Abstract
Background:
Municipal solid waste (MSW) landfills are significant contributors to the emission of greenhouse gases, particularly methane, during the breakdown of waste materials. Researchers have undertaken extensive investigations into quantifying methane emissions from landfills, utilizing various methodologies beyond reliance on the LandGEM model. Numerous studies have underscored the efficacy of this model in predicting methane generation and exploring its utility in activities such as energy generation and emission mitigation strategies.Objectives:
Municipal solid waste landfills are vital contributors to greenhouse gas emissions, notably methane, during waste decomposition. Significant research has focused on estimating methane output from landfills, utilizing various approaches beyond the LandGEM model. Studies highlight the model's potential in assessing methane generation and its relevance to activities like energy generation and emission management.Methods:
The LandGEM simulation model is employed to forecast future waste generation and provide valuable insights into the environmental consequences of landfill management on the island. By analyzing population data and waste generation statistics from 2023 to 2042, the study utilizes the LandGEM software to estimate methane emissions, incorporating site-specific data and waste composition analysis.Results:
The findings reveal that 36% of the total waste on Kish Island consists of putrescible material, with food waste being a significant component. Over the study period, approximately 1,282,637 tons of waste are projected to be disposed of in the Kish landfill. The LandGEM model predicts the highest landfill gas emission in 2043, primarily methane, along with carbon dioxide and non-metallic organic compounds. The estimated quantities for these gases in 2043 are 5.415E+03, 1.486E+04, and 2.327E+02 metric tons, respectively.Conclusions:
This research highlights the importance of tailored waste management strategies to mitigate environmental impacts and emphasizes the need for efficient gas collection systems. A proposed gas collection system combining vertical wells, horizontal trenches, and under-membrane pipes is discussed to enhance efficiency and reduce environmental risks. The study provides valuable insights into waste management and landfill gas emissions on Kish Island, underscoring the significance of accurate emission prediction and effective management to minimize environmental consequences and optimize the utilization of renewable energy resources.Keywords
Greenhouse Gas Emissions Methane Production Post-landfill Maintenance LandGEM Simulation Model Landfill Management Kish Island
1. Background
Municipal solid waste (MSW) landfills are substantial contributors to greenhouse gas emissions, notably methane, as waste undergoes decomposition (1-3). The assessment of methane gas production from landfills has received considerable attention, with the LandGEM model being widely employed for this purpose. Numerous studies have showcased the model's efficacy in both energy generation and emission control. Fallahizadeh et al. employed LandGEM to estimate methane gas production within an Iranian landfill from 2009 to 2012. Their findings revealed peak production occurring between 2010 and 2012, underscoring the potential of gas collection systems to mitigate environmental impact (4, 5). Chandra and Ganguly utilized the LandGEM model to forecast landfill gas emissions in India for the period from 2015 to 2030. Their investigation uncovered significant opportunities for energy recovery and emission control measures (6). Likewise, Ramprasad et al. quantified emissions from an Indian landfill using the LandGEM model, highlighting promising avenues for renewable energy generation (7).
Furthermore, the variation in methane production across diverse regions has been a focal point of inquiry. Certain researchers examined methane production in multiple locations throughout Iran by integrating waste analysis with the LandGEM model and other methodologies (8-10). The study underscored the significance of waste management strategies aimed at efficiently capturing and utilizing methane gas. Dimishkovska et al. conducted a comparative analysis of emission estimations from a landfill in Kosovo, employing both the Intergovernmental Panel on Climate Change (IPCC) and LandGEM methodologies. Their study emphasized the necessity of accounting for location-specific characteristics to ensure accurate estimations (11). Srivastava and Chakma assessed various models, including LandGEM, to estimate emissions from Indian landfills. Their evaluation determined the first-order decay model to be the most precise in this regard (12).
Although LandGEM is widely used for estimating landfill gas emissions, it is important to consider alternative prediction methods to justify the choice of this model. Other commonly used approaches include the Intergovernmental Panel on Climate Change (IPCC) methodology, first-order decay models, and fuzzy logic-based models. The IPCC methodology provides a standardized approach for estimating emissions based on waste composition and disposal practices, making it suitable for comparative studies between different regions (11, 12). First-order decay models, as demonstrated by Srivastava and Chakma, have been shown to provide accurate estimates for Indian landfills (12). Fuzzy logic-based models, as demonstrated by Mohsen and Abbassi, have shown higher predictive accuracy compared to LandGEM in certain cases (13). Despite these alternatives, LandGEM remains a widely accepted and validated tool for estimating landfill gas emissions, especially when site-specific data are available (4-7, 14-28). In the present study, LandGEM is used for the Kish Island landfill due to its proven track record, ease of use, and availability of the required input data.
Additionally, researchers have examined the potential for energy generation and emissions reduction through landfill gas management. Alam et al. used LandGEM to predict emissions from Pakistani landfills up to the year 2045, showing how effective management can significantly reduce emissions while generating energy (14). Alam et al. utilized both LandGEM and IPCC methods to assess emissions from Iranian landfills, revealing Tehran to have the highest emissions, thus underscoring the need for enhanced waste management practices in the region (14). Similarly, Suryawan and Afifah used both the LandGEM and IPCC methodologies to estimate emissions from an Indonesian landfill, highlighting the significant contribution of landfill gas to total emissions (15).
Although LandGEM has seen extensive use, alternative models have emerged and undergone comparison. Mohsen and Abbassi devised a fuzzy model that demonstrated superior predictive accuracy in estimating landfill emissions in Canada compared to LandGEM. Their findings offer valuable insights for devising effective management strategies (13). Andriani and Atmaja conducted a comprehensive review of waste management practices in Indonesia, estimating a considerable annual energy generation potential of 54,142 MWh from landfill gas, employing both LandGEM and IPCC methodologies. Their analysis underscores the substantial benefits that could be realized through enhanced waste management practices (29).
Numerous studies have investigated the energy potential of landfills across various regions. Osra et al. evaluated the energy potential of a landfill in Saudi Arabia employing LandGEM, revealing a potential gas yield of 83.52 m3 per ton of waste. Their findings underscore the opportunities for energy recovery and enhanced management practices (16). Choudhary et al. utilized both LandGEM and IPCC models to forecast waste emissions and energy potential in India. Their projections indicate a significant potential energy generation of 1387 MW from landfill gas by 2030, achievable through improved management practices (17).
