Abstract
Background:
Fusarium sp. and Rhizoctonia sp. fungi have been always threats to short-term crops. In Vietnam, corn and soybean suffer serious losses annually. Therefore, it is necessary to utilize an environmentally friendly antifungal compound that is highly effective against phytopathogenic fungi. Pseudomonas sp. is a popular soil bacterial strain and well known for its high antifungal activity.Objectives:
This study was carried out to evaluate and assess the antifungal activity of a local bacterial strain namely DA3.1 that was later identified as Pseudomonas aeruginosa. This would be strong scientific evidence to develop an environmentally friendly biocide from a local microorganism strain for commercial use.Methods:
The antifungal compound was purified from ethyl acetate extraction of deproteinized cell culture broth by a silica gel column (CH2Cl2/MeOH (0% - 10% MeOH)). The purity of the isolated compound was determined by HPLC, and its molecular structure was elucidated using spectroscopic experiments including one-dimensional (1D) (1H NMR, 13C NMR, DEPT) and two-dimensional (2D) (HMBC and HSQC) spectra. The activity of the purified compound against Fusarium sp. and Rhizoctonia sp. fungi was measured using the PDA-disk diffusion method, and its growth-promoting ability was evaluated using the seed germination test of corn and soybean.Results:
The results showed that the antifungal compound produced by Pseudomonas aeruginosa DA3.1 had a retention factor (Rf) of 0.86 on thin layer chromatography (TLC). Based on the evidence of spectral data including proton nuclear magnetic resonance (1H NMR), carbon nuclear magnetic resonance (13C NMR), distortionless enhancement by polarization transfer (DEPT), heteronuclear multiple bond correlation (HMBC), and heteronuclear single quantum coherence (HSQC), the chemical structure was elucidated as phenazine-1-carboxylic. The purified compound showed inhibitory activity against F. oxysporum and R. solani and exhibited the ability of the germination of corn and soybean seeds. The results revealed the benefit of native P. aeruginosa DA3.1 and phenazine-1-carboxylic acid for use as a biocontrol agent, as well as a plant growth promoter.Conclusions:
The antifungal compound isolated from local Pseudomonas DA3.1 was identified as phenazine-1-carboxylic acid that posed high antifungal activity and was a plant germination booster.Keywords
Antifungal Pseudomonas aeruginosa Identification Spectrum Analysis
1. Background
The past decades have witnessed a huge effort of mankind shifting from traditional agriculture to a sustainable model. The dramatic movement aims to protect the environment, expand natural resource bases, and maintain and improve soil fertility. Biological control is considered the most important method for sustainable cultivation by utilizing the advantages of biocides. It cuts down the dependence on synthetic pesticides, lessens the adverse environmental impacts, and enhances farmers’ safety, along with preserving the economic viability of crop production (1, 2). In the latter half of the seventies, Pseudomonas spp. were recognized as biocontrol and plant growth promoters (3, 4). Documents have emphasized several antibiotics developed by the strains of Pseudomonas such as phenazine (5, 6), 2,4-diacetylphloroglucinol (7), pyrrolnitrin (8), pyoluteorin (9), and siderophore (10) and their main functions are to control a variety of plant infection-causing fungi (11).
2. Objectives
In this study, we investigated a newly isolated microorganism, DA3.1, from Vietnam soil. The secondary metabolites were purified, identified, and evaluated for growth promotion and antifungal activity against R. solani and F. oxysporum through the ability of the germination of corn and soybean seeds.
3. Methods
3.1. Microorganisms
R. solani and F. oxysporum strains were provided by the Plant Diseases Division of the Institute of Plant Protection, Tu Liem, Hanoi, Vietnam. Besides, DA3.1 was isolated from a soil sample in Vietnam. The isolation method was adopted from a previous study (12). Accordingly, a mycelial plug aged four days of F. oxysporum and R. solani was put in the center of a PDA plate. At the same time, the microorganisms were streaked in one line separately at different corners of the plate and incubated for five days. The strain had antifungal activity and showed no growth of fungal mycelia near the microorganism colonies. In the next step, the microorganism was identified as Pseudomonas aeruginosa by the partial sequencing of 16S rRNA (GenBank code no.: JN592444.1).
