology, and bioelectrochemistry (99-101). In bioelectrochemical processes, electroactive bacterial consortia or strains residing on the electrodes act as the biocatalyst for the degradation of organic matter present in wastewater, which generates electron equivalents required for anodic oxidation and cathodic reduction reactions (102, 103). Bioelectrochemical systems utilize biological processes, microorganisms, or enzymes in the production of electricity and other products, such as biohydrogen (104). Bioelectrochemical systems are usually described as a novel technology that transforms wastewater into electricity with the aid of microbes (105).
Bioelectrochemical systems for sludge processing are two types of processes, namely microbial electrolysis cell (MEC) and microbial fuel cell (MFC). In the MFC process, microorganisms can oxidize the organic substrate in the anode and convert it into protons (H+), electrons, and carbon dioxide under anaerobic conditions. Electrons move to the cathode through internal circulation and generate electricity. In contrast, protons (H+) move to the cathode through a semi-permeable membrane, react with oxygen, and generate water (25, 29, 106, 107).
In the MEC process, microorganisms use an electric current to produce biohydrogen from organic waste. The process of MEC is entirely similar to MFC, with the difference that an electric power source replaces the resistance, and the cathode part becomes free of oxygen. This process does not produce water because the cathode part is free of oxygen. The electric power source in MEC provides an additional current that leads to the regeneration of protons into biohydrogen. Bioelectrochemical systems’ effluents are heavily contaminated with salts, which require a pre-treatment system for removal (29, 107). Bioelectrochemical processes have also been combined with dark fermentation and photofermentation processes.
These systems are used in many applications, such as biosensors, wastewater treatment, and water desalination (108-110). As explained earlier, global research has experimented with numerous substrates suitable for MFCs, mainly to increase the amount of electricity generated (111, 112). There has been significant variation in these substrates, spanning from simple and single organic molecules to complex substrates. Industrial wastewater, made up of simple and complex organic pollutants, has been utilized as a substrate for MFCs (112). Figure 4 shows a schematic illustration of bioelectrochemical systems. In addition, Table 5 shows some studies in which bioelectrochemical processes have been used for electricity production.
Schematic illustration of bioelectrochemical processes (29)
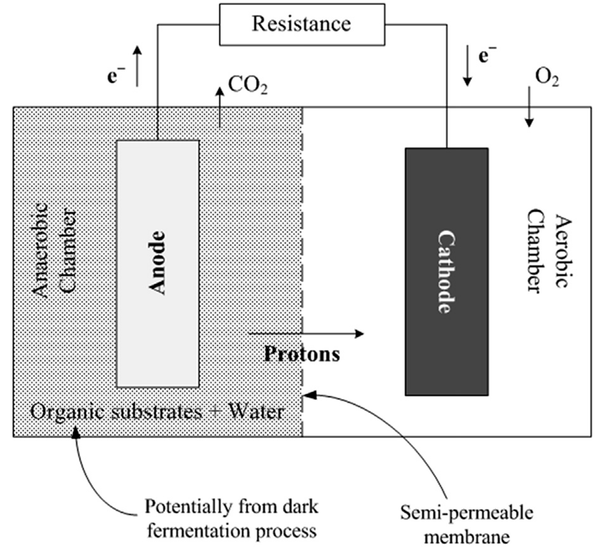
Studies Conducted with Bioelectrochemical Processes for Electricity Production
Substrate Type | Bioelectricity Production Efficiency | Publication Year | Ref. |
---|---|---|---|
Glycerol | 92 mW/m2 | 2014 | (64) |
Sucrose | 165 mW/m2 | 2014 | (113) |
Sucrose | 19.1 mW/m2 | 2015 | (114) |
Protein food industry wastewater | 230 mW/m2 | 2013 | (106) |
Dairy wastewater | 621 mW/m2 | 2014 | (115) |
Pig sewage | 45 mW/m2 | 2005 | (116) |
Cysteine | 39 mW/m2 | 2005 | (107) |
Anaerobic sewage | 274 mW/kg COD | 2007 | (117) |
Septic tank sewage sludge | 288 - 409 mW/m2 | 2010 | (118) |
Acetate | 506 mW/m2 | 2005 | (119) |
Butyrate | 305 mW/m2 | 2005 | (119) |
Acetate | 309 mW/m2 | 2004 | (120) |
Glucose | 220 mW/m2 | 2004 | (120) |
Chocolatier wastewater | 98 mW/m2 | 2019 | (121) |
Municipal wastewater | 78 mW/m2 | 2019 | (122) |
Dairy wastewater | 123.5 mW/m2 | 2019 | (123) |
4.11. Advantages and Disadvantages of MEC
The operating conditions of MEC are simple compared to other hydrogen production methods (124). The hydrogen produced in MEC is higher than in other hydrogen generation processes, such as fermentation-based processes (125). The purity of hydrogen produced via MEC is higher than other hydrogen generation methods. Due to the organic breakdown that occurs in dark fermentation, the hydrogen produced is normally accompanied by other gases, such as carbon dioxide (126). Hydrogen produced by MEC is considered to be ideal for various applications, as it is expected to be sulfur-free. The rate of hydrogen production is a key issue impeding the commercialization of this viable technology, i.e., MEC. For instance, the production of hydrogen, even under laboratory conditions, is limited to 3 m3 H2/m3/day (127). The hydrogen production rate undergoes deterioration when the entire process is scaled up. Some of the factors responsible for these phenomena include the reactor geometry, electrode material, glass fiber separators, possible connection resistances, and microbiological factors leading to slow start-up for the reactor (128). The integration of MEC with existing technologies provides an enormous impact in terms of futuristic industrial applications.
4.12. Microbial Electrolysis Cell for Hydrogen Production from Wastewater
Wastewater from different sources was recently investigated as a fuel to produce hydrogen in MEC. Table 6 summarizes some of the studies performed on hydrogen production by MEC from different wastewaters. As shown in Table 6, MEC is a viable option for simultaneous wastewater treatment and hydrogen production.