The assessment of emissions from waste management practices in diverse regions has garnered attention. Caetano et al. examined emissions from waste management in Angola, identifying landfills and anaerobic decomposition as primary contributors (18). The study proposed mitigating landfill emissions by implementing sorting, recycling, and treatment procedures. Şentürk and Yıldırım estimated total landfill gas, methane, and carbon dioxide emissions, as well as energy potential, from a landfill in Turkey using LandGEM. Their findings underscored the feasibility of gas collection and emission control measures (19). Furthermore, Arslan and Eren utilized LandGEM to estimate emissions from a Turkish landfill, confirming the model's efficacy in energy estimation (20).
Other studies have delved into the potential for reducing greenhouse gas emissions and recovering energy from waste management practices across various regions. Yaman et al. investigated the reduction in greenhouse gas emissions and energy recovery potential from Saudi Arabian waste using LandGEM under various management scenarios, including composting and waste-to-energy processes (30). Mir et al. utilized both LandGEM and IPCC models to quantify and validate methane emissions from an Indian landfill. Their study underscored the potential of gas collection to mitigate emissions and harness energy (21).
Precise data and coefficients are paramount for dependable estimations and effective energy planning. Ambuludi-Paredes et al. employed a customized LandGEM model to gauge methane potential from an Ecuadorian landfill, highlighting the significance of accurate data and coefficients for reliable estimations and energy strategizing (22). Sadeghi et al. utilized LandGEM to estimate escalating methane emissions from Iranian landfills over time, stressing the crucial role of control and management in safeguarding the environment (23).
Comparative analyses between LandGEM and alternative estimation models have been undertaken. Das and Hazra juxtaposed LandGEM with modified triangular models to gauge emissions and energy potential from an Indian landfill. Their findings underscored the potential of energy generation and enhanced management practices in mitigating emissions (31). Anh et al. utilized both LandGEM and IPCC models to quantify emissions from a Vietnamese landfill, emphasizing the impact of location-specific characteristics. Their study highlighted the potential for renewable energy generation and enhanced management practices (32).
Studies conducted across various regions have investigated the potential for landfill gas and methane production. Usman analyzed municipal waste in Nigeria to assess landfill gas and methane potential utilizing LandGEM. Their findings estimated a substantial potential annual heat energy of 33.78 GWh and electricity of 10.14 GWh, highlighting the economic and social advantages of waste-to-energy practices (24). Koushkinasab et al. utilized LandGEM to estimate emissions from an Iranian landfill, showcasing the potential for energy production while underlining the significance of factoring in emissions in waste management strategies (25).
Additionally, researchers have delved into landfill emissions and the potential for reduction across diverse regions. Pazoki and Ghasemzadeh utilized LandGEM to estimate methane emissions from a Ugandan landfill, proposing waste management strategies, public lifestyle alterations, and educational campaigns as potential avenues for emission reduction (26). Amini et al. used LandGEM to forecast biogas production from Iranian landfills over a 20-year timeframe, uncovering potential for energy generation. Their study recommended enhanced management practices to alleviate environmental impacts (27).
Consistent with these discoveries, Ghasemzade and Pazoki projected a rise in landfill gas emissions in Iran using LandGEM. Their estimations suggested emissions of 32,994 tonnes/year of methane, 8,813 tonnes/year of carbon dioxide, and 24,181 tonnes/year of non-methane organic compounds by 2045 (28). The study underscores the critical importance of implementing effective management strategies to achieve sustainability objectives.
2. Objectives
The present study aims to improve the existing knowledge base by assessing the applicability of the LandGEM model in describing total methane, carbon dioxide, and non-methane organic compounds (NMOC) production per mass of produced solid waste at the Kish Island landfill over 19 years. This research is distinguished by its focus on a specific geographical location, considering that the accurate estimation of greenhouse gas emissions from landfills depends largely on local conditions, such as waste composition, climate, and management. The detailed analysis of the LandGEM model's performance under the conditions of Kish Island will provide valuable insights into developing effective waste management strategies and assessing the potential for energy recovery in the region.
3. Methods
Kish Island, situated in the Bandar Lengeh District of Hormozgan Province, spans an area of 91.5 km² and showcases coral reefs and several smaller islands along its coast. With a population of approximately 40,000, the island features numerous shopping centers, tourist attractions, and hotels, and holds recognition as a free trade zone in Iran. Each year, Kish Island welcomes around one million tourists. The island experiences a semi-equatorial arid climate, characterized by a mean annual precipitation of 145 mm, with 54% occurring in winter, 28% in fall, and 14% in summer. The average annual temperature stands at 26.6°C, and humidity remains around 60% throughout most of the year. The weather is mild, ranging from 18-25°C, between October and April on Kish Island (33, 34).
The landfill site on Kish Island is situated in the southeast, covering an area of 9,500 square meters. Figure 1 provides the geographical location of Kish Island, including the waste disposal site. Based on available statistics, the per capita daily waste generation on Kish Island amounts to 2.38 kg. Table 1 details the physical composition of municipal solid waste on Kish Island.
Geographical location of Kish Island
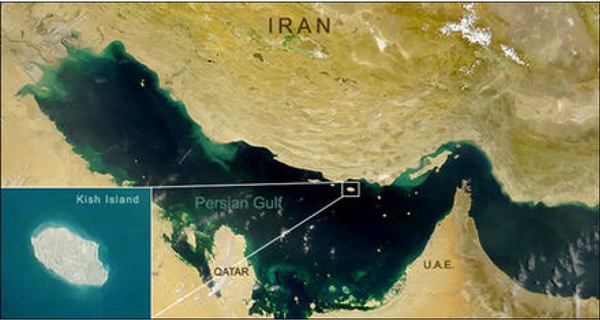
Physical Composition of Municipal Solid Waste in Kish Island (%)
Putrescible | Wood | Paper | Cardboard | Glass | Steel | Plastic | PET | Textile | Leather | Dangerous waste | Green | Other |
---|---|---|---|---|---|---|---|---|---|---|---|---|
36 | 3.1 | 8.2 | 6.5 | 1.6 | 0.75 | 14 | 3.1 | 14 | 0 | 0.11 | 11 | 1.64 |
Kish population is 40186 in 2023. The population growth rate of Kish Island has exhibited a remarkable degree of stability in recent years. A noteworthy factor contributing to this growth is the influx of approximately 1 million tourists in 2022. To estimate the approximate population residing in Kish, we can divide the annual tourist count by the number of days in a year and add it to the existing population of Kish residents. Moreover, it is important to acknowledge that the future expansion of hotels in Kish, projected to increase from 50 to 100 over the next few years, is expected to result in an annual growth rate of approximately 8% in tourist arrivals. Based on the aforementioned considerations, the anticipated population of Kish Island in the next two decades can be estimated in Table 2.
