Abstract
Background and Objectives:
Nitrogen is a potential pollutant of water resources. They penetrate in water resources through insufficiently treated wastewater that is rich in nitrogen. The present study aimed at evaluating the efficiency of an anoxic/aerobic cycling reactor (AACR) with continuous flow in removing nitrogen and organic matter from wastewater.Methods:
Experiments were performed using a reactor with continuous intermittent influent and effluent. In this reactor, 4 phases with aeration and mixing cycles were designed and efficiency of each phase in removing nitrogen compounds was evaluated at 600 mg/L chemical oxygen demand (COD) concentration, 40 mg/L ammonia concentration, and 18- to 30-hour hydraulic retention times (HRT).Results:
Results demonstrated that all cycles had great performance in removal of COD. Average COD removal efficiencies in phases 1, 2, 3, and 4 were 93%, 96.3%, 96%, and 94%, respectively. In phase 2, removal efficiency of 98.7% was obtained at hydraulic retention time of 24 hours, COD concentration of 600 mg/L, and ammonia concentration of 40 mg/L.Conclusions:
Phase 2 showed great efficiency in removal of nitrogen compounds. Combination of anoxic aeration stages and short cycles in anoxic/aerobic cycling reactor resulted in great performance of this reactor in removing nitrogen from wastewater. As a result, continuous influent and effluent flow, and not needing external carbon resulted in AACR good performance in removing nitrogen compounds and organic materials.Keywords
1. Background
Given the growing concerns about water quality in the world, approaches applied for wastewater treatment are playing key roles in environment protection. Regarding the increasing shortage of fresh water, false wastewater treatment could bring about many problems for the society. Also, releasing industrial and municipal wastewater is a serious environmental challenge for water resources (1-4).
Besides, nutrients are considered among the main factors of wastewater pollution. Indeed, nutrients’ penetration in final resources, such as lakes, can cause environmental problems, including algal bloom phenomenon, and lack of water quality for drinking, industrial, agricultural, and recreational usages (5, 6).
One important objective of wastewater treatment is removing nitrogen. The methods used for removing nitrogen compounds, include physical methods (reverse osmosis and air stripping), chemical methods (ion exchange and chlorination to the point of failure), and biological methods (nitrification-denitrification) (7, 8). Biological methods for wastewater treatment are one of the important priorities in the field of environmental engineering due to their compatibility with the environment, lower cost, lower sludge production, and higher flexibility compared to other methods (9, 10).
Among different biological processes, nitrification and denitrification processes are considered superior due to their economic advantages and efficient wastewater treatment (11, 12).
Nutrients biological removal processes include phordox (A/O), A2/O, 5-step Bardenpho process, Keep Town University (UCT) process, modified UCT, Virginia initiative plant (VIP), Phostrip processes, and sequencing batch reactor (SBR) process. The aerobic-anaerobic (A/O) process is designed for reducing phosphorus. The main features of this process are short hydraulic retention time (HRT), sludge production with good sedimentation, and good phosphorus removal; however, it is not able to remove nitrogen and phosphorus, simultaneously (13).
The SBR process in wastewater treatment has advantages of higher flexibility, less sludge, and low costs of operation; however, the flow is discontinuous in SBR and needs a more complex design. Additionally, other processes require a more complicated operation (14, 15).
Given these problems, as well as the necessity to remove nutrients from wastewater, it is essential to develop a method with a high quality outlet sewage, easy operation, low need for energy and land, and expandability in the future (16). What distinguishes this system from similar methods is the special design of this reactor, in which sedimentation is done and exploitation is facilitated through automatic return of sludge. The main idea for inventing this new reactor was developing a process, in which by integrating A/O and SBR methods, good features of both methods are enforced and their deficiencies are reduced.
Based on what was mentioned above, this study aimed at investigating the application of the invented aerobic anoxic cyclic reactor for removing nitrogen compounds and organic matter from wastewater and to assess the effects of different exploitation parameters on reactor’s performance.
2. Methods
2.1. Operational PHASES
According to Tables 1 and 4 different operational phases were used in AACR in order to determine the best conditions for removing nitrogen compounds. In these phases, efficiency of removing nitrogen compounds and organic matter was also investigated at different chemical oxygen demand (COD) concentrations of 400, 600, and 800 mg/L, HRT convert to 18, 24, and 30 hours, and internal sludge return percentage of 100%, 150%, and 200%.
