Abstract
Background:
Existence of fluoride in drinking water above the permissible level causes human skeletal fluorosis.Objectives:
Electrocoagulation by iron and aluminum electrodes was proposed for removing fluoride from drinking water.Materials and Methods:
Effects of different operating conditions such as treatment time, initial pH, applied voltage, type and number of electrodes, the spaces between aluminum and iron electrodes, and energy consumption during electrocoagulation were investigated in the batch reactor. Variable concentrations of fluoride solution were prepared by mixing proper amounts of sodium fluoride with deionized water.Results:
Experimental results showed that aluminum electrode is more effective in fluoride removal than iron, as in 40 minutes and initial pH of 7.5 at 20 V, the fluoride removal process reached to 97.86%. The final recommendable limit of fluoride (1.5 mg/L) was obtained in 10 minutes at 20 V with the aluminum electrode.Conclusions:
In electrocoagulation with iron and aluminum electrodes, increase of voltage, number of electrodes and reaction time as well as decrease of the spaces between electrodes, enhanced the fluoride removal efficiency from drinking water. In addition the effect of pH and initial concentration of fluoride varied with types of electrodes.Keywords
1. Background
Inorganic constituents that might be found in natural waters or contaminated-source waters are turning into major public health problems of drinking water. Presence of fluoride as an inorganic ion above the permissible limit in drinking water is a public health problem (1). The suitable level of fluoride in drinking water specified by the World Health Organization (WHO) is 1.5 mg/L (2-4). Different techniques like alum coagulation, bone char or calcite, (5) adsorption, membrane separation, ion-exchange, hybrid techniques, and electrocoagulation (EC) have been applied for removal of fluoride from drinking water (3, 6). The adsorption process using different adsorbents such as trimetal oxide (7), waste carbon slurry (8) and many low-cost materials were investigated for removal of fluoride from the aqueous medium. Membrane separation techniques were investigated for effective separation of fluoride, using electrodialysis (9), Donnan dialysis (10), nanofiltration (11), and anion-exchange membrane (12). Garmes et al. (13) performed defluoridation of ground water by a hybrid process, combining adsorption and Donnan dialysis. Integrated biological and physicochemical treatment for nitrate and fluoride removal was investigated by Mekonen et.al. (14). Fluoride distribution in electrocoagulation defluoridation process was investigated to explore the mechanism involved in fluoride removal process (15). The kinetics was developed empirically in the removal process of fluoride, using monopolar electrode connection (5, 16). Electro-coagulation is a simple and efficient method for removing the flocculating agent generated by electro-oxidation of a sacrificial anode, which is generally made of iron or aluminum. In this process, the treatment is performed without adding any chemical coagulant or flocculants; thus, it reduces the amount of sludge which must be disposed (17). On the other hand, electrocoagulation is based on in situ formation of the coagulant as the sacrificial anode corrodes due to an applied current, while simultaneous evolution of hydrogen at the cathode allows for pollutant removal by flotation. This technique combines three main interdependent processes, operating synergistically to remove the pollutants: electrochemistry, coagulation, and hydrodynamics. Assessment of the chemical reactions of electrocoagulation process shows that the main reactions occurring at the electrodes (aluminum and iron electrodes) are:


In addition, Al3+ and OH- ions generated at the electrode surfaces react in water to form aluminum hydroxide:

Similar chemical reactions occurring in the electrocoagulation process happen for iron electrodes:



The aluminum and iron hydroxide flocs normally act as adsorbents and/or traps for metal ions. Therefore, they eliminate metal ions from the solution (17).
2. Objectives
The main purpose of this research was to investigate the electrocoagulation process efficiency for fluoride removal from aqueous environments with iron and aluminum electrodes and determine the effects of voltage, distances between the electrodes, number of electrodes, electrode material, initial pH, initial concentration of fluoride, and reaction time on the removal efficiency. Finally, the electrical energy consumption for electrocoagulation process using aluminum and iron electrodes was calculated.