Hydrogen Production Using Wastewater in Microbial Electrolysis Cell
Type of Wastewater | Anode | Cathode | Hydrogen Production Rate | Publication Year | Ref. |
---|---|---|---|---|---|
Sugar industry | Graphite plate | NiO-rGO/Ni foam | 4.38 mmol/L/day | 2020 | (129) |
Sugar industry | Graphite plate | Co3O4-rGO/Ni foam | 3.66 mmol/L/day | 2020 | (129) |
Domestic | Graphite felt | Pt/carbon cloth | 0.18 m3/m3/day | 2019 | (130) |
Leachate and dairy | Carbon cloth | Graphite sheet | 15 mL/L/day | 2020 | (131) |
5. Conclusions
The continuous surge in global energy demand, fossil fuel depletion, and related climate change issues have oriented worldwide researchers’ endeavors to the investigation and development of sustainable and coeffective technology to satisfy global energy needs. Referring to the non-toxic properties of hydrogen, it is considered a suitable renewable energy source that can replace fossil fuel-based energy. It is the cleanest energy carrier, combustible with high calorific value and high energy yield. The studies demonstrated that the biohydrogen produced from the dark fermentation process has disadvantages. The combination of dark fermentation and photofermentation as the first and second stages, respectively, can increase the biohydrogen efficiency due to the conversion of more substrates into H2. Additionally, it can be partially solved by combining it with other processes, such as anaerobic digestion or bioelectrochemical systems. However, combining dark fermentation with other processes also has its disadvantages. Combining the dark fermentation process with other processes generally increases biohydrogen production. Moreover, the final products of the dark fermentation process can be used to produce electricity through bioelectrochemical systems and bioplastic production. By reviewing the studies, it was obtained that dark fermentation and bioelectrochemical processes were generally successful in producing energy and bioplastics. Future studies should focus on integrated processes, especially the integration of dark fermentation and biochemical processes in continuous reactors.
Acknowledgements
References
-
1.
Kamzon MA, Abderafi S, Bounahmidi T. Promising bioethanol processes for developing a biorefinery in the Moroccan sugar industry. Int J Hydrogen Energy. 2016;41(45):20880-96. https://doi.org/10.1016/j.ijhydene.2016.07.035.
-
2.
Fytili D, Zabaniotou A. Utilization of sewage sludge in EU application of old and new methods—A review. Renew Sustain Energy Rev. 2008;12(1):116-40. https://doi.org/10.1016/j.rser.2006.05.014.
-
3.
Nikolaidis P, Poullikkas A. A comparative overview of hydrogen production processes. Renew Sustain Energy Rev. 2017;67:597-611. https://doi.org/10.1016/j.rser.2016.09.044.
-
4.
Hosseini SE, Wahid MA. Hydrogen production from renewable and sustainable energy resources: Promising green energy carrier for clean development. Renew Sustain Energy Rev. 2016;57:850-66. https://doi.org/10.1016/j.rser.2015.12.112.
-
5.
Kim SH, Han SK, Shin HS. Feasibility of biohydrogen production by anaerobic co-digestion of food waste and sewage sludge. Int J Hydrogen Energy. 2004;29(15):1607-16. https://doi.org/10.1016/j.ijhydene.2004.02.018.
-
6.
Manara P, Zabaniotou A. Towards sewage sludge based biofuels via thermochemical conversion – A review. Renew Sustain Energy Rev. 2012;16(5):2566-82. https://doi.org/10.1016/j.rser.2012.01.074.
-
7.
Jung KW, Kim DH, Kim SH, Shin HS. Bioreactor design for continuous dark fermentative hydrogen production. Bioresour Technol. 2011;102(18):8612-20. [PubMed ID: 21489782]. https://doi.org/10.1016/j.biortech.2011.03.056.
-
8.
Kumar G, Shobana S, Nagarajan D, Lee DJ, Lee KS, Lin CY, et al. Biomass based hydrogen production by dark fermentation-recent trends and opportunities for greener processes. Curr Opin Biotechnol. 2018;50:136-45. [PubMed ID: 29367127]. https://doi.org/10.1016/j.copbio.2017.12.024.
-
9.
Dinesh GK, Chauhan R, Chakma S. Influence and strategies for enhanced biohydrogen production from food waste. Renew Sustain Energy Rev. 2018;92:807-22. https://doi.org/10.1016/j.rser.2018.05.009.
-
10.
Liu H, Grot S, Logan BE. Electrochemically assisted microbial production of hydrogen from acetate. Environ Sci Technol. 2005;39(11):4317-20. [PubMed ID: 15984815]. https://doi.org/10.1021/es050244p.
-
11.
Logan BE, Regan JM. Microbial Fuel Cells—Challenges and Applications. Environ Sci Technol. 2006;40(17):5172-80. https://doi.org/10.1021/es0627592.
-
12.
Steinberg M, Cheng HC. Modern and prospective technologies for hydrogen production from fossil fuels. Int J Hydrogen Energy. 1989;14(11):797-820. https://doi.org/10.1016/0360-3199(89)90018-9.
-
13.
Esposito DV, Hunt ST, Kimmel YC, Chen JG. A new class of electrocatalysts for hydrogen production from water electrolysis: metal monolayers supported on low-cost transition metal carbides. J Am Chem Soc. 2012;134(6):3025-33. [PubMed ID: 22280370]. https://doi.org/10.1021/ja208656v.
-
14.
Dufour J, Serrano DP, Gálvez JL, Moreno J, González A. Hydrogen Production from Fossil Fuels: Life Cycle Assessment of Technologies with Low Greenhouse Gas Emissions. Energy Fuels. 2011;25(5):2194-202. https://doi.org/10.1021/ef200124d.
-
15.
Singh R, White D, Demirel Y, Kelly R, Noll K, Blum P. Uncoupling Fermentative Synthesis of Molecular Hydrogen from Biomass Formation in Thermotoga maritima. Appl Environ Microbiol. 2018;84(17):e00998-18. [PubMed ID: 29959252]. [PubMed Central ID: PMC6102995]. https://doi.org/10.1128/AEM.00998-18.
-
16.
Łukajtis R, Rybarczyk P, Kucharska K, Konopacka-Łyskawa D, Słupek E, Wychodnik K, et al. Optimization of Saccharification Conditions of Lignocellulosic Biomass under Alkaline Pre-Treatment and Enzymatic Hydrolysis. Energies. 2018;11(4):886. https://doi.org/10.3390/en11040886.
-
17.