Population, Total Generated Waste per Day and Total Putrescible Waste Generated per day in Kish Island During 2023 - 2042
Year | Population | Total Waste Generated (kg/day) | Total Putrescible Waste Generated (kg/day) |
---|---|---|---|
2023 | 43145 | 107863 | 38831 |
2024 | 46104 | 115261 | 41494 |
2025 | 49064 | 122659 | 44157 |
2026 | 52023 | 130057 | 46821 |
2027 | 54982 | 137455 | 49484 |
2028 | 57941 | 144853 | 52147 |
2029 | 60900 | 152251 | 54810 |
2030 | 63860 | 159649 | 57474 |
2031 | 66819 | 167047 | 60137 |
2032 | 69778 | 174445 | 62800 |
2033 | 72737 | 181843 | 65463 |
2034 | 75696 | 189241 | 68127 |
2035 | 78656 | 196639 | 70790 |
2036 | 81615 | 204037 | 73453 |
2037 | 84574 | 211435 | 76117 |
2038 | 87533 | 218833 | 78780 |
2039 | 90492 | 226231 | 81443 |
2040 | 93452 | 233629 | 84106 |
2041 | 96411 | 241027 | 86770 |
2042 | 99370 | 248425 | 89433 |
According to extensive study and taking into account the distinctive nature of Kish Island as a prominent tourist destination, it has been observed that the average quantity of waste generated per individual on a daily basis in this island amounts to approximately 2.5 kilograms. Table 2 illustrates the population, total generated waste per day and total putrescible waste generated per day in Kish Island during 2023 - 2042.
The Landfill Gas Emissions Model (LandGEM) is a widely used tool developed by the United States Environmental Protection Agency (US EPA) to estimate landfill gas emissions. LandGEM is based on a first-order decay equation that considers the history of waste disposal, landfill characteristics, and climatic conditions to predict the generation of methane, carbon dioxide, and other landfill gases over time (35). Features of the model include the ability to input site-specific data, such as the waste acceptance rate, landfill opening and closure year, and values for methane generation potential (L0) and rate constant (k). LandGEM also provides default parameters derived from empirical data from landfills in the United States that can be used when site-specific data are not available (36).
LandGEM is a Microsoft Excel-based software tool designed to gauge gas generation at landfill sites. It estimates various gases, including methane, carbon dioxide, nonmetallic organic compounds, and pollutants. This software is adaptable, allowing users to input real-time data from landfill sites or opt for default information available in two categories: Default CAA information and Full list default information. The Default CAA information is derived from US federal legislation related to municipal waste disposal, aiding in assessing the need for implementing air pollution control systems at landfills.
The LandGEM model has been extensively validated and applied in numerous studies worldwide to predict greenhouse gas emissions and methane production from landfills. For instance, Amini et al. used LandGEM to estimate methane emissions from landfills in Tehran, Iran, and found that the model's predictions aligned well with field measurements (37). Similarly, Kalantarifard and Yang used LandGEM to assess the potential for energy recovery from landfill gas in Malaysia and confirmed the model's reliability in estimating methane generation (38). Karanjekar et al. conducted a comprehensive review of various landfill gas emission models and concluded that LandGEM is a robust and widely accepted tool for estimating methane emissions from landfills, especially when site-specific data are available (39).
Utilizing the first-order solid-state decay rate equation, LandGEM offers a streamlined approach to estimating waste disposal. It relies on empirical landfill data, predominantly sourced from the United States. Operating as a screening tool, LandGEM enhances the precision of input data estimation. However, it's essential to acknowledge that the accuracy of gas emission estimates may be influenced by limited information concerning landfill quantity, composition, and processes. Changes in landfill conditions, such as elevated moisture content due to leachate reintroduction, can result in heightened gas production.
Equation (1) represents the fundamental first-order decay equation employed within LandGEM for estimation purposes.
The mathematical representation of the first-order decay equation used in this study is as follows:
Equation 1:
In the given equation, QCH4 represents the estimated annual methane production. The variable i denotes the incremental increase in the studied years, while n represents the difference between the predicted year and the initial year of waste disposal. The parameter j is assigned a value of 0.1, representing the incremental increase within the observed years. The methane production rate (k) is measured in units of year-1. L0 denotes the methane production potential per unit mass (m3/Mg) and is influenced by the type and composition of landfill waste. Mi represents the waste mass in the ith year, measured in Mg or tons. Lastly, tij signifies the age of the jth section of waste mass Mi in decimal years.
The validity of the LandGEM method for predicting greenhouse gas emissions and methane production in this study is supported by several previous studies conducted under similar climatic conditions and waste compositions. Farzadkia et al. used LandGEM to estimate methane emissions from a landfill in Isfahan, Iran, which has comparable climatic conditions to Kish Island. Their results demonstrate the applicability and reliability of the model in predicting methane formation under semi-arid conditions (40). Additionally, Mohseni et al. used LandGEM to assess the potential for landfill gas recovery in Tehran, Shiraz, Mashhad, Esfahan, and Karaj, Iran, and found that the model's predictions were consistent with the observed waste composition and decomposition rates in the region (41). These studies, along with the extensive validation of LandGEM in various contexts worldwide, confirm the validity of the method for estimating greenhouse gas emissions and methane production from the Kish Island landfill.