3. Materials and Methods
An experimental study in laboratory scale was carried out and a glass tank, 0.15 × 0.15 × 0.15 m, with effective volume of 3.375 m3 was used to conduct the experiments. Aluminum and iron electrodes with a total effective area of 120 cm2 were used. Thickness of aluminum and iron plates was 2 mm and the inter-electrode distance was maintained at 2 cm. The electrodes were connected to a direct-current (DC) power supply (PS-305 D, 0-5 A, 0-30 V). The batch electrocoagulation cell with bipolar electrode connection is shown in Figure 1 A. Magnetic stirring at 100 rpm maintained a homogeneous solution in the batch reactor. The temperature of each system was maintained at 25 ± 1°C. Samples were extracted from the Izeh drinking water supply, a city in Khoozestan province, Iran. Characteristics of Izeh water supply are shown in Table 1. Fluoride solutions were prepared synthetically by dissolving proper amounts of NaF in water. The fluoride concentration in each reaction was raised to 5 mg/L. Three different initial fluoride concentrations (1, 3, 5 mg/L) were used to test the influence of the initial fluoride concentration. Three voltages (5, 10, 20 V) were used to examine the effect of applied voltage. To achieve the desired pH, sulfuric acid or NaOH (0.1 N) were used. The pH values were measured using pH meter. Experiments were carried out in the influent pH values of 4, 7.5, 10, and with AL-AL and Fe-Fe electrodes in various contact times (10, 20, 30, 40 minutes). The treated fluoride samples were filtered before any analysis. The residual fluoride concentrations were analyzed using a spectrophotometer (DR/2000, Hach, Germany) at 580 nm according to the standard methods (18). Finally, the energy consumption of electrocoagulation process per cubic meter of treated water, during 40 minutes of detention in various situations was calculated.
Characteristics of Izeh Water Supply
Concentration | |
---|---|
Total hardness, mg/L CaCO3 | 250 |
Alkalinity, mg/L CaCO3 | 105 |
Electrical conductivity, μs/cm | 450 |
Fluoride, mg/L | 0.46 |
pH | 7.5 |
4. Results
4.1. Effect of Applied Voltage
Current density is the most important parameter for controlling the reaction rate in most electrocoagulation processes, because it determines the coagulant dosage rate, bubble production rate, and size and growth rate of the floc, which can influence the treatment efficiency of electrocoagulation (17, 19, 20). The effect of current density or applied voltage on fluoride removal was investigated. Figure 1 reveals the variation of fluoride concentration during electrocoagulation by aluminum and iron electrodes. As expected, for a given time, the removal efficiency increased with increase in the current density. In electrocoagulation with four iron electrodes with 20 mm distances and initial pH of 7.5, maximum fluoride removal was 29.6%. The residual fluoride concentration in the effluent at the end of the reaction time (40 minutes) did not reach to < 1.5 mg/L with iron electrodes. As seen in Figure 1 B, the highest electrical potential (20 V) produced the quickest treatment with 97.86% of the reductions occurring after 10 minutes and the lowest fluoride removal efficiency occurred in the lowest electrical potential (5 V). This is ascribed to the fact that at the higher voltage the amount of aluminum oxidized increases, resulting in a greater amount of precipitate for removal of pollutants. In addition, the bubbles densities increase and their sizes decrease with increase of the current density, resulting in a greater upward flux, faster removal of pollutants, and sludge flotation (17, 21). These findings were in line with the result of the following studies: Malakootian's study in 2009 on hardness removal by electrocoagulation (21); Bazrafshan et al. study in 2007 on the capability of electrocoagulation method with aluminum and iron electrodes in fluoride removal (17); Ghosh et al. study in 2008 on fluoride treatment with electrocoagulation using monopolar and bipolar electrode connections (6). As the current density decreased, the time to achieve similar efficiencies increased and the results of this research confirmed that the treatment efficiency was mainly affected by charge loading (Q = It), as reported by Bazrafshan et al. (17). It was also confirmed by Ghernaout's study in 2008 on Escherichia coli removal from the surface water by electrocoagulation (22). Hence, based on the results of the present study and previous studies, electrocoagulation can act as a pH moderator (17, 23).