Designed Phases for Operational Anoxic/Aerobic Cycling Reactor
Phase | Tank 1 | Tank 2 |
---|---|---|
1 | Aeration continues without mixing | Aeration 1 hour -mixing 1 hour |
2 | Aeration 1 hour -mixing 1 hour | Aeration 1 hour -mixing 1 hour |
3 | Aeration 2 hours -mixing 2 hours | Aeration 2 hours -mixing 2 hours |
4 | Aeration 1 hour -mixing 2 hours | Aeration 2 hours -mixing 1 hour |
2.2. Specifications of Wastewater and Chemical Materials
In this study, synthetic wastewater was used. The materials needed for producing synthetic sewage have been listed in Tables 2 and 3. In this wastewater, the carbon source, including glucose and sucrose, was used in order to maintain the rate of COD/N/P to 100/5/1 and NH4CL and KH2PO4, were used as a nitrogen source and phosphorus source, respectively. Furthermore, synthetic wastewater contained different combinations of trace elements, including 0.82 mg/L sodium molybdate (Na2MoO4), 0.44 mg/L zinc sulfate (ZnSO4), 0.22 mg/L iron chloride (FeCl3), 0.42 mg/L cobalt chloride (CoCl2), and 0.42 mg/L copper sulfate (CuSO4). In order to produce the synthetic sewage, municipal water of Shiraz was used. A 20-liter tank was used to reserve synthetic sewage. The synthetic sewage was produced daily in order to prevent the contamination, which may result from microorganisms’ growth in the tank and other contaminations (17).
Characteristics of the Influent to the Reactor (17)
Material | Concentration, g/L |
---|---|
Glucose | 0.3 - 0.6 |
Sucrose | 0.1 - 0.2 |
NH4Cl | 0.15 - 0.2 |
KH2PO4 | 0.011 - 0.013 |
The Amount and Type of Material Needed to Prepare the Stock Solution Trace Elements (17)
Trace Elements | Concentration, mg/L |
---|---|
Na2MoO4 | 0.82 |
ZnSO4 | 0.44 |
FeCl3 | 0.22 |
CoCl2 | 0.42 |
CuSO4 | 0.42 |
2.3. Anoxic/Aerobic Cycling Reactor
This study was carried out in a pilot scale. The study sample was the synthetic wastewater produced in the laboratory and the flow entered the reactor continuously. It should also be noted that AACR was made of plexiglas with total volume of 28 liters and useful volume of 18 liters. This reactor was 46 cm long and 21 cm wide and 21 cm height and it consisted of three horizontal parts separated from each other through a baffle in order to reach uniform flow. In the first and second part, aeration and mixing were set up decussately. In the third part, sedimentation unit was designed diagonally for automatic return of sludge. The incoming wastewater was supplied by a peristaltic pump from a 20-liter tank. The schematic image of the reactor and its parts has been depicted in Figure 1 and actual image of AACR showed in Figure 2.
Schematic presentation of a novel anoxic/aerobic cycling reactor. 1, feed tank; 2, peristaltic pump; 3, 4, 5, timer; 6, 8, aeration pump, 7, 9, mixing; 10, recycle sludge line; 11- tank 1, 12, tank 2; 13, recycle sludge pump, 14, settling; 15, effluent tank.