The methane generation rate (k) holds significant importance in methane production, as higher rates signify faster decomposition. This rate is influenced by several factors, including waste moisture, microbial activity responsible for decomposing waste into methane and carbon dioxide, waste pH, and waste temperature. In this study, both the Clean Air Act (CAA) and LandGEM (version 3.02) typically assign a conventional landfill gas value of 0.05. The methane production potential (L0) is determined solely by the type and composition of the landfill waste. Following the CAA's recommendations for arid and semi-arid regions, the suggested methane production potential is 170 cubic meters per ton. Therefore, in this study, the default value for L0 in the LandGEM software for arid regions is set to 170 (Table 3).
Parameters and Specifications of the Landfills Studied in LandGEM Model
Parameters | Values |
---|---|
Beginning year of waste disposal | 2023 |
Year of landfill closure | 2042 |
Does the year of landfill closure require a computing model? No | No |
Landfill capacity | Not available (ton) |
Methane production rate (k) | 0.05; 1(y) |
Methane production potential (L0) | 170; m3/ton |
Concentration of non-methane organic compounds | 4000; ppmv |
Methane content | 50; Volume rate (%) |
4. Results
It should be mentioned that the statistics related to Kish Island are different compared to other regions of Iran due to the fact that this island is a tourist destination. During 2023 to 2042, a total of 1,282,637 tons of waste is expected to be disposed of in the Kish landfill.
Considering the environmental repercussions of waste disposal, the LandGEM model is indispensable in forecasting landfill gas emissions. The principal gases emitted during the decomposition process are methane, carbon dioxide, and hydrogen. Notably, methane constitutes approximately 60% of landfill gases, with the remaining portion primarily comprising carbon dioxide (42, 43). Projections based on the LandGEM model suggest that the highest release of landfill gas will occur in 2042, the year following the cessation of waste disposal at the landfill.
The generated waste in Kish is expected to increase from 107 tons per day in 2023 to 248 tons per day in 2042. These figures underscore the mounting waste management challenges faced by Kish due to tourist population growth and the expansion of the tourism and commercial sectors. To effectively mitigate the environmental impact, it is crucial to develop tailored waste management strategies.
An intriguing observation arises concerning the composition of waste, notably food waste, across different regions. In Iran, including Kish, food waste forms a substantial proportion of the total waste generated, likely influenced by the prevailing food culture and preferences in the country. However, comparative analysis reveals that other nations exhibit a lower percentage of food waste in their waste streams. For instance, a study conducted by Kalantarifard and Yang suggests that food waste constitutes 30% of waste in Malaysia (44). Statistics indicate that within the waste stream of the Kish landfill, food waste alone comprises approximately 36% of the total waste composition.
To assess the impact of waste management practices on methane production, annual methane production figures are essential. Notably, there is an upward trend in methane production over time. In 2043, one year after landfill closure, the methane emission volume is projected to be 7,378,091.51 m³/year. Additionally, the carbon dioxide and NMOC emission volumes in 2043 are projected to be 7,378,091.51 m³/year and 59,024.73 m³/year, respectively. This is due to variations in annual putrescible waste production, ranging from 14,173 tons to 32,643 tons per year, as the landfill transitioned from an open landfill to a closed landfill. Figure 2 provides insights into the volume of methane, carbon dioxide, and NMOC gases produced over time.
Total volume emission of methane, carbon dioxide and non-methane organic compounds (NMOC) over time
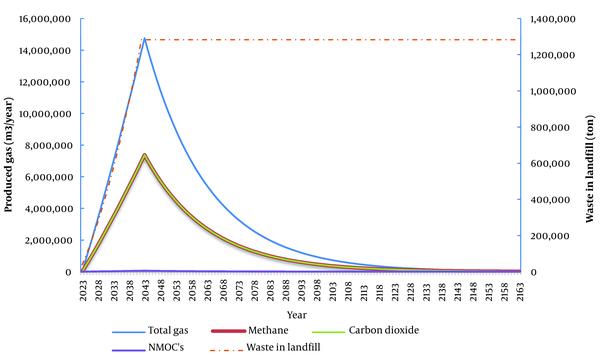
The weight of the produced gases is shown in Figure 3. The weight of CO2 is greater than that of CH4, with the weight ratio of carbon dioxide to methane being approximately 2.74. This indicates that methane is positioned above carbon dioxide in the landfill, resulting in higher volatility. According to the predicted data, the highest weights of carbon dioxide and methane produced will be 13,505.59 Mg/year and 4,922.28 Mg/year, respectively, in 2043.
Total weight emission of methane, carbon dioxide and non-methane organic compounds (NMOC) over time
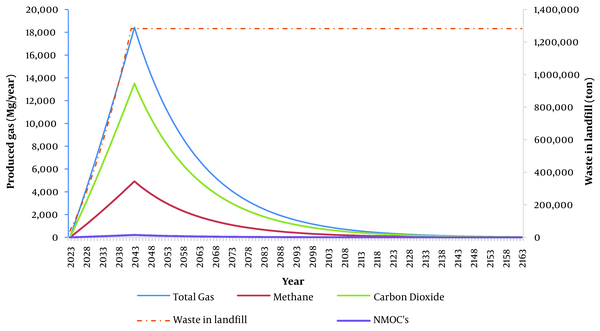
non-methane organic compounds (non-methane organic compounds) gases represent a category of chemical compounds characterized by similar atmospheric behavior despite having distinct chemical structures. These gases play a role in the generation of tropospheric ozone. The quantities of NMOC gases generated are illustrated in Figures 2, and 3, but their cumulative volume is significantly smaller than that of methane and carbon dioxide, constituting approximately 1%. Consequently, the annual NMOC production chart for the Kish Island landfill is presented independently in Figure 4.