3.2. Culture Conditions
Pseudomonas aeruginosa DA3.1 was grown in a 1000 mL flask with 250 ml BDT medium at pH = 7.5 containing 10 g soybean extract, 10 g glucose, 0.5 g MgSO4, 0.5 g KH2PO4, and 5 g NaCl. The inoculated flasks were incubated on a rotary shaker at 150 rpm for five days at 30°C.
3.3. Determination of Antifungal Activity of Extracellular Extracts
Various ratios of cultured broth were applied to the PDA plates at 55°C, mixed well, and poured into Petri dishes (90 mm × 15 mm). The crude supernatants’ final concentrations were 5, 10, 20, and 50%. Finally, a mycelial plug (0.5 cm diameter) aged four days of R. solani or F. oxysporum was placed in the center of each PDA plate and incubated for five days at 30°C. The PDA plates without extracellular extracts served as negative controls. The growth of R. solani and F. oxysporum was determined by measuring the mycelium diameter. The inhibitory activity was calculated using the following formula: I(%) = [(Scontrol - Ssamples)/Scontrol] × 100, where S was the area occupied by the mycelium on PDA plates with and without supplementing crude extract of samples and control, respectively.
3.4. Purification of the Antifungal Compound
For this purpose, 100 mL of cell culture broth was centrifuged at 12,000 rpm for 15 min. The supernatant was examined by HPLC and acidified with phosphoric acid adjusting to pH = 2.5. Next, the precipitated protein was removed by centrifugation at 12,500 rpm for After the sample was dried in vacuo by vaporation. The residue was dissolved in absolute methanol in a ratio of 1:10 (w/vl). 4 ml of this sample was loaded to a silica gel column for purification. Next, 20 fractions of 1 mL each were collected at 20 min intervals. The antifungal compounds were detected by TLC. To this end, 10 µL of samples were chromatographed on TLC plates (Merck, Germany) with a solvent (chloroform:methanol; 7:3 (vl/vl)). Then, they were sprayed with iodine. After purification, the fractions were mixed and evaporated at 40°C in vacuo and crystallized.
3.5. Purity Determination of the Isolated Compound
For this purpose, HPLC was performed with an Agilent Technologies 1200 series system with DAD-G1315D, Zorbax extend C18 column (5 μm, 4.6 mm × 250 mm), linear-gradient [2% - 98% (v/v)] of methanol in water, a sample size of 5 µL, column temperature of 40°C, a flow rate of 0.5 mL/min, maximum UV absorption at 254 nm in methanol, and running time of 80 min.
3.6. Identification of the Purified Compound
Spectroscopic experiments were carried out to elucidate the structure of the putative compound. The NMR spectra were recorded by a Bruker Advance 500 MHz instrument (Bruker, Germany) using TMS as the internal standard. The one-dimensional (1D) (1H NMR, 13C NMR, DEPT) and two-dimensional (2D) (HMBC and HSQC) spectra were used for the completed assignment.
3.7. Determination of Antifungal Activity of the Purified Compound
The antifungal activity of the purified compound was measured using the agar-disk diffusion method. First, a mycelial plug from aged days of R. solani or F. oxysporum was placed in the center of each PDA plate embedded with 0.1% ampicillin. Next, 15 µL of the purified compound was dropped on a sterilized filter paper with 0.5 cm diameter (Whatman paper no.: 3) and put onto the plate surface. Negative control disks were soaked in absolute methanol. These agar plates were then incubated for 3 - 5 days at 30°C (Incubator, Sanyo, Japan), and then, the diameters of inhibition zones around the disk thrice were measured.