Kucharska K, Lukajtis R, Slupek E, Cieslinski H, Rybarczyk P, Kaminski M. Hydrogen Production from Energy Poplar Preceded by MEA Pre-Treatment and Enzymatic Hydrolysis. Molecules. 2018;23(11):3029. [PubMed ID: 30463326]. [PubMed Central ID: PMC6278490]. https://doi.org/10.3390/molecules23113029.
-
18.
Karmann S, Panke S, Zinn M. Fed-Batch Cultivations of Rhodospirillum rubrum Under Multiple Nutrient-Limited Growth Conditions on Syngas as a Novel Option to Produce Poly(3-Hydroxybutyrate) (PHB). Front Bioeng Biotechnol. 2019;7:59. [PubMed ID: 31001525]. [PubMed Central ID: PMC6454858]. https://doi.org/10.3389/fbioe.2019.00059.
-
19.
Ghimire A, Frunzo L, Pirozzi F, Trably E, Escudie R, Lens PNL, et al. A review on dark fermentative biohydrogen production from organic biomass: Process parameters and use of by-products. Appl Energy. 2015;144:73-95. https://doi.org/10.1016/j.apenergy.2015.01.045.
-
20.
Lora Grando R, de Souza Antune AM, da Fonseca FV, Sánchez A, Barrena R, Font X. Technology overview of biogas production in anaerobic digestion plants: A European evaluation of research and development. Renew Sustain Energy Rev. 2017;80:44-53. https://doi.org/10.1016/j.rser.2017.05.079.
-
21.
Parthiba Karthikeyan O, Trably E, Mehariya S, Bernet N, Wong JWC, Carrere H. Pretreatment of food waste for methane and hydrogen recovery: A review. Bioresour Technol. 2018;249:1025-39. [PubMed ID: 29111164]. https://doi.org/10.1016/j.biortech.2017.09.105.
-
22.
Monnet F. An introduction to anaerobic digestion of organic wastes. 2003. Available from: https://www.cti2000.it/Bionett/BioG-2003-002%20IntroAnaerobicDigestion.pdf.
-
23.
Wang P, Wang H, Qiu Y, Ren L, Jiang B. Microbial characteristics in anaerobic digestion process of food waste for methane production-A review. Bioresour Technol. 2018;248(Pt A):29-36. [PubMed ID: 28779951]. https://doi.org/10.1016/j.biortech.2017.06.152.
-
24.
Ariunbaatar J, Panico A, Esposito G, Pirozzi F, Lens PNL. Pretreatment methods to enhance anaerobic digestion of organic solid waste. Appl Energy. 2014;123:143-56. https://doi.org/10.1016/j.apenergy.2014.02.035.
-
25.
Hamelers HV, Ter Heijne A, Sleutels TH, Jeremiasse AW, Strik DP, Buisman CJ. New applications and performance of bioelectrochemical systems. Appl Microbiol Biotechnol. 2010;85(6):1673-85. [PubMed ID: 20024546]. https://doi.org/10.1007/s00253-009-2357-1.
-
26.
Logan BE, Rabaey K. Conversion of wastes into bioelectricity and chemicals by using microbial electrochemical technologies. Science. 2012;337(6095):686-90. [PubMed ID: 22879507]. https://doi.org/10.1126/science.1217412.
-
27.
Harding KG, Dennis JS, von Blottnitz H, Harrison ST. Environmental analysis of plastic production processes: comparing petroleum-based polypropylene and polyethylene with biologically-based poly-beta-hydroxybutyric acid using life cycle analysis. J Biotechnol. 2007;130(1):57-66. [PubMed ID: 17400318]. https://doi.org/10.1016/j.jbiotec.2007.02.012.
-
28.
Ghimire A, Frunzo L, Pontoni L, d'Antonio G, Lens PN, Esposito G, et al. Dark fermentation of complex waste biomass for biohydrogen production by pretreated thermophilic anaerobic digestate. J Environ Manage. 2015;152:43-8. [PubMed ID: 25617867]. https://doi.org/10.1016/j.jenvman.2014.12.049.
-
29.
Bundhoo ZMA. Coupling dark fermentation with biochemical or bioelectrochemical systems for enhanced bio-energy production: A review. Int J Hydrogen Energy. 2017;42(43):26667-86. https://doi.org/10.1016/j.ijhydene.2017.09.050.
-
30.
Krupp M, Widmann R. Biohydrogen production by dark fermentation: Experiences of continuous operation in large lab scale. Int J Hydrogen Energy. 2009;34(10):4509-16. https://doi.org/10.1016/j.ijhydene.2008.10.043.
-
31.
Rai PK, Singh SP, Asthana RK. Biohydrogen production from cheese whey wastewater in a two-step anaerobic process. Appl Biochem Biotechnol. 2012;167(6):1540-9. [PubMed ID: 22183564]. https://doi.org/10.1007/s12010-011-9488-4.
-
32.
Orozco RL, Redwood MD, Leeke GA, Bahari A, Santos RCD, Macaskie LE. Hydrothermal hydrolysis of starch with CO2 and detoxification of the hydrolysates with activated carbon for bio-hydrogen fermentation. Int J Hydrogen Energy. 2012;37(8):6545-53. https://doi.org/10.1016/j.ijhydene.2012.01.047.
-
33.
Si B, Li J, Li B, Zhu Z, Shen R, Zhang Y, et al. The role of hydraulic retention time on controlling methanogenesis and homoacetogenesis in biohydrogen production using upflow anaerobic sludge blanket (UASB) reactor and packed bed reactor (PBR). Int J Hydrogen Energy. 2015;40(35):11414-21. https://doi.org/10.1016/j.ijhydene.2015.04.035.
-
34.
Chang S, Li JZ, Liu F. Evaluation of different pretreatment methods for preparing hydrogen-producing seed inocula from waste activated sludge. Renew Energy. 2011;36(5):1517-22. https://doi.org/10.1016/j.renene.2010.11.023.
-
35.
Elbeshbishy E, Hafez H, Nakhla G. Enhancement of biohydrogen producing using ultrasonication. Int J Hydrogen Energy. 2010;35(12):6184-93. https://doi.org/10.1016/j.ijhydene.2010.03.119.
-
36.
Xiao B, Liu J. Effects of various pretreatments on biohydrogen production from sewage sludge. Sci Bull. 2009;54(12):2038-44. https://doi.org/10.1007/s11434-009-0100-z.