Total volume and weight emission of non-methane organic compounds (NMOC’s) over time
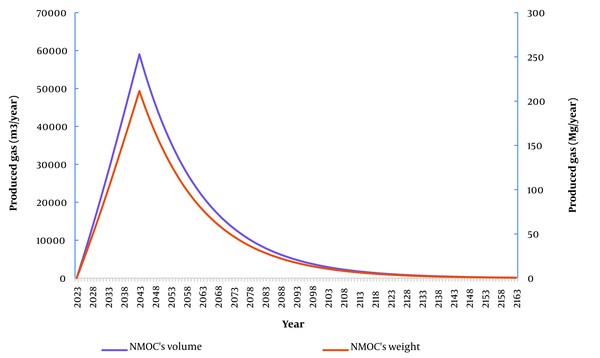
The significance of non-methane organic gases lies in their impact on human health, even though their concentrations are lower. Notably, these gases can have more detrimental effects on well-being, with several compounds identified as primary contributors to cancer. Table 4 provides a comprehensive list of approximately 46 non-methane organic compounds produced in the Kish Island landfill in 2043, as estimated by LandGEM.
Non-methane Organic Compounds Gases Produced at Kish Island Landfill in 2043 (LandGEM Estimation)
Gas/Emission | Emission Rate | |
---|---|---|
(m3/y) | Ton/y | |
Total landfill gas | 1.476E + 07 | 2.027E + 04 |
Methane | 7.378E + 06 | 5.415E + 03 |
Carbon dioxide | 7.378E + 06 | 1.486E + 04 |
NMOC | 5.902E + 04 | 2.327E + 02 |
1,1,1-Trichloroethane (methyl chloroform) - HAP | 7.083E + 00 | 4.323E - 02 |
1,1,2,2-Tetrachloroethane - HAP/VOC | 1.623E + 01 | 1.247E - 01 |
1,1-Dichloroethane (ethylidene dichloride) - HAP/VOC | 3.541E + 01 | 1.604E - 01 |
1,1-Dichloroethene (vinylidene chloride) - HAP/VOC | 2.951E + 00 | 1.309E - 02 |
1,2-Dichloroethane (ethylene dichloride)-HAP/VOC | 6.050E + 00 | 2.739E - 02 |
1,2-Dichloropropane (propylene dichloride)-HAP/VOC | 2.656E + 00 | 1.373E - 02 |
2-Propanol (isopropyl alcohol)-VOC | 7.378E + 02 | 2.029E + 00 |
Acetone | 1.033E + 02 | 2.745E - 01 |
Acrylonitrile-HAP/VOC | 9.296E + 01 | 2.257E - 01 |
Benzene-No or Unknown Co-disposal-HAP/VOC | 2.804E + 01 | 1.002E - 01 |
Benzene-Co-disposal - HAP/VOC | 1.623E + 02 | 5.801E - 01 |
Bromodichloromethane - VOC | 4.574E + 01 | 3.429E - 01 |
Butane-VOC | 7.378E + 01 | 1.962E - 01 |
Carbon disulfide - HAP/VOC | 8.559E + 00 | 2.981E - 02 |
Carbon monoxide | 2.066E + 03 | 2.647E + 00 |
Carbon tetrachloride-HAP/VOC | 5.902E-02 | 4.154E - 04 |
Carbonyl sulfide-HAP/VOC | 7.231E + 00 | 1.987E - 02 |
Chlorobenzene-HAP/VOC | 3.689E + 00 | 1.900E - 02 |
Chlorodifluoromethane | 1.918E + 01 | 7.589E - 02 |
Chloroethane (ethyl chloride)-HAP/VOC | 1.918E + 01 | 5.663E - 02 |
Chloroform-HAP/VOC | 4.427E-01 | 2.418E - 03 |
Chloromethane-VOC | 1.771E + 01 | 4.090E - 02 |
Dichlorobenzene-(HAP for para isomer/VOC) | 3.099E + 00 | 2.084E - 02 |
Dichlorodifluoromethane | 2.361E + 02 | 1.306E + 00 |
Dichlorofluoromethane-VOC | 3.837E + 01 | 1.807E - 01 |
Dichloromethane (methylene chloride)-HAP | 2.066E + 02 | 8.028E - 01 |
Dimethyl sulfide (methyl sulfide)-VOC | 1.151E + 02 | 3.272E - 01 |
Ethane | 1.313E + 04 | 1.807E + 01 |
Ethanol-VOC | 3.984E + 02 | 8.400E - 01 |
Ethyl mercaptan (ethanethiol)-VOC | 3.394E + 01 | 9.647E - 02 |
Ethylbenzene-HAP/VOC | 6.788E + 01 | 3.297E - 01 |
Ethylene dibromide-HAP/VOC | 1.476E-02 | 1.268E - 04 |
Fluorotrichloromethane-VOC | 1.121E + 01 | 7.049E - 02 |
Hexane-HAP/VOC | 9.739E + 01 | 3.840E - 01 |
Hydrogen sulfide | 5.312E + 02 | 8.283E - 01 |
Mercury (total)-HAP | 4.279E-03 | 3.928E - 05 |
Methyl ethyl ketone-HAP/VOC | 1.048E + 02 | 3.457E - 01 |
Methyl isobutyl ketone-HAP/VOC | 2.804E + 01 | 1.285E - 01 |
Methyl mercaptan-VOC | 3.689E + 01 | 8.120E - 02 |
Pentane-VOC | 4.870E + 01 | 1.607E - 01 |
Perchloroethylene (tetrachloroethylene)-HAP | 5.460E + 01 | 4.142E - 01 |
Propane-VOC | 1.623E + 02 | 3.274E - 01 |
t-1,2-Dichloroethene-VOC | 4.132E + 01 | 1.833E - 01 |
Toluene - No or Unknown Co-disposal-HAP/VOC | 5.755E + 02 | 2.426E + 00 |
Toluene-Co-disposal-HAP/VOC | 2.509E + 03 | 1.057E + 01 |
Trichloroethylene (trichloroethene)-HAP/VOC | 4.132E + 01 | 2.484E - 01 |
Vinyl chloride-HAP/VOC | 1.077E + 02 | 3.080E - 01 |
Xylenes-HAP/VOC | 1.771E + 02 | 8.601E - 01 |
5. Discussion
Waste generation poses a significant global concern, especially in developing nations. According to the United Nations, developing countries typically produce between 500 to 900 grams of waste per person per day. In Iran, the average is 850 grams per capita per day (45-47). Waste generation in urban and rural areas in Iran exhibits variation due to the distinct lifestyles of their residents. Comprehensive studies conducted by Vahidi et al. have delved into waste patterns in rural areas across three provinces of Iran. Their findings revealed that rural areas in Yazd, Chahar Mahal and Bakhtiari, and Isfahan provinces generate 507, 293, and 497 grams of waste per capita per day, respectively. Furthermore, their research indicates that a significant portion of waste in rural areas of Chaharmahaal and Bakhtiari (39.3%) and Yazd provinces (41%) is classified as putrescible waste (48). This underscores the necessity for effective waste management strategies in these regions.