3.8. Evaluation of the Effects of the Purified Compound on Germination of Corn and Soybean Seeds
Good-quality seeds were sterilized with 5% NaOCl for four minutes and washed with distilled water four times. The effects of the purified compound on the germination of corn and soybean seeds were evaluated at varying concentrations (5, 10, 15, 20, and 25 µg/mL) and different treatment times (15, 30, 45, 60, and 75 min). Seeds were transferred to a moisture paper (Whatman paper no.: 2) at 25°C after they had been soaked with the purified compound and positive control-PCA (Fluka) solutions. The germination rate, amount of roots, and length of sprouts were determined. Controls were normal-dry seeds without PCA treatment.
3.9. Statistical Analysis
All measurements were carried out in triplicate and analyzed by IRRISTAT 4.0 software. The value that we presented in our figure is the mean of 3 replicated experiments with standard deviation calculated by IRRISTAT 4.0 software.
4. Results
4.1. Antifungal Activity of the Extracellular Extracts of Pseudomonas aeruginosa DA3.1
Four different concentrations of P. aeruginosa DA3.1 extracellular extracts (5%, 10%, 20%, and 50%) were examined for their antifungal activities against R. solani and F. oxysporum. The results (Figure 1) showed that the extracellular extracts of P. aeruginosa DA3.1 suppressed both fungal pathogens. At the culture broth concentration of 50%, the growth inhibition reached 51.2% and 94.1% for F. oxysporum and R. solani, respectively. The crude supernatant exhibited antifungal activity against the growth of phytopathogenic fungi, which was the evidence of putative phenazine compounds. This antifungal activity of the extracellular extracts of P. aeruginosa DA3.1 was confirmed by monitoring the germination and growth of R. solani sclerotia. When R. solani sclerotia were grown on the PDA medium containing 5% or 10% crude supernatant, it was still able to induce germination. However, the growth of sclerotia was inhibited by more than 30% by 10% crude supernatant and over 97% by a concentration of 20%. It was almost completely inhibited by a concentration of 50% (Figure 1).
Inhibitory activity of extracellular extracts from Pseudomonas aeruginosa DA3.1 on the growth of Fusarium oxysporum (A) and Rhizoctonia solani (B)
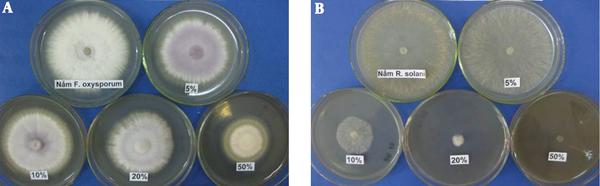
4.2. Purification and Identification of the Chemical Structure of the Antifungal Compound
The antifungal compound was purified from extracellular extracts of P. aeruginosa DA3.1 using the silica gel column as described above. The collected fractions confined a single band on TLC, suggesting the uniformity of the putative compounds with an Rf of about 0.86 (Figure 2). The purified fractions were collected and elucidated to determine the chemical formula. The putative compound was obtained in the form of yellow crystals with a melting point of 237°C - 239°C. The chemical structure of this compound was further elucidated by 1H NMR and 13C NMR spectroscopy, DEPT experiments, and various two-dimensional NMR spectral studies, including HMBC and HSQC. In the 1H NMR spectrum, seven peaks in the region of 7.93 - 8.95 ppm were shown, indicating the presence of seven aromatic protons. The chemical shift of the carboxyl group appeared at δ 15.41 ppm. There were 13 signals in the 13C NMR in the DEPT spectrum, including 12 peaks between 124.92 and 144.02 ppm, indicating the presence of 12 aromatic carbons. Among them, four carbon signals at δ 139.8, 140.1, 143.4, and 144.1 ppm suggested that they were attached to nitrogen atoms. The carboxylic acid carbon was observed at δ 165.80 ppm.