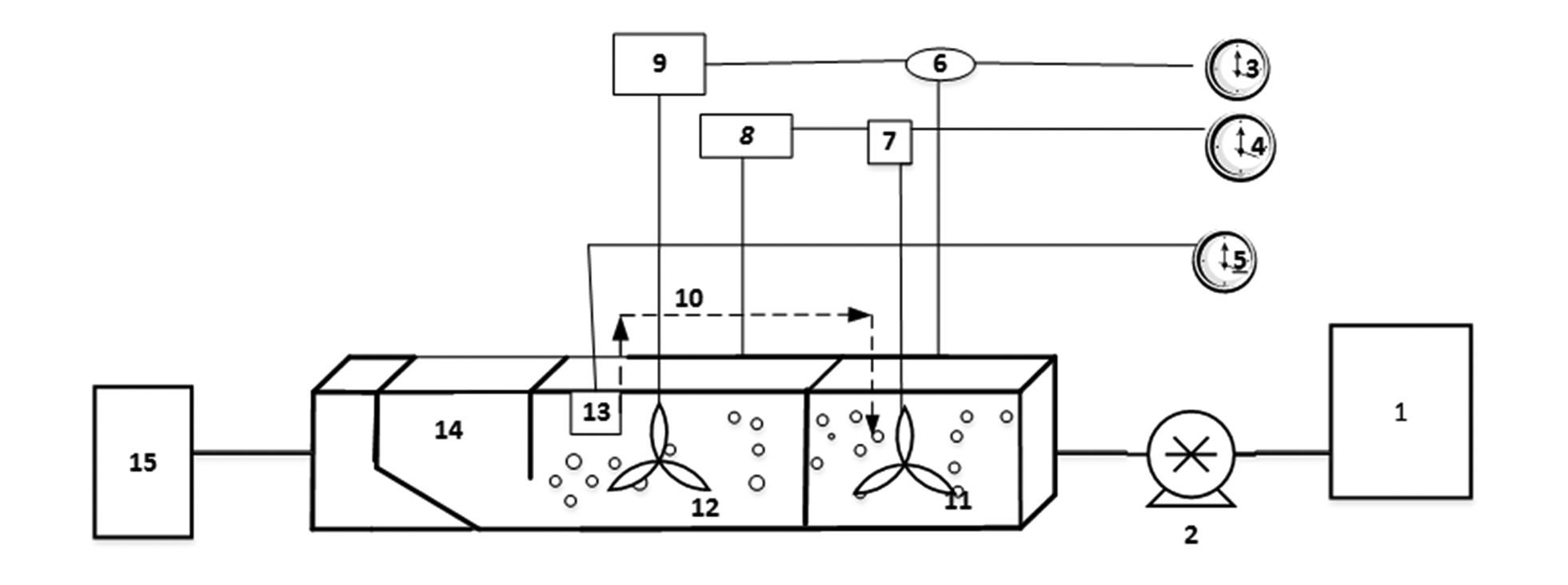
Image of Anoxic/Aerobic Cycling Reactor
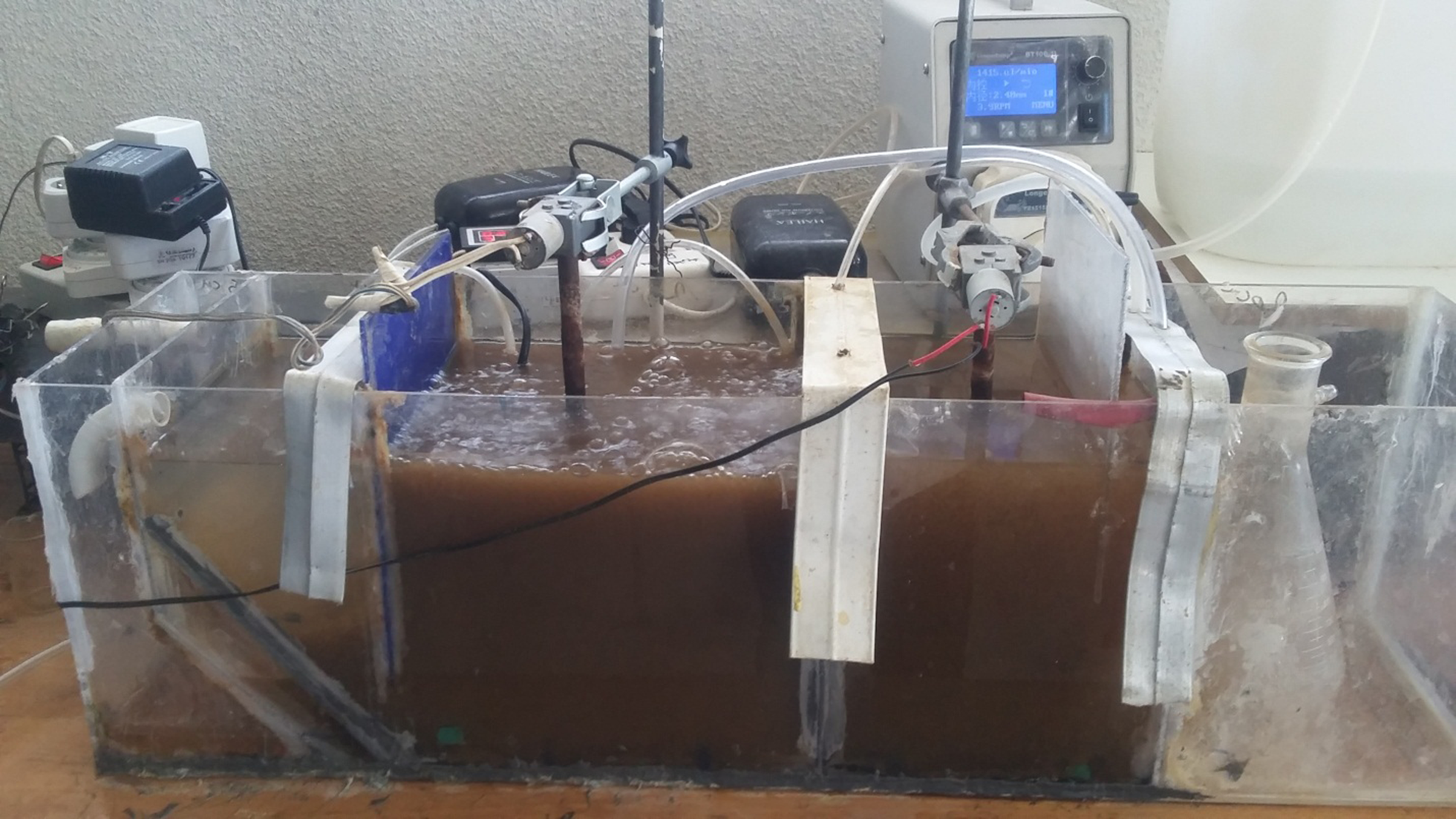
2.4. Reactor Operation
The aforementioned reactor was set up during 180 days. Initially, in order to operate the reactor, the returned sludge from the second sedimentation unit of Shiraz municipal wastewater treatment plant with an approximate volume of 2 liters in tanks 1 and 2 was used for microbial seeding of the system. Daily feeding with synthetic sewage was done through the peristaltic pump (L 100-1 longer pump BT). It should be mentioned that incoming and outgoing flows of wastewater were continuous.
The temperature of the reactor operation environment was set at 20°C. During the operation, a diffuser connected to the HAILIA air pump was used at the bottom of tank 1 and 2 for aeration. To ensure uniform air distribution, especially at lower rates of aeration, and to make anoxic conditions, a mixer with 4 blades and 50 rounds per minute, with 40 cm length, 20 cm of which was in the wastewater, was set up on top of the reactor. The amount of dissolved oxygen (DO) was 2 to 3 mg/L in the aerobic phase and less than 0.2 mg/L in the anoxic phase. This was measured through a Do-meter of HACH company and was monitored continuously and daily. Additionally, the pH of the sample was kept between 7 and 7.5, and it was measured using the Mertrohm pH-meter. Moreover, internal return of sludge was carried out through RS ELECTIAL aquarium pump from tank 2 to tank 1, which was connected to a digital timer. In order to evaluate the performance of AACR reactor and to decrease the operation costs 100%, 150%, and 200% internal sludge return was used. Moreover, mixed liquor suspended solid (MLSS) of the reactor was kept in the range of 1500 to 2500 mg/L. It should be noted that 2 analog timers were used for controlling the time in different phases. One timer was connected to the aeration of tank 1 and mixing of tank 2 while the other timer was connected to the aeration of tank 2 and mixing of tank 1, and hydraulic retention time was monitored as well.