In the urban areas of the country, the statistics differ from rural areas due to variances in residents' lifestyles and industrial activities. For instance, studies by Ghasemzadeh indicate that by 2045, 3,324,274 tons of waste will be disposed of in municipal landfills in Jiroft (28). Comparable studies carried out by Sadeghi et al. in Sanandaj provide insights into methane production rates across various regions (49). Their study suggests that methane production in Kish was relatively lower compared to other regions, attributable to its smaller population and lower waste generation. Table 3 illustrates the total emissions in 2043.
This study highlights the significant potential for methane production and subsequent energy recovery from the Kish Island landfill. The LandGEM model's estimates of methane, carbon dioxide, and NMOC emissions over a 19-year period provide valuable insight into the landfill's contributions to greenhouse gases and opportunities to reduce them through effective waste management practices. The observed spike in methane production underscores the need for gas collection systems to capture and utilize this resource and minimize its environmental impact. These results are critical for developing sustainable waste management strategies on Kish Island and for integrating landfill gas utilization into the regional energy mix.
The methane production rates and emission estimates from this study are consistent with similar studies in other parts of Iran and beyond. For example, Fallahizadeh et al. (4) and Mostafa Hatami et al. (42) reported comparable methane production patterns in Iranian landfills, with peak production occurring within a similar timeframe. The lower methane production found by Mostafa Hatami et al. (42) on Kish Island compared to other regions can be attributed to the island's lower population and waste generation. This highlights the impact of site-specific factors on landfill gas emissions and the need for tailored waste management strategies. This study contributes to the growing knowledge of landfill gas emissions in Iran and provides a basis for further research and informed decision-making in predicting and managing landfill gas emissions.
This study illuminated the importance of waste management and landfill gas emissions on Kish Island. The utilization of the LandGEM model proved effective in estimating methane gas emissions from landfills, facilitating accurate predictions of methane production rates over time. The study emphasized the importance of implementing methane gas collection systems in landfills to effectively address gas accumulation, mitigate potential explosion hazards, and harness methane as a viable renewable energy source. Additionally, it highlighted the significant contribution of landfill gas to greenhouse gas emissions, particularly methane and carbon dioxide resulting from biological processes within landfills. Recognizing methane as a valuable energy resource further underscores the need for accurately predicting and effectively managing landfill gas emissions to minimize environmental consequences and unlock the full energy potential inherent in these waste disposal sites.
To improve gas extraction on Kish Island, several constraints must be addressed. Although the combined approach of pipes under the membrane and horizontal trenches has shown some efficiency gains, its performance has been hampered by problems such as burning of liquid gas, cracks in the cover, and high levels of seepage. To overcome these obstacles, a solution is proposed that includes vertical wells, trenches, and sub-membrane pipes along with a high-density polyethylene (HDPE) cover. This cost-effective system improves drainage and gas capture, with more than 70% of the gas being captured by the pipes under the membrane. To ensure long-term success, operational optimization and extensive testing are essential. Implementing this solution will improve gas management, reduce environmental impact, and enhance resource utilization.
The main significance of this study lies in the use of a predictive model for the management of landfill gas emissions. As the LandGEM model has been shown to be effective in estimating methane production and resulting emissions from the Kish Island landfill, it will be of great importance to decision-makers and waste management authorities in assessing the environmental impact of landfills and developing appropriate mitigation strategies. The study emphasizes the importance of developing efficient landfill gas collection systems and utilizing energy recovery opportunities.
In the future, further studies should be conducted to calibrate the parameters of the LandGEM model for local conditions, investigate the possibilities of landfill gas recovery and its integration into the energy infrastructure, and measure the economic and social benefits of sustainable waste management practices on Kish Island and elsewhere.
Acknowledgements
References
-
1.
Padash A, Vahidi H, Fattahi R, Nematollahi H. Analyzing and evaluating industrial ecology development model in iran using FAHP-DPSIR. Inter J Environmental Res. 2021;15(4):615-29. https://doi.org/10.1007/s41742-021-00335-z.
-
2.
Sadeghi B, Sodagari M, Nematollahi H, Alikhani H. FMEA and AHP methods in managing environmental risks in landfills: A case study of kahrizak, iran. Environmental Energy Economic Res. 2021;5(2):1-15. https://doi.org/10.22097/eeer.2020.253735.1172.
-
3.
Sekhavatjou MS, Mehdipour A, Takdastan A, Hosseini Alhashemi A. CH4 and total GHGs emission from urban landfills in southwest Iran. J Integrative Environmental Sci. 2012;9(sup1):217-23. https://doi.org/10.1080/1943815X.2012.715584.
-
4.
Fallahizadeh S, Rahmatinia M, Mohammadi Z, Vaezzadeh M, Tajamiri A, Soleimani H. Estimation of methane gas by LandGEM model from Yasuj municipal solid waste landfill, Iran. MethodsX. 2019;6:391-8. [PubMed ID: 30906697]. [PubMed Central ID: PMC6411487]. https://doi.org/10.1016/j.mex.2019.02.013.
-
5.
Farokhi M, Kouti M, Mousavi GR, Takdastan A. [The study on biodegradability enhancement of landfill leachate by Fenton oxidation]. Iran J Health Environment. 2009;2(2):114-23. Persian.
-
6.
Chandra S, Ganguly R. Assessment of landfill gases by LandGEM and energy recovery potential from municipal solid waste of Kanpur city, India. Heliyon. 2023;9(4). e15187. [PubMed ID: 37089347]. [PubMed Central ID: PMC10113863]. https://doi.org/10.1016/j.heliyon.2023.e15187.
-
7.