-
37.
Nasr N, Elbeshbishy E, Hafez H, Nakhla G, El Naggar MH. Bio-hydrogen production from thin stillage using conventional and acclimatized anaerobic digester sludge. Int J Hydrogen Energy. 2011;36(20):12761-9. https://doi.org/10.1016/j.ijhydene.2011.07.032.
-
38.
Baghchehsaraee B, Nakhla G, Karamanev D, Margaritis A. Fermentative hydrogen production by diverse microflora. Int J Hydrogen Energy. 2010;35(10):5021-7. https://doi.org/10.1016/j.ijhydene.2009.08.072.
-
39.
Wang H, Fang M, Fang Z, Bu H. Effects of sludge pretreatments and organic acids on hydrogen production by anaerobic fermentation. Bioresour Technol. 2010;101(22):8731-5. [PubMed ID: 20663663]. https://doi.org/10.1016/j.biortech.2010.06.131.
-
40.
Wang H, Zhi Z, Wang J, Ma S. Comparison of various pretreatment methods for biohydrogen production from cornstalk. Bioprocess Biosyst Eng. 2012;35(7):1239-45. [PubMed ID: 22451078]. https://doi.org/10.1007/s00449-012-0711-7.
-
41.
Kargi F, Eren NS, Ozmihci S. Bio-hydrogen production from cheese whey powder (CWP) solution: Comparison of thermophilic and mesophilic dark fermentations. Int J Hydrogen Energy. 2012;37(10):8338-42. https://doi.org/10.1016/j.ijhydene.2012.02.162.
-
42.
Chen CC, Chuang YS, Lin CY, Lay CH, Sen B. Thermophilic dark fermentation of untreated rice straw using mixed cultures for hydrogen production. Int J Hydrogen Energy. 2012;37(20):15540-6. https://doi.org/10.1016/j.ijhydene.2012.01.036.
-
43.
Guo XM, Trably E, Latrille E, Carrere H, Steyer JP. Predictive and explicative models of fermentative hydrogen production from solid organic waste: Role of butyrate and lactate pathways. Int J Hydrogen Energy. 2014;39(14):7476-85. https://doi.org/10.1016/j.ijhydene.2013.08.079.
-
44.
Su H, Cheng J, Zhou J, Song W, Cen K. Hydrogen production from water hyacinth through dark- and photo- fermentation. Int J Hydrogen Energy. 2010;35(17):8929-37. https://doi.org/10.1016/j.ijhydene.2010.06.035.
-
45.
Su H, Cheng J, Zhou J, Song W, Cen K. Improving hydrogen production from cassava starch by combination of dark and photo fermentation. Int J Hydrogen Energy. 2009;34(4):1780-6. https://doi.org/10.1016/j.ijhydene.2008.12.045.
-
46.
Chandra R, Venkata Mohan S. Microalgal community and their growth conditions influence biohydrogen production during integration of dark-fermentation and photo-fermentation processes. Int J Hydrogen Energy. 2011;36(19):12211-9. https://doi.org/10.1016/j.ijhydene.2011.07.007.
-
47.
Kumari S, Das D. Improvement of gaseous energy recovery from sugarcane bagasse by dark fermentation followed by biomethanation process. Bioresour Technol. 2015;194:354-63. [PubMed ID: 26210150]. https://doi.org/10.1016/j.biortech.2015.07.038.
-
48.
Baeyens J, Zhang H, Nie J, Appels L, Dewil R, Ansart R, et al. Reviewing the potential of bio-hydrogen production by fermentation. Renew Sustain Energy Rev. 2020;131:110023. https://doi.org/10.1016/j.rser.2020.110023.
-
49.
Cheng J, Ding L, Lin R, Yue L, Liu J, Zhou J, et al. Fermentative biohydrogen and biomethane co-production from mixture of food waste and sewage sludge: Effects of physiochemical properties and mix ratios on fermentation performance. Appl Energy. 2016;184:1-8. https://doi.org/10.1016/j.apenergy.2016.10.003.
-
50.
Tao Y, Chen Y, Wu Y, He Y, Zhou Z. High hydrogen yield from a two-step process of dark- and photo-fermentation of sucrose. Int J Hydrogen Energy. 2007;32(2):200-6. https://doi.org/10.1016/j.ijhydene.2006.06.034.
-
51.
Su H, Cheng J, Zhou J, Song W, Cen K. Combination of dark- and photo-fermentation to enhance hydrogen production and energy conversion efficiency. Int J Hydrogen Energy. 2009;34(21):8846-53. https://doi.org/10.1016/j.ijhydene.2009.09.001.
-
52.
Cheng J, Xia A, Liu Y, Lin R, Zhou J, Cen K. Combination of dark- and photo-fermentation to improve hydrogen production from Arthrospira platensis wet biomass with ammonium removal by zeolite. Int J Hydrogen Energy. 2012;37(18):13330-7. https://doi.org/10.1016/j.ijhydene.2012.06.071.
-
53.
Cheng J, Song W, Xia A, Su H, Zhou J, Cen K. Sequential generation of hydrogen and methane from xylose by two-stage anaerobic fermentation. Int J Hydrogen Energy. 2012;37(18):13323-9. https://doi.org/10.1016/j.ijhydene.2012.06.049.
-
54.
Cheng J, Ding L, Lin R, Liu M, Zhou J, Cen K. Physicochemical characterization of typical municipal solid wastes for fermentative hydrogen and methane co-production. Energy Convers Manag. 2016;117:297-304. https://doi.org/10.1016/j.enconman.2016.03.016.
-
55.
Ding L, Cheng J, Xia A, Jacob A, Voelklein M, Murphy JD. Co-generation of biohydrogen and biomethane through two-stage batch co-fermentation of macro- and micro-algal biomass. Bioresour Technol. 2016;218:224-31. [PubMed ID: 27371795]. https://doi.org/10.1016/j.biortech.2016.06.092.
-
56.
Ding L, Cheng J, Yue L, Liu J, Zhang L, Zhou J, et al. Fermentative hydrogen and methane co-production from pretreated Spartina anglica biomass with optimal saccharification effect under acid/alkali-assisted steam/microwave heating and enzymolysis. Energy Convers Manag. 2016;127:554-60. https://doi.org/10.1016/j.enconman.2016.09.045.