2.5. Experiment Method
In order to measure COD in 620 nm wavelength and MLSS, standard methods were used (18). In addition, spectrophotometer-based methods (DR5000HACH-model) were applied to measure the concentration of ammonia (NH3) at 425 nm wavelength, nitrate (NO3) at 500 nm wave lenght, and nitrite (NO2) at 507 nm wave length in incoming wastewater and outgoing sewage from the reactor. The Nessler method (No.8038) was used for measuring ammonia, cadmium resuscitation method (No. 8039) for measuring nitrate, and deazotizon method (No.8507) for measuring nitrite.
2.6. Statistical Method
In order to compare the mean concentration of parameters, analysis of variance (ANOVA) was used and to compare the mean concentrations between distribution systems the SPSS software was used.
3. Results
3.1. Chemical Oxygen Demand Removal
The AACR performance in removing COD with incoming COD concentration of 600 mg/L and internal return of 100% in performed phases is shown in Figure 3. The efficiency of COD removal at 400 to 800 mg/LCOD concentration was also investigated in phase 2. According to Figure 3, at 600 mg/L incoming COD concentration the efficiency of COD removal in phases 1, 2, 3, and 4 was 93%, 96.3%, 96%, 94%, respectively. Additionally, at 400 mg/L and 800 mg/L COD concentration in phase 2, the efficiency of COD removal was 93% and 96.3%, respectively. The results indicated that the average concentration of outgoing COD was < 50 mg/L for all phases. As the output standard for municipal wastewater treatment in Iran is 100 mg/L for discharge into surface waters and 200 mg/L for agricultural and irrigation usages, the results of the present study correspond with the above mentioned standards.
Evaluation Influent COD Removal of AACR at Influent COD Concenteration 600 mg/L and Internal Recycle 100% in Four Phases
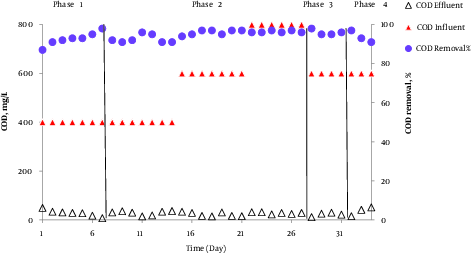
3.2. Removing Nitrogen Compounds
In this study, ammonia, nitrate, and nitrite compounds were measured to examine the performance of AACR in removing nitrogen compounds. According to Figure 4, phase1 and 2 had the best performance in removing ammonia and nitrification and more than 97% of ammonia was removed in these phases. Additionally, according to Figure 5, the highest concentration of effluent nitrate was observed in phase 1. The average concentration of effluent nitrate and nitrite in phase 2 was 12 mg/L NO3-N and 0.14 mg/L NO2-N, respectively, which indicates appropriate denitrification. Moreover, the average concentration of effluent nitrate and nitrite was respectively15.52 mg/L NO3-N, and 0.15 mg/L NO2 in phase3 and 14.5 mg/L NO3-N, and 0.3 mg/L NO2-N in phase 4. In phase 2, with 150% internal return, the amount of incoming ammonia increased from 40 mg/L to 60 mg/L and COD concentration increased to 800 mg/L. In addition, the efficiency of ammonia removal increased to 96% and the average concentration of nitrate and nitrite reached 10.2 mg/L and 0.15 mg/L, respectively. The results indicated that at 600 mg/L COD concentration and 150% internal return, the efficiency of ammonia removal was 97% and the average concentration of nitrate and nitrite was 8.4 mg/L and 0.13 mg/L, respectively. However, Table 4 compares effluent mean concentration of nitrogen compounds in 4 phases different by AACR.
Comparison of Effluent Mean Concentration of Nitrogen Compounds in 4 Phases Different by AACR
Phases | NH3 Effluent, mg/L | NO2 Effluent, mg/L | NO3 Effluent, mg/L |
---|---|---|---|
1 | 0.66 | 0.47 | 28.12 |
2 | 1.05 | 0.14 | 12.1 |
3 | 2.84 | 0.15 | 15.68 |
4 | 6.6 | 0.29 | 14.4 |
Removal of Influent Ammonia in Influent COD Concentration 600 mg/L and Influent Ammonia 40 mg/L by Internal Recycle Sludge 100% at Different Phases
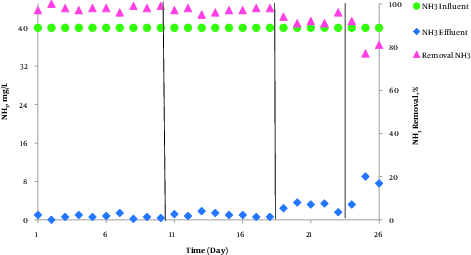
Removal of Ammonia and Producion of Nitrate and Nitrite in Influent COD Concentration 600 mg/L and Influent Ammonia 40 mg/L by Internal Return 100% in Different Phases. Error bars represent the standard deviation.