The Effect of Applied Voltage on Fluoride Residue
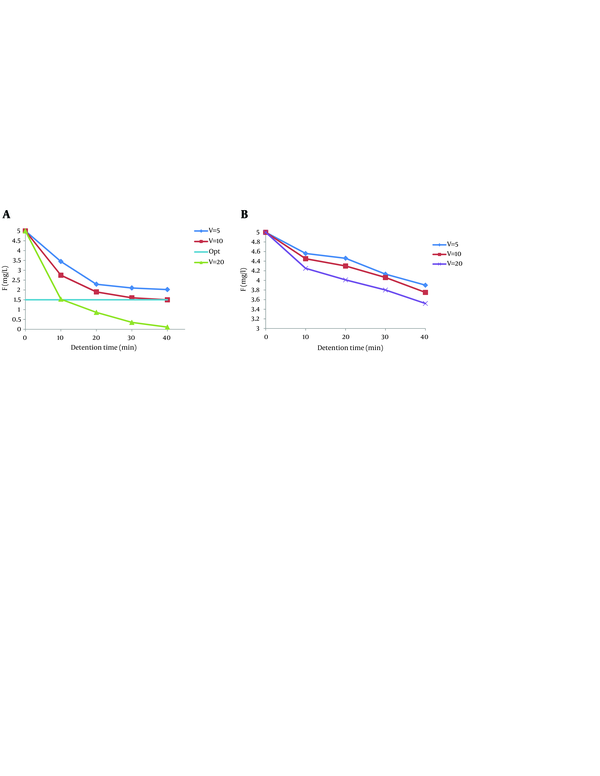
4.2. Effect of Distance Between the Electrodes
A set of experiments was performed with different electrode distances to determine the fluoride removal efficiency during electrocoagulation. The results obtained at 20, 30, and 40 mm electrode distances showed that the maximum fluoride removal was achieved at the minimum distance between the electrodes for iron and aluminum electrodes. As seen in Figure 2, the fluoride removal efficiency decreased with increase of the distance between the electrodes; so, the maximum fluoride removal was achieved at 20 mm distance between the electrodes in 40 minutes detention for both aluminum and iron electrodes. It was also confirmed by Escobar's study in 2006 on copper, lead, and cadmium removal from natural water sources by electrocoagulation as well as the results of Ghosh's study in 2008 on treatment of fluoride-containing drinking water by electrocoagulation, in which the optimum inter-electrode distance was 5 mm (6, 24).
The Effect of Distance Between the Electrodes on Fluoride Residue
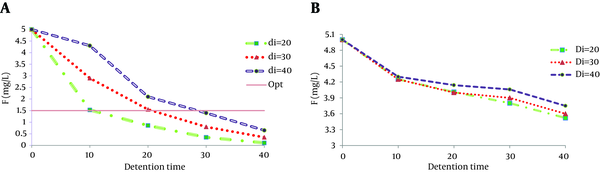
4.3. Effect of Number of Electrodes
The number of electrodes affecting the fluoride removal by electrocoagulation in equal conditions in two, four and six plates is shown in Figure 3. As the figure shows, up to 99% of fluoride removal was achieved with six aluminum electrodes and 36% of the removal was obtained with six iron electrodes. With increase in number of electrodes the electricity consumption increases, leading to increase of floc production and consequently removal efficiency enhancement (25).
The Effect of Number of Electrodes on Fluoride Residue
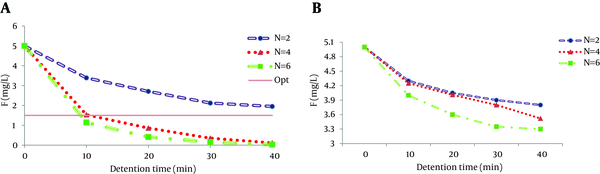
4.4. Effect of Initial pH
Initial pH has a considerable effect on the efficiency of electrocoagulation. pH of the medium can change during the process, depending on the electrode material and initial pH (17). In this study, pH values varied (4, 7.5, 10) to investigate its influence on fluoride removal. Removal efficiencies of fluoride with varying initial pH values using iron and aluminum electrodes are presented in Figure 4. As observed in the figure, the maximum fluoride removal for the aluminum electrode was achieved in pH = 4 and for the iron electrode in pH = 10. These findings were in line with the results of Bayramoglu et al. analyzing benzoquinone solution treatment by electrocoagulation (26) as well as the results of Malakootian et al. study in 2009 on hardness removal by iron electrodes via electrocoagulation (21).