-
57.
Cheng J, Lin R, Ding L, Song W, Li Y, Zhou J, et al. Fermentative hydrogen and methane cogeneration from cassava residues: effect of pretreatment on structural characterization and fermentation performance. Bioresour Technol. 2015;179:407-13. [PubMed ID: 25553572]. https://doi.org/10.1016/j.biortech.2014.12.050.
-
58.
Cheng J, Zhang J, Lin R, Liu J, Zhang L, Cen K. Ionic-liquid pretreatment of cassava residues for the cogeneration of fermentative hydrogen and methane. Bioresour Technol. 2017;228:348-54. [PubMed ID: 28088097]. https://doi.org/10.1016/j.biortech.2016.12.107.
-
59.
Kongjan P, O-Thong S, Angelidaki I. Performance and microbial community analysis of two-stage process with extreme thermophilic hydrogen and thermophilic methane production from hydrolysate in UASB reactors. Bioresour Technol. 2011;102(5):4028-35. [PubMed ID: 21216592]. https://doi.org/10.1016/j.biortech.2010.12.009.
-
60.
Carrillo-Reyes J, Buitron G. Biohydrogen and methane production via a two-step process using an acid pretreated native microalgae consortium. Bioresour Technol. 2016;221:324-30. [PubMed ID: 27648852]. https://doi.org/10.1016/j.biortech.2016.09.050.
-
61.
Cheng J, Xie B, Zhou J, Song W, Cen K. Cogeneration of H2 and CH4 from water hyacinth by two-step anaerobic fermentation. Int JHydrogen Energy. 2010;35(7):3029-35. https://doi.org/10.1016/j.ijhydene.2009.07.012.
-
62.
Zhu Z, Shi J, Zhou Z, Hu F, Bao J. Photo-fermentation of Rhodobacter sphaeroides for hydrogen production using lignocellulose-derived organic acids. Process Biochem. 2010;45(12):1894-8. https://doi.org/10.1016/j.procbio.2010.08.017.
-
63.
Muhamad NS, Johan NA, Isa MH, Kutty SRM. Biohydrogen production using dark and photo fermentation: A mini review. 2011 National Postgraduate Conference. 19-20 September 2011; Perak, Malaysia. 2011. p. 1-9.
-
64.
Chookaew T, Prasertsan P, Ren ZJ. Two-stage conversion of crude glycerol to energy using dark fermentation linked with microbial fuel cell or microbial electrolysis cell. N Biotechnol. 2014;31(2):179-84. [PubMed ID: 24380781]. https://doi.org/10.1016/j.nbt.2013.12.004.
-
65.
Lo YC, Chen SD, Chen CY, Huang TI, Lin CY, Chang JS. Combining enzymatic hydrolysis and dark–photo fermentation processes for hydrogen production from starch feedstock: A feasibility study. Int J Hydrogen Energy. 2008;33(19):5224-33. https://doi.org/10.1016/j.ijhydene.2008.05.014.
-
66.
Mishra P, Thakur S, Singh L, Ab Wahid Z, Sakinah M. Enhanced hydrogen production from palm oil mill effluent using two stage sequential dark and photo fermentation. Int J Hydrogen Energy. 2016;41(41):18431-40. https://doi.org/10.1016/j.ijhydene.2016.07.138.
-
67.
Özgür E, Mars AE, Peksel B, Louwerse A, Yücel M, Gündüz U, et al. Biohydrogen production from beet molasses by sequential dark and photofermentation. Int J Hydrogen Energy. 2010;35(2):511-7. https://doi.org/10.1016/j.ijhydene.2009.10.094.
-
68.
Rai PK, Singh SP, Asthana RK, Singh S. Biohydrogen production from sugarcane bagasse by integrating dark- and photo-fermentation. Bioresour Technol. 2014;152:140-6. [PubMed ID: 24291314]. https://doi.org/10.1016/j.biortech.2013.10.117.
-
69.
Yang H, Guo L, Liu F. Enhanced bio-hydrogen production from corncob by a two-step process: dark- and photo-fermentation. Bioresour Technol. 2010;101(6):2049-52. [PubMed ID: 19963373]. https://doi.org/10.1016/j.biortech.2009.10.078.
-
70.
Chen CY, Yang MH, Yeh KL, Liu CH, Chang JS. Biohydrogen production using sequential two-stage dark and photo fermentation processes. Int J Hydrogen Energy. 2008;33(18):4755-62. https://doi.org/10.1016/j.ijhydene.2008.06.055.
-
71.
Cheng J, Su H, Zhou J, Song W, Cen K. Microwave-assisted alkali pretreatment of rice straw to promote enzymatic hydrolysis and hydrogen production in dark- and photo-fermentation. Int J Hydrogen Energy. 2011;36(3):2093-101. https://doi.org/10.1016/j.ijhydene.2010.11.021.
-
72.
Cheng J, Xia A, Su H, Song W, Zhou J, Cen K. Promotion of H2 production by microwave-assisted treatment of water hyacinth with dilute H2SO4 through combined dark fermentation and photofermentation. Energy Convers Manag. 2013;73:329-34. https://doi.org/10.1016/j.enconman.2013.05.018.
-
73.
Chookaew T, O-Thong S, Prasertsan P. Biohydrogen production from crude glycerol by two stage of dark and photo fermentation. Int J Hydrogen Energy. 2015;40(24):7433-8. https://doi.org/10.1016/j.ijhydene.2015.02.133.
-
74.
Chua H, Yu PH, Ma CK. Accumulation of biopolymers in activated sludge biomass. Appl Biochem Biotechnol. 1999;77-79:389-99. [PubMed ID: 15304709]. https://doi.org/10.1385/abab:78:1-3:389.
-
75.
Barnett R. Biodegradable plastic made from plants, not oil, is emerging. 2008. Available from: https://abcnews.go.com/Business/story?id=6528674&page=1.
-
76.
Künkel A, Becker J, Börger L, Hamprecht J, Koltzenburg S, Loos R, et al. Polymers, Biodegradable. Ullmann's encyclopedia of industrial chemistry. New York: Wiley; 2000. p. 1-29.
-
77.