TLC analysis of the extracellular extract and purified fraction of Pseudomonas aeruginosa DA3.1: Lane 1, purified fraction; lane 2, extracellular extract
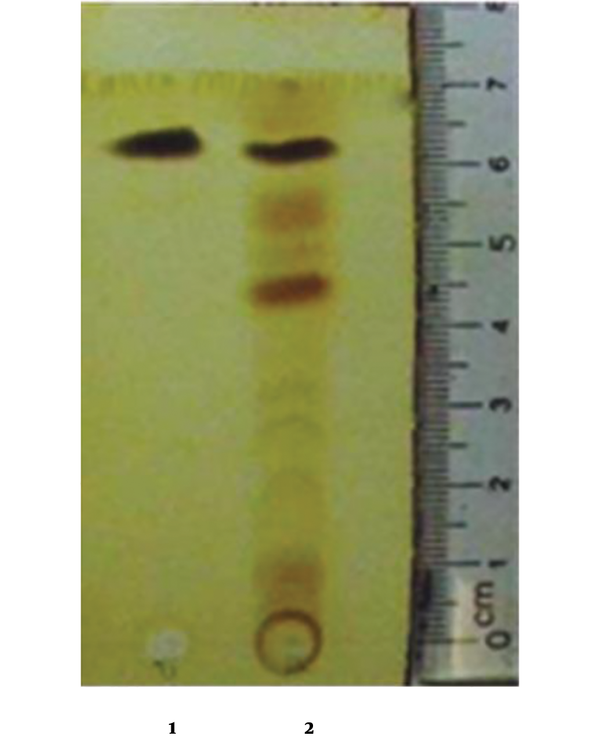
The HSQC spectrum gave a correlation between the carbons and their attached protons: H(2)/C(2), H(3)/C(3), H(4)/C(4), H(6)/C(6), H(7)/C(7), H(8)/C(8), and H(9)/C(9). The HMBC correlations are illustrated in Figure 3: from H-2 [δH 8.50 (dd, 7.0 Hz, 1.5 Hz)] to C-11 [δC 165.9]/ C-3 (δC 137.4]/C-4 [δC 135.1]; from H-3 [δH (8.22 - 8.49, m)] to C-2 [δC 130.1]/ C-4 [δC 135.1]/ C-4a [δC 139.1]; from H-4 [δH 8.95 (dd, 8.7 Hz, 1.5 Hz)] to C-4a (δC 139.8)/ C-2 [δC 130.1]; from H-9 [δH (7.93 - 8.02, m)] to C-9a [δC 143.4]/ C-8 [δC 130.2]/ C-7 [δC 128.0]; and from H-6 [δH (7.93 - 8.02, m)] to C-5a [δC 140.1]/ C-8 [δC 130.2]. From the evidence mentioned above, the antifungal compound was identified as phenazine-1-carboxylic acid (C13H8N2O2). The signals of the putative antifungal compound were matched with P. aeruginosa GC-B26 strain isolated in Korea (Table 1) (13). All of the spectra had similar values to those of PCA. The next experiments were carried out to assess the antifungal activity of the antifungal compound extracted from P. aeruginosa DA3.1.