The Effect of Initial pH on Fluoride Residue
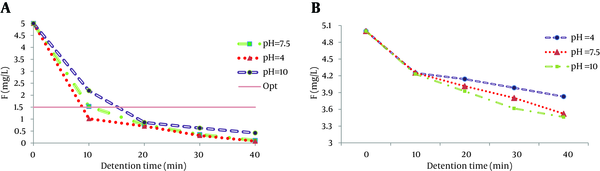
4.5. Effect of Contact Time
The effect of residence time on the defluoridation process in electrocoagulation was also investigated. Figure 5 shows the effect of detention in electrocoagulation at the initial pH and voltage, when the initial fluoride concentration was 5 mg/L. Theoretically, based on the Faraday’s law, current and duration of electrolysis should affect the quantity of aluminum and iron electrodes. As seen in Figure 5, up to 69% of the initial fluoride concentration decreased within 10 minutes of processing and the residual fluoride concentration at the end of the reaction time (40 minutes) reached to 1 mg/L for the aluminum electrodes. However, as shown in Figure 5, the residual concentration of fluoride with iron electrodes did not reach to < 1 mg/L, so it cannot be safely discharged to the environment. For both aluminum and iron electrodes, the maximum removal occurred in the first 10 minutes. Emamjomeh investigated fluoride removal by a continuous flow electrocoagulation reactor with aluminum electrodes and reported that maximum fluoride removal occurred in the initial fluoride concentration of 5 mg/L by 200 mL/min flow rate and current density of 12.5 A/m2 in 40 minutes of residence (20). The effect of reaction time for finding the optimum fluoride concentration in the effluent was investigated. The results showed that the reaction time increased when the current density decreased. With increase of the distances between the electrodes the reaction time increased, as in 30 mm interior electrode distance the reaction time for achieving the optimum fluoride removal was 20 minutes and it increased up to 30 minutes when the distance between the electrodes increased to 40 mm.
Effect of Contact Time on Fluoride Residue
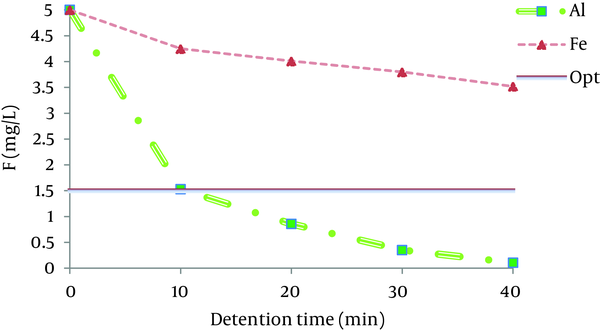
The effect of number of electrodes on the reaction time showed that with increase of the number of electrodes, the detention time decreased. The initial concentration of fluoride as well as the initial pH had direct effects on the reaction time, as with increase of the fluoride concentration and pH, the detention time increased. The effect of electrodes materials on the residence time revealed that the optimum fluoride concentration for a similar electrolysis condition was obtained in 10 minutes for the aluminum electrode and in 40 minutes for the iron electrode.
4.6. Effect of Initial Concentration of Fluoride Ion
Preliminary laboratory testing of electrolysis cell involved determining the effect of different initial concentrations of fluoride on efficiency of fluoride removal. From Figure 6 A, it appears that for various concentrations of fluoride, the residual fluoride concentration increased with increase of the fluoride concentration from 1 mg/L to 5 mg/L. Figure 6 B shows that higher concentrations of fluoride led to lower fluoride removal efficiencies of electrocoagulation with iron electrodes. As shown, the maximum fluoride removal was achieved in 1 mg/L, which was up to 43% and then decreased to 30% with increase of the fluoride concentration to 5 mg/L. Vasudevan et al. study in 2009 on an Mg-Al-Zn alloy as an anode for removal of fluoride through electrocoagulation reveal fluoride removal efficiency reduction from 96% to 20% with increase of fluoride concentration from 5 mg/L to 50 mg/L (27).