Brockhaus S, Petersen M, Kersten W. A crossroads for bioplastics: exploring product developers' challenges to move beyond petroleum-based plastics. J Clean Prod. 2016;127:84-95. https://doi.org/10.1016/j.jclepro.2016.04.003.
-
78.
Serafim LS, Lemos PC, Oliveira R, Reis MA. Optimization of polyhydroxybutyrate production by mixed cultures submitted to aerobic dynamic feeding conditions. Biotechnol Bioeng. 2004;87(2):145-60. [PubMed ID: 15236243]. https://doi.org/10.1002/bit.20085.
-
79.
Shrivastav A, Kim HY, Kim YR. Advances in the applications of polyhydroxyalkanoate nanoparticles for novel drug delivery system. Biomed Res Int. 2013;2013:581684. [PubMed ID: 23984383]. [PubMed Central ID: PMC3741897]. https://doi.org/10.1155/2013/581684.
-
80.
Michinaka A, Arou J, Onuki M, Satoh H, Mino T. Analysis of polyhydroxyalkanoate (PHA) synthase gene in activated sludge that produces PHA containing 3-hydroxy-2-methylvalerate. Biotechnol Bioeng. 2007;96(5):871-80. [PubMed ID: 16933327]. https://doi.org/10.1002/bit.21085.
-
81.
Jia Q, Xiong H, Wang H, Shi H, Sheng X, Sun R, et al. Production of polyhydroxyalkanoates (PHA) by bacterial consortium from excess sludge fermentation liquid at laboratory and pilot scales. Bioresour Technol. 2014;171:159-67. [PubMed ID: 25194265]. https://doi.org/10.1016/j.biortech.2014.08.059.
-
82.
Chen GQ, Zhang G, Park SJ, Lee SY. Industrial scale production of poly(3-hydroxybutyrate-co-3-hydroxyhexanoate). Appl Microbiol Biotechnol. 2001;57(1-2):50-5. [PubMed ID: 11693933]. https://doi.org/10.1007/s002530100755.
-
83.
Lee SY, Wong HH, Choi JI, Lee SH, Lee SC, Han CS. Production of medium-chain-length polyhydroxyalkanoates by high-cell-density cultivation ofPseudomonas putida under phosphorus limitation. Biotechnol Bioeng. 2000;68(4):466-70. https://doi.org/10.1002/(sici)1097-0290(20000520)68:4<466::aid-bit12>3.0.co;2-t.
-
84.
Cavalheiro JM, Raposo RS, de Almeida MC, Cesario MT, Sevrin C, Grandfils C, et al. Effect of cultivation parameters on the production of poly(3-hydroxybutyrate-co-4-hydroxybutyrate) and poly(3-hydroxybutyrate-4-hydroxybutyrate-3-hydroxyvalerate) by Cupriavidus necator using waste glycerol. Bioresour Technol. 2012;111:391-7. [PubMed ID: 22382294]. https://doi.org/10.1016/j.biortech.2012.01.176.
-
85.
Sun Z, Ramsay JA, Guay M, Ramsay BA. Carbon-limited fed-batch production of medium-chain-length polyhydroxyalkanoates from nonanoic acid by Pseudomonas putida KT2440. Appl Microbiol Biotechnol. 2007;74(1):69-77. [PubMed ID: 17063330]. https://doi.org/10.1007/s00253-006-0655-4.
-
86.
Ienczak JL, Quines LK, de Melo AA, Brandellero M, Mendes CR, Schmidell W, et al. High cell density strategy for poly(3-hydroxybutyrate) production by Cupriavidus necator. Braz J Chem Eng. 2011;28(4):585-96. https://doi.org/10.1590/s0104-66322011000400004.
-
87.
Pradella JG, Taciro MK, Mateus AY. High-cell-density poly (3-hydroxybutyrate) production from sucrose using Burkholderia sacchari culture in airlift bioreactor. Bioresour Technol. 2010;101(21):8355-60. [PubMed ID: 20580221]. https://doi.org/10.1016/j.biortech.2010.05.046.
-
88.
Shang L, Jiang M, Chang HN. Poly(3-hydroxybutyrate) synthesis in fed-batch culture of Ralstonia eutropha with phosphate limitation under different glucose concentrations. Biotechnol Lett. 2003;25(17):1415-9. [PubMed ID: 14514042]. https://doi.org/10.1023/a:1025047410699.
-
89.
Kulpreecha S, Boonruangthavorn A, Meksiriporn B, Thongchul N. Inexpensive fed-batch cultivation for high poly(3-hydroxybutyrate) production by a new isolate of Bacillus megaterium. J Biosci Bioeng. 2009;107(3):240-5. [PubMed ID: 19269585]. https://doi.org/10.1016/j.jbiosc.2008.10.006.
-
90.
Rocha RCS, da Silva LF, Taciro MK, Pradella JGC. Production of poly(3-hydroxybutyrate-co-3-hydroxyvalerate) P(3HB-co-3HV) with a broad range of 3HV content at high yields by Burkholderia sacchari IPT 189. World J Microbiol Biotechnol. 2008;24(3):427-31. https://doi.org/10.1007/s11274-007-9480-x.
-
91.
Coats ER, Loge FJ, Wolcott MP, Englund K, McDonald AG. Synthesis of polyhydroxyalkanoates in municipal wastewater treatment. Water Environ Res. 2007;79(12):2396-403. [PubMed ID: 18044356]. https://doi.org/10.2175/106143007x183907.
-
92.
Gurieff N. Production of biodegradable polyhydroxyalkanoate polymers using advanced biological wastewater treatment process technology [dissertation]. St Lucia QLD, Australia: The University of Queensland; 2007.
-
93.
Bengtsson S, Pisco AR, Johansson P, Lemos PC, Reis MA. Molecular weight and thermal properties of polyhydroxyalkanoates produced from fermented sugar molasses by open mixed cultures. J Biotechnol. 2010;147(3-4):172-9. [PubMed ID: 20380854]. https://doi.org/10.1016/j.jbiotec.2010.03.022.
-
94.
Cesario MT, Raposo RS, de Almeida MC, van Keulen F, Ferreira BS, da Fonseca MM. Enhanced bioproduction of poly-3-hydroxybutyrate from wheat straw lignocellulosic hydrolysates. N Biotechnol. 2014;31(1):104-13. [PubMed ID: 24157713]. https://doi.org/10.1016/j.nbt.2013.10.004.