Structure of the antifungal compound (A) PCA and (B) HMBC correlations
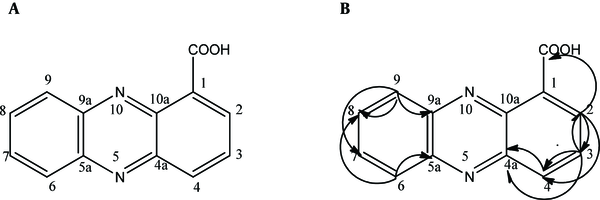
1H NMR and 13C NMR Data of the Purified Compounda
Atom Position | δC | δ*C | δH | δ*H |
---|---|---|---|---|
1 | 124.9 | 125.2 | - | - |
2 | 130.1 | 130.5 | 8.50 (dd, 7.0, 1.5) | 8.57 (dd, 7.0, 1.5) |
3 | 137.4 | 137.6 | 8.22 - 8.49 (m) | 8.31 - 8.39 (m) |
4 | 135.1 | 135.3 | 8.95 (dd, 8.7, 1.5) | 9.01 (dd, 8.7, 1.5) |
4a | 139.8 | 140.1 | - | - |
5a | 140.1 | 140.3 | - | - |
6 | 131.7 | 131.9 | 7.93 - 8.02 (m) | 7.99 - 8.08 (m) |
7 | 128.0 | 128.2 | 8.22 - 8.49 (m) | 8.31 - 8.39 (m) |
8 | 130.2 | 130.3 | 8.22 - 8.49 (m) | 8.31 - 8.39 (m) |
9 | 133.2 | 133.4 | 7.93 - 8.02 (m) | 7.99 - 8.08 (m) |
9a | 143.4 | 143.6 | - | - |
10a | 144.1 | 144.3 | - | - |
11 (-COOH) | 165.9 | 166.1 | 15.41 | 15.61 (m) |
The concentration of putative PCA in the supernatant and the purity of isolated PCA were determined by the HPLC method. Accordingly, a standard calibration was established using different concentrations of PCA (Fluka): y = 90926.58349x + 30.65723 (Figure 4A), in which, y is the area under peak, and x is the concentration of putative phenazine. The PCA concentration was calculated as 31 µg/µL (Figure 4B), and the purity of PCA was verified as 93.8% (Figure 4C). From 100 mL of the initial supernatant, the total PCA amount was calculated as 3.1 mg, followed by purified PCA reaching 0.72 mg after flowing through the chromatography column, making the purification efficacy of PCA about 23.2%. The active antifungal component was examined using the agar disk diffusion method. The results revealed that purified PCA suppressed the proliferation of both fungi. However, the inhibitory activity was slightly higher against R. solani than against F. oxysporum (Figure 5).
Determination of PCA concentration from extracellular extracts by HPLC method: A, Standard calibration of PCA (Fluka); B, sample from extracellular extracts; C, the purity of purified PCA.
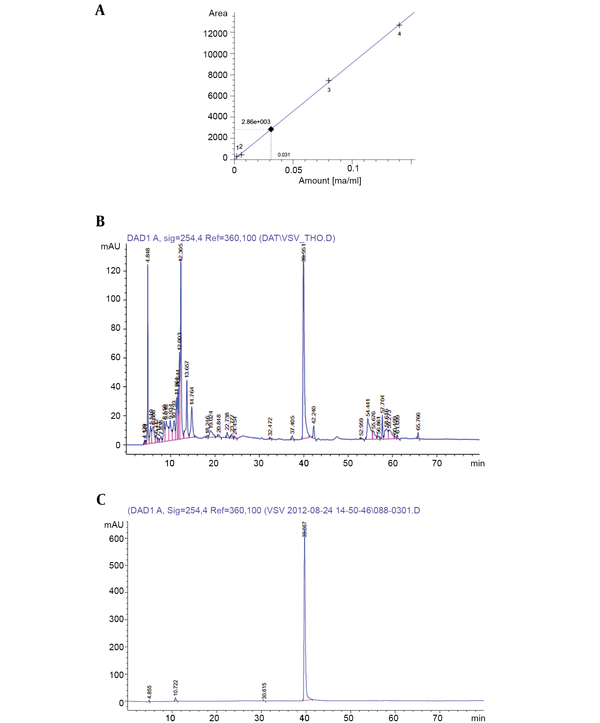
Antifungal activity of the purified PCA against F. oxysporum (A) and R. solani (B) D/C-: Negative control (15 µL of absolute methanol); D/C +: Positive control (15 µL of standard PCA (Fluka) at a concentration of 5 µg); 1 - 4: 15 µL of purified PCA at concentrations of 2, 5, 10, and 12 µg, respectively.