The Effect of Initial Concentration of Fluoride Ion on Fluoride Residue
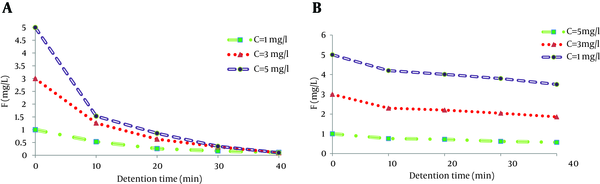
4.7. Effect of Electrode Material
As shown in Figure 5, aluminum electrodes are more effective for fluoride ion removal than iron electrodes. This is in complete agreement with the results obtained previously by Essadki et al. in 2009 (28) as well as Bazrafshan et al. results on fluoride removal (17).
4.8. Energy Consumption
The relationship between the electrical energy consumption and fluoride concentration are presented in Table 2 for iron and aluminum electrodes. In any electrical process, there are incurred charges due to electrical energy demand, which affect the operating costs. For an Electrocoagulation (EC) process, the operating costs include materials, mainly electrodes, electrical energy costs, labor, maintenance, sludge dewatering and disposal, and fixed costs. In this investigation, energy consumption was taken into account as a major cost in calculation of the operating costs. As the table represents, the energy consumption for the aluminum electrodes was dramatically less than the iron electrodes. In equal conditions of initial fluoride concentration, number of electrodes and distances between them, voltage and detention time, the energy consumption for aluminum and iron electrodes was respectively 1435 kwh/kgF- and 5590 kwh/kgF-. In addition, the energy consumption increased with increase of voltage and decreased with increase of distance between the electrodes.
Electrical Energy Consumption During Electrocoagulation Using Aluminum and Iron Electrodes
Variation | Removal Efficiency | Energy Consumption | ||||
---|---|---|---|---|---|---|
Aluminum | Iron | Aluminum | Iron | |||
kWh/m3 | kWh/kgF- | kWh/m3 | kWh/kgF- | |||
Current density, V | ||||||
5 | 59.6 | 22 | 0.29 | 97 | 0.32 | 293 |
10 | 70 | 25 | 1.56 | 445 | 1.87 | 1493 |
20 | 97.86 | 29.58 | 7.02 | 1435 | 8.27 | 5590 |
Electrode distance, mm | ||||||
20 | 97.86 | 29.58 | 7.02 | 1435 | 8.27 | 5590 |
30 | 93 | 28 | 5.33 | 1147 | 4.8 | 3429 |
40 | 86.96 | 25 | 4 | 920 | 4 | 3200 |
Number of electrodes | ||||||
2 | 61.02 | 24 | 2.04 | 670 | 2.53 | 2111 |
4 | 97.86 | 29.58 | 7.02 | 1435 | 8.27 | 5590 |
6 | 99.35 | 34 | 11.29 | 2273 | 12.22 | 7190 |
pH | ||||||
4 | 98.44 | 23.44 | 7.02 | 1427 | 8.27 | 7054 |
7.5 | 97.86 | 29.58 | 7.02 | 1435 | 8.27 | 5590 |
10 | 91.56 | 30.77 | 7.02 | 1534 | 8.27 | 5373 |
Initial concentration, mg/L | ||||||
1 | 89 | 43.09 | 4.89 | 5493 | 7.69 | 17844 |
3 | 96.67 | 38 | 6 | 2069 | 8 | 7018 |
5 | 98 | 30 | 7.02 | 1435 | 8.27 | 5590 |
5. Discussion
The present research investigated the applicability of the electrocoagulation process using aluminum and iron electrodes for fluoride removal from aqueous solutions. The influence of various variables such as pH, reaction time, voltage, fluoride concentration, electrode material, and distances between the electrodes was investigated. The results showed that electrocoagulation using iron and aluminum electrodes could successfully remove fluoride from the aqueous solutions. The results obtained with synthetic solutions revealed that the increase of detention time, voltage and number of electrodes enhanced the treatment rate for both iron and aluminum electrodes. The contact time for achieving the optimum residual fluoride increased with increase of the distances between the electrodes and the initial fluoride concentration and decreased with voltage and number of electrodes. The fluoride removal efficiency according to the study results decreased with increase of the distances between the electrodes. The energy consumption with aluminum electrodes was less than iron electrodes; thus, electrocoagulation with aluminum electrodes can be more effective compared with iron electrodes for fluoride removal.