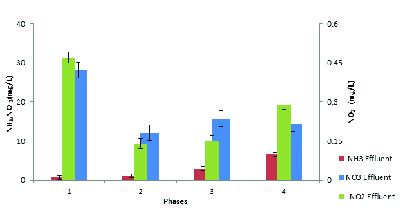
4. Discussion
In all phases, COD removal was implemented very well. The results demonstrated that by increasing the incoming COD concentration to 800 mg/L in phase 2, the efficiency of COD removal increased the concentration of 600 mg/L and then, it became stable. In this phase, the reactor was able to tolerate COD loading shocks and wastewater with severe contamination. According to Figure 3, despite different applied conditions in phases 1 to 4, the efficiency of COD removal remained high and AACR showed good performance in removing COD. By increasing ammonia concentration to 60 mg/L in phase 2, the efficency of ammonia removal reached higher than 90%. Simoultanious removal of nitrogen compounds and organic matter often has good results (17).
In previous studies, Jiang et al. tried to remove nutrients and carbon, simultaneously, and by using a loop airlift reactor under balking limited conditions. The results demonstrated that the reactors efficiency was 91%, 92%, 86%, and 94% for COD, NH4, TN, and TP, respectively (19). According to Jiang et al., simultaneous removal of nitrogen compounds and organic matter was done very well, as well and this was due to urgent need of denitrifier microorganisms to organic matter for removing nitrogen compounds during the denitrification process.
In another study, Yang evaluated a consecutive discontinuous reactor with a moveable bed and with a membrane module for simultaneous removal of nitrogen, phosphorus, and COD. The results showed that in anaerobic phase, remaining nitrate, and nitrite were removed by using COD as a substrate and it had a good performance in removing the organic matter (20).
Similarly, the results of the present study indicated that AACR had good efficiency in removing COD. However, operations in AACR, showed 96% efficiency in COD removal without using any module membranes or any complexities in operation and design. Generally, the most common wastewater treatment systems have a good performance in removing COD from wastewater. However, their efficiency in removing nutrients, such as nitrogen compounds and phosphorus, was very low and about 30%. According to Liu and Hu’s studies, specific environmental conditions have been considered for successful implementation of nitrification and denitrification. For example, the amount of dissolved oxygen has been reported to be 2 to 5 mg/L in aerobic nitrification and less than 0.3 mg/L in anoxic denitrification (21, 22). In the current study, phase 2 with 1-hour aerobic anoxic cycle showed better performance in removing nutrients. Increase in nutrients removal efficiency in phase 2 could be attributed to the shortness of the aerobic anoxic cycle. Long aerobic cycle that was set up in phase 3 and 4, in spite of increasing nitrification and nitrate production, could reduce the organic matter required for heterotrophic denitrifier microorganisms. Therefore, the concentration of outgoing nitrate increased in these phases.
In the research of Freitas and Cassidy, increase in anaerobic/aerobic sequences and decrease in cycles duration improved the efficiency of the SBR in removing compounds. Hence, short aerobic anoxic phases could improve the outgoing sewage for biological nitrogen removal (BNR) and at the same time reduced the amount of carbon required for denitrification in order to decrease the operation costs (23, 24). In Ding’s study, carried out in a vertical membrane bioreactor, the researchers found the best nitrogen removal efficiency in internal return of 400% compared to 100% and 200% internal return. In contrast to Ding’s study, in which higher internal return percentage improved the denitrification process, in the present study, AACR showed the best efficiency for nitrogen removal in 150% compared to 100% and 200% internal return. Therefore, the rate of internal return was directly related to energy consumption and operation costs (25).