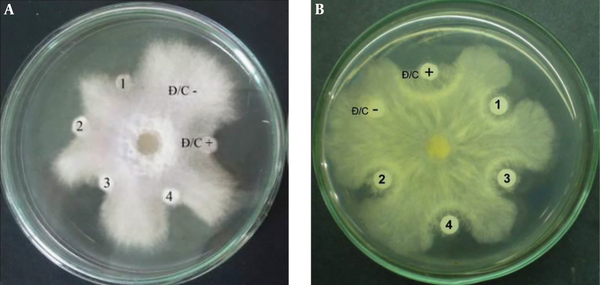
4.3. Effects of PCA on the Germination of Corn and Soybean Seeds
To evaluate the effects of purified PCA on the germination of soybean and corn, the experiments were carried out by soaking seeds in a solution of PCA at different concentrations for 30 min, as described earlier. Next, seeds were placed on a moisture paper at room temperature and evaluated for the germination rate, length of sprouts, and extent of roots. The results showed that the germination capacity of corn seeds in all PCA-treated groups was higher than that of the control group. The germination rates were from 91.4% to 97.5% in the purified PCA treatment group, while that of the control group was 88.9%.
The extent of roots and the length of sprouts were also significantly higher in the treatment group than in the control group (Table 2, Figure 6). Similarly, the germination capacity of soybean seeds after treatment with purified PCA and PCA (Fluka) was significantly different from that of controls. The germination rate of the control group reached 53.1%, and the average length of sprouts was 0.3 cm, while in the PCA-treated groups, the germination rate reached 66.7% to 85.2%, and the length of sprouts was 0.4 to 1.2 cm (Table 2). To investigate the effect of treatment time on the germination rate, seeds were soaked in a solution of 20 µg/mL PCA for 15, 30, 45, 60, and 75 min. The results showed that the highest response was observed in the 30 min treatment groups of soybean and corn seeds by 68.1 and 96.4%, respectively, while that was the lowest in the 75 min treatment groups (Table 3). This evidence suggested that the treatment of seeds with PCA promoted the germination of soybean and corn seeds.
Effects of PCA on the Germination of Corn and Soybean Seedsa
Treatment Dose, µg/mL | Corn Seeds (After Six Days) | Soybean Seeds (After Six Days) | ||||||
---|---|---|---|---|---|---|---|---|
Germination Rate, % | Duration of germination, d | Amount of Roots | Length of Sprouts, cm | Germination Rate, % | Duration of germination, d | Amount of Roots | Length of Sprouts, cm | |
PCA 5 µg | 92.6AB | 3 | 1.1C | 1.4B | 66.7D | 3 | 0.4C | 0.4C |
PCA 10 µg | 91.4AB | 3 | 1.7B | 1.6B | 69.1C | 3 | 0.6B | 0.6B |
PCA 15 µg | 92.3AB | 3 | 2.7A | 1.6B | 70.7C | 3 | 0.7B | 0.8B |
PCA 20 µg | 93.8AB | 3 | 2.9A | 1.7A | 73.2B | 3 | 0.9A | 1.1A |
PCA 25 µg | 94.6AB | 3 | 3.1A | 1.8A | 74.4B | 3 | 1.0A | 1.2A |
PCA(Fluka) 10 µg | 97.5A | 3 | 3.2A | 1.9A | 85.2A | 3 | 1.0A | 1.4A |
Control | 88.9B | 3 | 1.0C | 1.3C | 53.1E | 3 | 0.4C | 0.3C |
CVb, % | 3.7 | 5.1 | 7.2 | 4.0 | 6.4 | 8.5 |
Effects of PCA Treatment Time on the Germination of Corn and Soybean Seedsa