Acknowledgements
References
-
1.
Emamjomeh MM, Sivakumar M, Safari Varyani A. Analysis and the understanding of fluoride removal mechanisms by an electrocoagulation/flotation (ECF) process. Desalination. 2011;275(1):102-6.
-
2.
Nemerow NL, Agardy FJ, Sullivan P, Salvato JA. Environmental engineering, water, wastewater, soil and ground water treatment and remediation. 6th ed. John Wiley & Sons, Inc; 2009. p. 23-6.
-
3.
Zuo Q, Chen X, Li W, Chen G. Combined electrocoagulation and electroflotation for removal of fluoride from drinking water. J Hazard Mater. 2008;159(2-3):452-7. [PubMed ID: 18359559]. https://doi.org/10.1016/j.jhazmat.2008.02.039.
-
4.
Behbahani M, Moghaddam MR, Arami M. Techno-economical evaluation of fluoride removal by electrocoagulation process: Optimization through response surface methodology. Desalination. 2011;271(1):209-18. https://doi.org/10.1016/j.desal.2010.12.033.
-
5.
Hu CY, Lo SL, Kuan WH. Simulation the kinetics of fluoride removal by electrocoagulation (EC) process using aluminum electrodes. J Hazard Mater. 2007;145(1-2):180-5. [PubMed ID: 17161907]. https://doi.org/10.1016/j.jhazmat.2006.11.010.
-
6.
Ghosh D, Medhi CR, Purkait MK. Treatment of fluoride containing drinking water by electrocoagulation using monopolar and bipolar electrode connections. Chemosphere. 2008;73(9):1393-400. [PubMed ID: 18840387]. https://doi.org/10.1016/j.chemosphere.2008.08.041.
-
7.
Wu X, Zhang Y, Dou X, Yang M. Fluoride removal performance of a novel Fe-Al-Ce trimetal oxide adsorbent. Chemosphere. 2007;69(11):1758-64. [PubMed ID: 17624402]. https://doi.org/10.1016/j.chemosphere.2007.05.075.
-
8.
Gupta VK, Ali I, Saini VK. Defluoridation of wastewaters using waste carbon slurry. Water Res. 2007;41(15):3307-16. [PubMed ID: 17583767]. https://doi.org/10.1016/j.watres.2007.04.029.
-
9.
Amor Z, Bariou B, Mameri N, Taky M, Nicolas S, Elmidaoui A. Fluoride removal from brackish water by electrodialysis. Desalination. 2001;133(3):215-23. https://doi.org/10.1016/S0011-9164(01)00102-3.
-
10.
Hichour M, Persin F, Sandeaux J, Gavach C. Fluoride removal from waters by Donnan dialysis. Sep Purif Technol. 1999;18(1):1-11. https://doi.org/10.1016/S1383-5866(99)00042-8.
-
11.
Hu K, Dickson JM. Nanofiltration membrane performance on fluoride removal from water. J Membr Sci. 2006;279(1):529-38.
-
12.
Tor A. Removal of fluoride from water using anion-exchange membrane under Donnan dialysis condition. J Hazard Mater. 2007;141(3):814-8. [PubMed ID: 16949737]. https://doi.org/10.1016/j.jhazmat.2006.07.043.
-
13.
Garmes H, Persin F, Sandeaux J, Pourcelly G, Mountadar M. Defluoridation of groundwater by a hybrid process combining adsorption and Donnan dialysis. Desalination. 2002;145(1):287-91. https://doi.org/10.1016/S0011-9164(02)00424-1.