-
95.
Kachrimanidou V, Kopsahelis N, Vlysidis A, Papanikolaou S, Kookos IK, Monje Martínez B, et al. Downstream separation of poly(hydroxyalkanoates) using crude enzyme consortia produced via solid state fermentation integrated in a biorefinery concept. Food Bioprod Process. 2016;100:323-34. https://doi.org/10.1016/j.fbp.2016.08.002.
-
96.
Ben M, Kennes C, Veiga MC. Optimization of polyhydroxyalkanoate storage using mixed cultures and brewery wastewater. J ChemTechnol Biotechnol. 2016;91(11):2817-26. https://doi.org/10.1002/jctb.4891.
-
97.
Din MF, Mohanadoss P, Ujang Z, van Loosdrecht M, Yunus SM, Chelliapan S, et al. Development of Bio-PORec® system for polyhydroxyalkanoates (PHA) production and its storage in mixed cultures of palm oil mill effluent (POME). Bioresour Technol. 2012;124:208-16. [PubMed ID: 22989648]. https://doi.org/10.1016/j.biortech.2012.08.036.
-
98.
Colombo B, Pepe Sciarria T, Reis M, Scaglia B, Adani F. Polyhydroxyalkanoates (PHAs) production from fermented cheese whey by using a mixed microbial culture. Bioresour Technol. 2016;218:692-9. [PubMed ID: 27420156]. https://doi.org/10.1016/j.biortech.2016.07.024.
-
99.
Olabi AG, Wilberforce T, Sayed ET, Elsaid K, Rezk H, Abdelkareem MA. Recent progress of graphene based nanomaterials in bioelectrochemical systems. Sci Total Environ. 2020;749:141225. [PubMed ID: 32814206]. https://doi.org/10.1016/j.scitotenv.2020.141225.
-
100.
Kataki S, Chatterjee S, Vairale MG, Sharma S, Dwivedi SK, Gupta DK. Constructed wetland, an eco-technology for wastewater treatment: A review on various aspects of microbial fuel cell integration, low temperature strategies and life cycle impact of the technology. Renew Sustain Energy Rev. 2021;148:111261. https://doi.org/10.1016/j.rser.2021.111261.
-
101.
Ramírez-Vargas C, Prado A, Arias C, Carvalho P, Esteve-Núñez A, Brix H. Microbial Electrochemical Technologies for Wastewater Treatment: Principles and Evolution from Microbial Fuel Cells to Bioelectrochemical-Based Constructed Wetlands. Water. 2018;10(9):1128. https://doi.org/10.3390/w10091128.
-
102.
Mittal Y, Dash S, Srivastava P, Mishra PM, Aminabhavi TM, Yadav AK. Azo dye containing wastewater treatment in earthen membrane based unplanted two chambered constructed wetlands-microbial fuel cells: A new design for enhanced performance. Chem Eng J. 2022;427:131856. https://doi.org/10.1016/j.cej.2021.131856.
-
103.
Mohanakrishna G, Abu-Reesh IM, Pant D. Enhanced bioelectrochemical treatment of petroleum refinery wastewater with Labaneh whey as co-substrate. Sci Rep. 2020;10(1):19665. [PubMed ID: 33184377]. [PubMed Central ID: PMC7665216]. https://doi.org/10.1038/s41598-020-76668-0.
-
104.
Ivase TJP, Nyakuma BB, Oladokun O, Abu PT, Hassan MN. Review of the principal mechanisms, prospects, and challenges of bioelectrochemical systems. Environ Prog Sustain Energy. 2020;39(1):13298. https://doi.org/10.1002/ep.13298.
-
105.
Gude VG. Integrating bioelectrochemical systems for sustainable wastewater treatment. Clean Technol Environ Policy. 2018;20(5):911-24. https://doi.org/10.1007/s10098-018-1536-0.
-
106.
Mansoorian HJ, Mahvi AH, Jafari AJ, Amin MM, Rajabizadeh A, Khanjani N. Bioelectricity generation using two chamber microbial fuel cell treating wastewater from food processing. Enzyme Microb Technol. 2013;52(6-7):352-7. [PubMed ID: 23608504]. https://doi.org/10.1016/j.enzmictec.2013.03.004.
-
107.
Logan BE, Murano C, Scott K, Gray ND, Head IM. Electricity generation from cysteine in a microbial fuel cell. Water Res. 2005;39(5):942-52. [PubMed ID: 15743641]. https://doi.org/10.1016/j.watres.2004.11.019.
-
108.
Meena RAA, Yukesh Kannah R, Sindhu J, Ragavi J, Kumar G, Gunasekaran M, et al. Trends and resource recovery in biological wastewater treatment system. Bioresour Technol Rep. 2019;7:100235. https://doi.org/10.1016/j.biteb.2019.100235.
-
109.
Hua T, Li S, Li F, Zhou Q, Ondon BS. Microbial electrolysis cell as an emerging versatile technology: a review on its potential application, advance and challenge. J Chem Technol Biotechnol. 2019;94(6):1697-711. https://doi.org/10.1002/jctb.5898.
-
110.
Roy S, Pandit S. Microbial Electrochemical System: Principles and Application. In: Venkata Mohan S, Varjani S, Pandey A, editors. Microbial Electrochemical Technology: Sustainable Platform for Fuels, Chemicals and Remediation, A volume in Biomass, Biofuels and Biochemicals. Amsterdam: Elsevier; 2019. p. 19-48. https://doi.org/10.1016/b978-0-444-64052-9.00002-9.
-
111.
Pant D, Singh A, Van Bogaert G, Irving Olsen S, Singh Nigam P, Diels L, et al. Bioelectrochemical systems (BES) for sustainable energy production and product recovery from organic wastes and industrial wastewaters. RSC Adv. 2012;2(4):1248-63. https://doi.org/10.1039/c1ra00839k.
-
112.
Pandey P, Shinde VN, Deopurkar RL, Kale SP, Patil SA, Pant D. Recent advances in the use of different substrates in microbial fuel cells toward wastewater treatment and simultaneous energy recovery. Appl Energy. 2016;168:706-23. https://doi.org/10.1016/j.apenergy.2016.01.056.