Treatment time | Corn Seeds (After Six Days) | Soybean Seeds (After Six Days) | ||||
---|---|---|---|---|---|---|
Germination Rate, % | Amount of Roots, Roots | Length of Sprouts, cm | Germination Rate, % | Amount of Roots, Roots | Length of Sprouts, cm | |
15 min | 92.1A | 2.2B | 1.4B | 61.1AB | 1.1B | 0.9B |
30 min | 96.4A | 3.1A | 1.7A | 68.1A | 1.4A | 1.2A |
45 min | 95.7A | 2.9A | 1.8A | 64.7A | 1.5A | 1.3A |
60 min | 91.4A | 1.1C | 0.6D | 53.6B | 0.7C | 0.6C |
75 min | 85.4B | 0.5D | 0.3E | 51.3B | 0.3D | 0.4D |
Control | 89.7AB | 1.0C | 1.1C | 58.4AB | 0.9BC | 0.7C |
CVb, % | 9.7 | 6.8 | 5.7 | 9.3 | 7.4 | 6.3 |
Effects of PCA (Fluka) and different concentrations of purified PCA on the germination of corn seeds
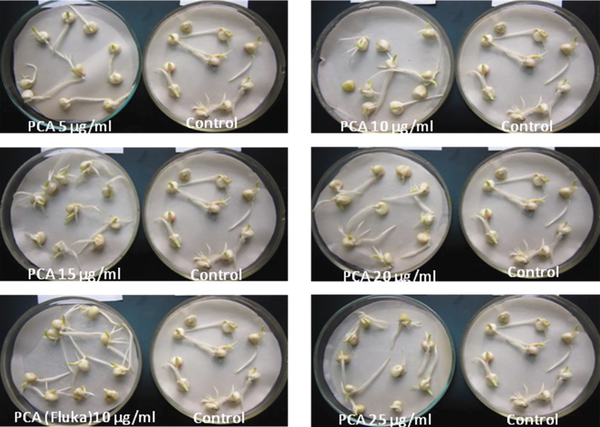
5. Discussion
R. solani and F. oxysporum are two widespread pathogenic fungi that cause several plant diseases. They both live in soil and are transmitted to many plants, including leguminous, solanaceous, cucurbits, and cotton plants (14, 15). To find out which biocontrol agent is efficient and economic, the antifungal activity of P. aeruginosa DA3.1 (JN592444.1) was examined and elucidated. The purified band was constrained on the TLC layer at an Rf of 0.86. This suggested further proof of the diversity of antifungal compounds produced by different Pseudomonas sp. strains. Putative antifungal compounds from P. fluorescens strains were observed on TLC at Rf of 0.22, 0.35, 0.42, and 0.51. The antifungal component with Rf of 0.35 completely inhibited the mycelial growth of all fungal pathogens at 0.5%, while another antifungal compound at Rf of 0.22 showed a 71% - 89% inhibition (16). In another report, P. fluorescens strains produced various types of antibiotics including diacetylphloroglucinol, phenazine, and pyoluteorin. The bands were confined at TLC at the Rf values of 0.88, 0.57, and 0.05, respectively (17).
Other research revealed that the most commonly identified derivatives produced by Pseudomonas sp. are pyocyanin (18), phenazine-1-carboxylic acid (19), phenazine-1-carboxamide (20), and several hydroxy phenazines with broad-spectrum antibiotic activity (21). Among them, PCA was reported to possess strong antifungal activity (22), especially from P. fluorescens (5) and P. aureofaciens (23). Besides, another report suggested that PCA seems to be a potent agent in ecological fitness (24). Corn and soy are the most economically important crops damaged by the above-mentioned fungal diseases. Seed-borne pathogenic fungi can obviate germination, deteriorate seedlings, and hinder plant growth by damaging the roots and vascular system.