-
14.
Mekonen A, Kumar P, Kumar A. Integrated biological and physiochemical treatment process for nitrate and fluoride removal. Water Res. 2001;35(13):3127-36. [PubMed ID: 11487109].
-
15.
Zhu J, Zhao H, Ni J. Fluoride distribution in electrocoagulation defluoridation process. Sep Purif Technol. 2007;56(2):184-91. https://doi.org/10.1016/j.seppur.2007.01.030.
-
16.
Emamjomeh MM, Sivakumar M. An empirical model for defluoridation by batch monopolar electrocoagulation/flotation (ECF) process. J Hazard Mater. 2006;131(1-3):118-25. [PubMed ID: 16298054]. https://doi.org/10.1016/j.jhazmat.2005.09.030.
-
17.
Bazrafshan E, Ownagh KA, Mahvi AH. Application of electrocoagulation process using Iron and Aluminum electrodes for fluoride removal from aqueous environment. J Chem. 2012;9(4):2297-308. https://doi.org/10.1155/2012/876739.
-
18.
Standard methods for the examination of water and wastewate. 21th ed. Washington DC: American Water Works Association; 2005.
-
19.
Holt PK, Barton GW, Wark M, Mitchell CA. A quantitative comparison between chemical dosing and electrocoagulation. Colloids Surf A Physicochem Eng Asp. 2002;211(2):233-48. https://doi.org/10.1016/S0927-7757(02)00285-6.
-
20.
Emamjomeh MM, Sivakumar M. Fluoride removal by a continuous flow electrocoagulation reactor. J Environ Manage. 2009;90(2):1204-12. [PubMed ID: 18649987]. https://doi.org/10.1016/j.jenvman.2008.06.001.
-
21.
Malakootian M, Yousefi N. The efficiency of electrocoagulation process using aluminum electrodes in removal of hardness from water. J Environ Health Sci Eng. 2009;6(2):131-6.
-
22.
Ghernaout D, Badis A, Kellil A, Ghernaout B. Application of electrocoagulation in Escherichia coli culture and two surface waters. Desalination. 2008;219(1):118-25. https://doi.org/10.1016/j.desal.2007.05.010.
-
23.
Kim TH, Park C, Shin EB, Kim S. Decolorization of disperse and reactive dyes by continuous electrocoagulation process. Desalination. 2002;150(2):165-75. https://doi.org/10.1016/S0011-9164(02)00941-4.
-
24.
Escobar C, Soto-Salazar C, Toral MI. Optimization of the electrocoagulation process for the removal of copper, lead and cadmium in natural waters and simulated wastewater. J Environ Manage. 2006;81(4):384-91. [PubMed ID: 16616411]. https://doi.org/10.1016/j.jenvman.2005.11.012.
-
25.
Mahvi AH, Bazrafshan E, Mesdaghinia AR, Naseri S, Vaezi F. [Investigation the applicability of electrocoagulation process using aluminum electrodes in removing chromium from aqueous environments water and wastewater]. Water Wastewater J. 2007;62:28-34.
-
26.
Bayramoglu M, Kobya M, Eyvaz M, Senturk E. Technical and economic analysis of electrocoagulation for the treatment of poultry slaughterhouse wastewater. Sep Purif Technol. 2006;51(3):404-8.
-
27.
Vasudevan S, Lakshmi J, Sozhan G. Studies on a Mg‐Al‐Zn Alloy as an Anode for the Removal of Fluoride from Drinking Water in an Electrocoagulation Process. Clean Soil Air Water. 2009;37(4‐5):372-8. https://doi.org/10.1002/clen.200900031.
-
28.
Essadki AH, Gourich B, Vial C, Delmas H, Bennajah M. Defluoridation of drinking water by electrocoagulation/electroflotation in a stirred tank reactor with a comparative performance to an external-loop airlift reactor. J Hazard Mater. 2009;168(2-3):1325-33. [PubMed ID: 19375221]. https://doi.org/10.1016/j.jhazmat.2009.03.021.