4.1. Conclusion
In the present study, AACR showed good efficiency in removing nitrogen compounds and organic matter and was able to remove COD and nitrogen compounds, simultaneously. In addition, the best removal efficiency was observed in COD concentration of 600 mg/L, 24-hour HRT, and 150% internal return. In this study, 2 aerobic anoxic processes were placed in the reactor. Furthermore, AACR with cycling aerobic anoxic stages showed good efficiency in removing nitrogen compounds and organic matter in phase 2. In other words, the key advantage of this new reactor, includes lower space requirements through the use of compressed tanks, simple design and exploitation, lower sludge production, and automatic sludge return, and the efficient simultaneous removal of nitrogen compounds and organic matter.
Acknowledgements
References
-
1.
Aslan S, Kapdan IK. Batch kinetics of nitrogen and phosphorus removal from synthetic wastewater by algae. Ecol Eng. 2006;28(1):64-70. https://doi.org/10.1016/j.ecoleng.2006.04.003.
-
2.
Rawat I, Ranjith Kumar R, Mutanda T, Bux F. Dual role of microalgae: Phycoremediation of domestic wastewater and biomass production for sustainable biofuels production. Appl Energy. 2011;88(10):3411-24. https://doi.org/10.1016/j.apenergy.2010.11.025.
-
3.
Malla FA, Khan SA, Rashmi, Sharma GK, Gupta N, Abraham G. Phycoremediation potential of Chlorella minutissima on primary and tertiary treated wastewater for nutrient removal and biodiesel production. Ecol Eng. 2015;75:343-9. https://doi.org/10.1016/j.ecoleng.2014.11.038.
-
4.
Wang S, Peng Y. Natural zeolites as effective adsorbents in water and wastewater treatment. Chem Eng J. 2010;156(1):11-24. https://doi.org/10.1016/j.cej.2009.10.029.
-
5.
Chowdhury N, Nakhla G, Zhu J. Load maximization of a liquid-solid circulating fluidized bed bioreactor for nitrogen removal from synthetic municipal wastewater. Chemosphere. 2008;71(5):807-15. [PubMed ID: 18262217]. https://doi.org/10.1016/j.chemosphere.2007.11.070.
-
6.
Abdel daiem MM, Rivera-Utrilla J, Ocampo-Perez R, Mendez-Diaz JD, Sanchez-Polo M. Environmental impact of phthalic acid esters and their removal from water and sediments by different technologies--a review. J Environ Manage. 2012;109:164-78. [PubMed ID: 22796723]. https://doi.org/10.1016/j.jenvman.2012.05.014.
-
7.
Renman A, Hylander LD, Renman G. Transformation and removal of nitrogen in reactive bed filter materials designed for on-site wastewater treatment. Ecol Eng. 2008;34(3):207-14. https://doi.org/10.1016/j.ecoleng.2008.08.006.
-
8.
Zhang Y, Angelidaki I. A new method for in situ nitrate removal from groundwater using submerged microbial desalination-denitrification cell (SMDDC). Water Res. 2013;47(5):1827-36. [PubMed ID: 23375601]. https://doi.org/10.1016/j.watres.2013.01.005.
-
9.
Kim YM, Park D, Lee DS, Park JM. Inhibitory effects of toxic compounds on nitrification process for cokes wastewater treatment. J Hazard Mater. 2008;152(3):915-21. [PubMed ID: 17804160]. https://doi.org/10.1016/j.jhazmat.2007.07.065.
-
10.
Sibag M, Kim HS. Nitrification denitrification enhanced biological phosphorous removal (NDEBPR) occurs in a lab-scale alternating hypoxic/oxic membrane bioreactor. Bioresour Technol. 2012;104:173-80. [PubMed ID: 22130083]. https://doi.org/10.1016/j.biortech.2011.11.001.
-
11.
Ruiz G, Jeison D, Chamy R. Nitrification with high nitrite accumulation for the treatment of wastewater with high ammonia concentration. Water Res. 2003;37(6):1371-7. [PubMed ID: 12598199]. https://doi.org/10.1016/S0043-1354(02)00475-X.
-
12.