Ramprasad C, Teja HC, Gowtham V, Vikas V. Quantification of landfill gas emissions and energy production potential in Tirupati Municipal solid waste disposal site by LandGEM mathematical model. MethodsX. 2022;9:101869. [PubMed ID: 36204474]. [PubMed Central ID: PMC9529986]. https://doi.org/10.1016/j.mex.2022.101869.
-
8.
Golbaz S, Mahvi AH, Emamjomeh MM, Baghani AN. Formulating landfill gas emissions model for forecasting methane generation from waste under Iranian scenario. Inter J Environment Waste Manage. 2021;28(3):298-316. https://doi.org/10.1504/IJEWM.2021.118367.
-
9.
Ghasemzadeh R, Abdoli MA, Bozorg Haddad O, Pazoki M. Performance evaluation of hydrothermal carbonation treatment to biogas production from anaerobic digestion of organic Waste. J Water Wastewater Sci Engineering. 2022;7(2):14-22. https://doi.org/10.22112/JWWSE.2021.297114.1281.
-
10.
Ghasemzadeh R, Abdoli MA, Bozorg-Haddad O, Pazoki M. Optimizing the effect of hydrochar on anaerobic digestion of organic fraction municipal solid waste for biogas and methane production. J Environ Health Sci Eng. 2022;20(1):29-39. [PubMed ID: 35669808]. [PubMed Central ID: PMC9163232]. https://doi.org/10.1007/s40201-021-00751-5.
-
11.
Dimishkovska B, Berisha A, Lisichkov K. Estimation of methane emissions from Mirash municipal solid waste sanitary landfill, differences between IPPC 2006 and LandGEM method. J Ecological Engineering. 2019;20(5). https://doi.org/10.12911/22998993/105332.
-
12.
Srivastava AN, Chakma S. Quantification of landfill gas generation and energy recovery estimation from the municipal solid waste landfill sites of Delhi, India. Energy Sources, Part A: Recovery, Utilization, Environmental Effects. 2020:1-14.
-
13.
Mohsen RA, Abbassi B. Prediction of greenhouse gas emissions from Ontario's solid waste landfills using fuzzy logic based model. Waste Manag. 2020;102:743-50. [PubMed ID: 31805447]. https://doi.org/10.1016/j.wasman.2019.11.035.
-
14.
Alam A, Chaudhry MN, Ahmad SR, Ullah R, Batool SA, Butt TE, et al. Application of LandGEM mathematical model for the estimation of gas emissions from contaminated sites. A case study of a dumping site in Lahore, Pakistan. Environment Protection Engineering. 2022;48(1). https://doi.org/10.37190/epe220105.
-
15.
Suryawan IWK, Afifah AS. Estimation of Green House Gas (GHG) emission at Telaga Punggur landfill using triangular, LandGEM, and IPCC methods. J Physics: Conference Series. IOP Publishing; 2020. 12001 p.
-
16.
Osra FA, Ozcan HK, Alzahrani JS, Alsoufi MS. Municipal solid waste characterization and landfill gas generation in kakia landfill, makkah. Sustainability. 2021;13(3):1462. https://doi.org/10.3390/su13031462.
-
17.
Choudhary A, Kumar A, Kumar S. National municipal solid waste energy and global warming potential inventory: India. J Hazardous, Toxic, Radioactive Waste. 2020;24(4):6020002. https://doi.org/10.1061/(ASCE)HZ.2153-5515.0000521.
-
18.
Caetano M, Góis J, Leitão A. Challenges and perspectives of greenhouse gases emissions from municipal solid waste management in Angola. Energy Reports. 2020;6:364-9. https://doi.org/10.1016/j.egyr.2019.08.074.
-
19.
Şentürk İ, Yıldırım B. A study on estimating of the landfill gas potential from solid waste storage area in Sivas, Turkey. Scientific J Mehmet Akif Ersoy Univ. 2020;3(2):63-76.
-
20.
Arslan Z, Eren Z. Modelling and assessment of landfill gas generation at Erzurum municipal landfill site by LandGEM. Inter J Agriculture Environment Food Sci. 2022;6(3):378-88. https://doi.org/10.31015/jaefs.2022.3.6.
-
21.
Mir AA, Mushtaq J, Dar AQ, Patel M. A quantitative investigation of methane gas and solid waste management in mountainous Srinagar city-A case study. J Material Cycles Waste Manageme. 2023;25(1):535-49. https://doi.org/10.1007/s10163-022-01516-4.
-
22.
Ambuludi-Paredes RR, Carvajal-Padilla VP, Diéguez Santana K. Estimation of methane gas by LandGEM model from Patate-Pelileo municipal solid waste landfill, Tungurahua, Ecuador. Tungurahua, Ecuador. 2021;35(1):67-78. https://doi.org/10.18845/tm.v35i1.5413.
-
23.
Sadeghi S, Shahmoradi B, Azadi NA, Karami K, Ghaslani M, Karami Cheshmeh Zangi M, et al. [A prospective study on methane gas emission from Saqqez solid waste landfills]. J Res Environmental Health. 2020;6(2):173-81. Persian.
-
24.
Usman AM. An estimation of bio-methane and energy project potentials of municipal solid waste using landfill gas emission and cost models. Frontiers in Engineering and Built Environment. 2022;2(4):233-45. https://doi.org/10.1108/FEBE-06-2022-0021.
-
25.
Koushkinasab F, Ghaderpoori M, Jafari A. [Estimation of gas emission from municipal solid waste landfill in Kuhdasht city using LandGEM software]. Yafteh. 2020;22(3). Persian.
-
26.
Pazoki M, Ghasemzadeh R. Municipal landfill leachate management. Springer; 2020. https://doi.org/10.1007/978-3-030-50212-6.
-
27.
Amini E, Nematollahi H, Moradi Banestani N. Estimation and modeling of biogas production in rural small landfills (Case study: Chaharmahaal and Bakhtiari and Yazd rural areas). Environmental Energy Economic Res. 2017;1(4):383-92. https://doi.org/10.22097/eeer.2018.122498.1023.
-
28.
Ghasemzade R, Pazoki M. Estimation and modeling of gas emissions in municipal landfill (Case study: Landfill of Jiroft City). Pollution. 2017;3(4):689-700. https://doi.org/10.22059/POLL.2017.62783.