-
113.
ElMekawy A, Srikanth S, Vanbroekhoven K, De Wever H, Pant D. Bioelectro-catalytic valorization of dark fermentation effluents by acetate oxidizing bacteria in bioelectrochemical system (BES). J Power Sources. 2014;262:183-91. https://doi.org/10.1016/j.jpowsour.2014.03.111.
-
114.
Pasupuleti SB, Srikanth S, Venkata Mohan S, Pant D. Continuous mode operation of microbial fuel cell (MFC) stack with dual gas diffusion cathode design for the treatment of dark fermentation effluent. Int J Hydrogen Energy. 2015;40(36):12424-35. https://doi.org/10.1016/j.ijhydene.2015.07.049.
-
115.
Jafari Mansoorian H, Mahvi AH, Jonidi Jafari A, Khanjani N. Evaluation of dairy industry wastewater treatment and simultaneous bioelectricity generation in a catalyst-less and mediator-less membrane microbial fuel cell. J Saudi Chem Soc. 2016;20(1):88-100. https://doi.org/10.1016/j.jscs.2014.08.002.
-
116.
Min B, Kim J, Oh S, Regan JM, Logan BE. Electricity generation from swine wastewater using microbial fuel cells. Water Res. 2005;39(20):4961-8. [PubMed ID: 16293279]. https://doi.org/10.1016/j.watres.2005.09.039.
-
117.
Mohan SV, Raghavulu SV, Srikanth S, Sarma PN. Bioelectricity production by mediatorless microbial fuel cell under acidophilic condition using wastewater as substrate: Influence of substrate loading rate. Curr Sci. 2007;92(12):1720-6.
-
118.
More TT, Ghangrekar MM. Improving performance of microbial fuel cell with ultrasonication pre-treatment of mixed anaerobic inoculum sludge. Bioresour Technol. 2010;101(2):562-7. [PubMed ID: 19736004]. https://doi.org/10.1016/j.biortech.2009.08.045.
-
119.
Liu H, Cheng S, Logan BE. Production of electricity from acetate or butyrate using a single-chamber microbial fuel cell. Environ Sci Technol. 2005;39(2):658-62. [PubMed ID: 15707069]. https://doi.org/10.1021/es048927c.
-
120.
Min B, Logan BE. Continuous electricity generation from domestic wastewater and organic substrates in a flat plate microbial fuel cell. Environ Sci Technol. 2004;38(21):5809-14. [PubMed ID: 15575304]. https://doi.org/10.1021/es0491026.
-
121.
Subha C, Kavitha S, Abisheka S, Tamilarasan K, Arulazhagan P, Rajesh Banu J. Bioelectricity generation and effect studies from organic rich chocolaterie wastewater using continuous upflow anaerobic microbial fuel cell. Fuel. 2019;251:224-32. https://doi.org/10.1016/j.fuel.2019.04.052.
-
122.
Hiegemann H, Littfinski T, Krimmler S, Lubken M, Klein D, Schmelz KG, et al. Performance and inorganic fouling of a submergible 255 L prototype microbial fuel cell module during continuous long-term operation with real municipal wastewater under practical conditions. Bioresour Technol. 2019;294:122227. [PubMed ID: 31610498]. https://doi.org/10.1016/j.biortech.2019.122227.
-
123.
Sekar AD, Jayabalan T, Muthukumar H, Chandrasekaran NI, Mohamed SN, Matheswaran M. Enhancing power generation and treatment of dairy waste water in microbial fuel cell using Cu-doped iron oxide nanoparticles decorated anode. Energy. 2019;172:173-80. https://doi.org/10.1016/j.energy.2019.01.102.
-
124.
Kitching M, Butler R, Marsili E. Microbial bioelectrosynthesis of hydrogen: Current challenges and scale-up. Enzyme Microb Technol. 2017;96:1-13. [PubMed ID: 27871368]. https://doi.org/10.1016/j.enzmictec.2016.09.002.
-
125.
Yuan H, Lu Y, Abu-Reesh IM, He Z. Bioelectrochemical production of hydrogen in an innovative pressure-retarded osmosis/microbial electrolysis cell system: experiments and modeling. Biotechnol Biofuels. 2015;8:116. [PubMed ID: 26273320]. [PubMed Central ID: PMC4535853]. https://doi.org/10.1186/s13068-015-0305-0.
-
126.
Gude VG, Kokabian B, Gadhamshetty V. Beneficial Bioelectrochemical Systems for Energy, Water, and Biomass Production. J Microb Biochem Technol. 2013;S6:5. https://doi.org/10.4172/1948-5948.s6-005.
-
127.
Escapa A, Mateos R, Martínez EJ, Blanes J. Microbial electrolysis cells: An emerging technology for wastewater treatment and energy recovery. From laboratory to pilot plant and beyond. Renew Sustain Energy Rev. 2016;55:942-56. https://doi.org/10.1016/j.rser.2015.11.029.
-
128.
Heidrich ES, Edwards SR, Dolfing J, Cotterill SE, Curtis TP. Performance of a pilot scale microbial electrolysis cell fed on domestic wastewater at ambient temperatures for a 12 month period. Bioresour Technol. 2014;173:87-95. [PubMed ID: 25285764]. https://doi.org/10.1016/j.biortech.2014.09.083.
-
129.
Jayabalan T, Matheswaran M, Preethi V, Naina Mohamed S. Enhancing biohydrogen production from sugar industry wastewater using metal oxide/graphene nanocomposite catalysts in microbial electrolysis cell. Int J Hydrogen Energy. 2020;45(13):7647-55. https://doi.org/10.1016/j.ijhydene.2019.09.068.
-
130.
Jwa E, Yun YM, Kim H, Jeong N, Park SC, Nam JY. Domestic wastewater treatment in a tubular microbial electrolysis cell with a membrane electrode assembly. Int J Hydrogen Energy. 2019;44(2):652-60. https://doi.org/10.1016/j.ijhydene.2018.11.036.
-
131.
Rani G, Nabi Z, Rajesh Banu J, Yogalakshmi KN. Batch fed single chambered microbial electrolysis cell for the treatment of landfill leachate. Renew Energy. 2020;153:168-74. https://doi.org/10.1016/j.renene.2020.01.118.