Hua G, Salo MW, Schmit CG, Hay CH. Nitrate and phosphate removal from agricultural subsurface drainage using laboratory woodchip bioreactors and recycled steel byproduct filters. Water Res. 2016;102:180-9. [PubMed ID: 27344249]. https://doi.org/10.1016/j.watres.2016.06.022.
-
13.
Metcalf E, Eddy M. Wastewater engineering treatment and reuse. McgrawHill; 2003.
-
14.
Monclus H, Puig S, Coma M, Bosch A, Balaguer MD, Colprim J. Nitrogen removal from landfill leachate using the SBR technology. Environ Technol. 2009;30(3):283-90. [PubMed ID: 19438061]. https://doi.org/10.1080/09593330802622105.
-
15.
Dubber D, Gray NF. The effect of anoxia and anaerobia on ciliate community in biological nutrient removal systems using laboratory-scale sequencing batch reactors (SBRs). Water Res. 2011;45(6):2213-26. [PubMed ID: 21329959]. https://doi.org/10.1016/j.watres.2011.01.015.
-
16.
Park W, Nam YK, Lee MJ, Kim TH. Simultaneous nitrification and denitrification in a CEM (cation exchange membrane)-bounded two chamber system. Water Res. 2009;43(15):3820-6. [PubMed ID: 19564033]. https://doi.org/10.1016/j.watres.2009.05.039.
-
17.
Azhdarpoor A, Mohammadi P, Dehghani M. Simultaneous removal of nutrients in a novel anaerobic–anoxic/aerobic sequencing reactor: removal of nutrients in a novel reactor. Int J Environ Sci Technol. 2015;13(2):543-50. https://doi.org/10.1007/s13762-015-0871-5.
-
18.
American Public Health Association, American Water Works Association, Water Environmental Federation. Standard methods for the examination of water and wastewater. 21st ed. Washington DC: American Public Health Association; 2005.
-
19.
Jiang M, Zhang Y, Zhou X, Su Y, Zhang M, Zhang K. Simultaneous carbon and nutrient removal in an airlift loop reactor under a limited filamentous bulking state. Bioresour Technol. 2013;130:406-11. [PubMed ID: 23313686]. https://doi.org/10.1016/j.biortech.2012.11.129.
-
20.
Yang S, Yang F, Fu Z, Wang T, Lei R. Simultaneous nitrogen and phosphorus removal by a novel sequencing batch moving bed membrane bioreactor for wastewater treatment. J Hazard Mater. 2010;175(1-3):551-7. [PubMed ID: 19896271]. https://doi.org/10.1016/j.jhazmat.2009.10.040.
-
21.
Hu Z, Zhang J, Li S, Xie H, Wang J, Zhang T, et al. Effect of aeration rate on the emission of N2O in anoxic-aerobic sequencing batch reactors (A/O SBRs). J Biosci Bioeng. 2010;109(5):487-91. [PubMed ID: 20347772]. https://doi.org/10.1016/j.jbiosc.2009.11.001.
-
22.
Liu Y, Shi H, Xia L, Shi H, Shen T, Wang Z, et al. Study of operational conditions of simultaneous nitrification and denitrification in a Carrousel oxidation ditch for domestic wastewater treatment. Bioresour Technol. 2010;101(3):901-6. [PubMed ID: 19818603]. https://doi.org/10.1016/j.biortech.2009.09.015.
-
23.
Freitas F, Temudo MF, Carvalho G, Oehmen A, Reis MA. Robustness of sludge enriched with short SBR cycles for biological nutrient removal. Bioresour Technol. 2009;100(6):1969-76. [PubMed ID: 19056261]. https://doi.org/10.1016/j.biortech.2008.10.031.
-
24.
Cassidy DP, Belia E. Nitrogen and phosphorus removal from an abattoir wastewater in a SBR with aerobic granular sludge. Water Res. 2005;39(19):4817-23. [PubMed ID: 16278003]. https://doi.org/10.1016/j.watres.2005.09.025.
-
25.
Ding A, Qu F, Liang H, Ma J, Han Z, Yu H, et al. A novel integrated vertical membrane bioreactor (IVMBR) for removal of nitrogen from synthetic wastewater/domestic sewage. Chem Eng J. 2013;223:908-14. https://doi.org/10.1016/j.cej.2013.01.096.