-
29.
Andriani D, Atmaja TD. The potentials of landfill gas production: A review on municipal solid waste management in Indonesia. J Material Cycles Waste Manage. 2019;21:1572-86. https://doi.org/10.1007/s10163-019-00895-5.
-
30.
Yaman C, Anil I, Alagha O. Potential for greenhouse gas reduction and energy recovery from MSW through different waste management technologies. J Cleaner Production. 2020;264:121432. https://doi.org/10.1016/j.jclepro.2020.121432.
-
31.
Das B, Hazra T. Quantification of landfill gas emission and energy recovery potential: A comparative assessment of LandGEM and MTM Model for Kolkata. Spatial Modeling Assessment Environmental Contaminants: Risk Assessment Remediation. 2021:55-67. [PubMed Central ID: PMC8637061]. https://doi.org/10.1007/978-3-030-63422-3_4.
-
32.
Anh LH, Thanh Truc NT, Tuyen NTK, Bang HQ, Son NP, Schneider P, et al. Site-specific determination of methane generation potential and estimation of landfill gas emissions from municipal solid waste landfill: A case study in Nam Binh Duong, Vietnam. Biomass Conversion Biorefinery. 2021:1-12. https://doi.org/10.1007/s13399-020-01192-0.
-
33.
Fazelpour F, Soltani N, Rosen MA. Feasibility of satisfying electrical energy needs with hybrid systems for a medium-size hotel on Kish Island, Iran. Energy. 2014;73:856-65. https://doi.org/10.1016/j.energy.2014.06.097.
-
34.
Ataie‐Ashtiani B, Rajabi MM, Ketabchi H. Inverse modelling for freshwater lens in small islands: Kish Island, Persian Gulf. Hydrological Processes. 2013;27(19):2759-73. https://doi.org/10.1002/hyp.9411.
-
35.
Model LGE. Version 3.02 user’s guide. US Environmental Protection Agency: Washington, DC. 2005.
-
36.
Bouyakhsass R, Rifi SK, Madinzi A, Digua K, Anouzla A, Abrouki Y, et al. Assessing leachate and landfill biogas generation: Key to sustainable Waste Solutions. A review of landfill leachate: Characterization leachate environment impacts and sustainable treatment methods. Springer; 2024. p. 203-14. https://doi.org/10.1007/978-3-031-55513-8_10.
-
37.
Amini HR, Reinhart DR, Mackie KR. Determination of first-order landfill gas modeling parameters and uncertainties. Waste Manag. 2012;32(2):305-16. [PubMed ID: 22000722]. https://doi.org/10.1016/j.wasman.2011.09.021.
-
38.
Kalantarifard A, Yang GS. Energy potential from municipal solid waste in Tanjung Langsat landfill, Johor, Malaysia. Int J Eng Sci Technol. 2011;3(12):8560-8.
-
39.
Karanjekar RV, Bhatt A, Altouqui S, Jangikhatoonabad N, Durai V, Sattler ML, et al. Estimating methane emissions from landfills based on rainfall, ambient temperature, and waste composition: The CLEEN model. Waste Manag. 2015;46:389-98. [PubMed ID: 26346020]. https://doi.org/10.1016/j.wasman.2015.07.030.
-
40.
Farzadkia M, Jorfi S, Akbari H, Ghasemi M. Evaluation of dry solid waste recycling from municipal solid waste: Case of Mashhad city, Iran. Waste Manag Res. 2012;30(1):106-12. [PubMed ID: 21406505]. https://doi.org/10.1177/0734242X10395659.
-
41.
Mohseni N, Omrani GA, Harati SAN. [Potential prediction of Methane production from landfill in Iranian metropolises (Tehran, Shiraz, Mashhad, Esfahan, Karaj)]. Sustainability, Develop Environment. 2020;1(2):35-49. Persian.
-
42.
Mostafa Hatami A, Sabour MR, Haj Babaei MR, Nematollahi H. Global trends of VOSviewer research, emphasizing Environment and Energy areas: A bibliometric analysis during 2000-2020. Environmental Energy Economic Res. 2022;6(1):1-11. https://doi.org/10.22097/EEER.2021.301784.1216.
-
43.
Maleki Delarestaghi R, Ghasemzadeh R, Mirani M, Yaghoubzadeh P. [The comparison between different waste management methods of Tabas city with life cycle assessment assessment]. J Environmental Sci Studies. 2018;3(3):782-93. Persian.
-
44.
Kalantarifard A, Yang GS. Estimation of methane production by LANDGEM simulation model from Tanjung Langsat municipal solid waste landfill, Malaysia. Inter J Sci Technol. 2012;1(9):481-7.
-
45.
Vahidi H, Hoveidi H, Khoie JK, Nematollahi H, Heydari R. Analyzing material flow in Alborz industrial estate, Ghazvin, Iran. J Material Cycles Waste Manage. 2018;20:450-60. https://doi.org/10.1007/s10163-017-0601-9.
-
46.
Pazoki M, Delarestaghi RM, Rezvanian MR, Ghasemzade R, Dalaei P. Gas production potential in the landfill of Tehran by landfill methane outreach program. Jundishapur J Health Sci. 2015;7(4). https://doi.org/10.17795/jjhs-29679.
-
47.
Amini E, Baniasadi M, Vahidi H, Nematollahi H, Khatami M, Amandadi M, et al. Affecting factors of knowledge-based companies using fuzzy AHP model, case study Tehran University Enterprise Park. J Knowledge Economy. 2020;11(2):574-92. https://doi.org/10.1007/s13132-018-0554-9.
-
48.
Vahidi H, Nematollahi H, Padash A, Sadeghi B, RiyaziNejad M. Comparison of rural solid waste management in two central provinces of Iran. Environmental energy and economic research. 2017;1(2):195-206. https://doi.org/10.22097/eeer.2017.47246.
-
49.
Sadeghi S, Shahmoradi B, Maleki A. Estimating methane gas generation rate from Sanandaj City Landfill using LandGEM software. Res J Environmental Sci. 2015;9(6):280. https://doi.org/10.3923/rjes.2015.280.288.