Consequently, the disease impedes the transport of water and nutrients (25, 26). In addition, seed-borne pathogenic fungi can cause losses of mass and grade of common crops worldwide, including Alternaria alternata (Fr.) Keissler, Aspergillus niger Van Tiegh, F. moniliforme Sheldon, Fusarium sp., Penicillium sp., and Ustilago zeae Unger (27). Therefore, seed treatments have been shown to prevent the epidemics of plant diseases caused by seed-borne fungal pathogens. In addition, seed treatments can be used in reducing the quantities of pesticides required for managing diseases, and eliminate the need for foliar application (28). In this study, we also witnessed an alternative benefit of PCA in promoting the growth of corn seeds, followed by the increase in the number and length of roots. This trait is not only possessed in P. aeruginosa but also in relative species of Pseudomonas spp. (29).
5.1. Conclusions
In conclusion, we successfully purified and identified the chemical structure of an antifungal compound from native P. aeruginosa DA3.1 and evaluated its effects on the germination of corn and soybean seeds. The significant inhibitory activity of P. aeruginosa DA3.1 against R. solani and F. oxysporum and the positive effects of its metabolite constitute, PCA, on the germination of corn and soybean seed suggest its potential utility as an alternative to chemical pesticides for preventing fungal plant diseases.
Acknowledgements
References
-
1.
Forgia D, Verheggen F. Biological alternatives to pesticides to control wireworms (Coleoptera: Elateridae). Agri Gene. 2019;11(100080). https://doi.org/10.1016/j.aggene.2018.100080.
-
2.
Tracy EF. The promise of biological control for sustainable agriculture: A stakeholder-based analysis. J Sci Policy Governance. 2015;5(1).
-
3.
Adhikary A, Kumar R, Pandir R, Bhardwaj P, Wusirika R, Kumar S. Pseudomonas citronellolis; a multi-metal resistant and potential plant growth promoter against arsenic (V) stress in chickpea. Plant Physiol Biochem. 2019;142:179-92. [PubMed ID: 31299600]. https://doi.org/10.1016/j.plaphy.2019.07.006.
-
4.
Kakembo D, Lee YH. Analysis of traits for biocontrol performance of Pseudomonas parafulva JBCS1880 against bacterial pustule in soybean plants. Biol Control. 2019;134:72-81. https://doi.org/10.1016/j.biocontrol.2019.04.006.
-
5.
Gorantla JN, Kumar SN, Nisha GV, Sumandu AS, Dileep C, Sudaresan A, et al. Purification and characterization of antifungal phenazines from a fluorescent Pseudomonas strain FPO4 against medically important fungi. J Mycol Med. 2014;24(3):185-92. [PubMed ID: 24746721]. https://doi.org/10.1016/j.mycmed.2014.02.003.
-
6.
Veselova M, Klein S, Bass IA, Lipasova VA, Metlitskaia AZ, Ovadis MI, et al. [Quorum sensing systems of regulation, synthesis of phenazine antibiotics, and antifungal (corrected) activity in rhizospheric bacterium Pseudomonas chlororaphis 449]. Genetika. 2008;44(12):1617-26. Russian. [PubMed ID: 19178080].
-
7.
Patel JK, Archana G. Engineered production of 2,4-diacetylphloroglucinol in the diazotrophic endophytic bacterium Pseudomonas sp. WS5 and its beneficial effect in multiple plant-pathogen systems. Appl Soil Ecol. 2018;124:34-44. https://doi.org/10.1016/j.apsoil.2017.10.008.
-
8.
Huang R, Feng Z, Chi X, Sun X, Lu Y, Zhang B, et al. Pyrrolnitrin is more essential than phenazines for Pseudomonas chlororaphis G05 in its suppression of Fusarium graminearum. Microbiol Res. 2018;215:55-64. [PubMed ID: 30172309]. https://doi.org/10.1016/j.micres.2018.06.008.
-
9.
Wang QL, Zhang XH, Fan LY, Zhang W, Xu YQ, Hu HB, et al. Quantitative analysis of pyoluteorin in anti-fungal fermentation liquor of Pseudomonas species by capillary zone electrophoresis with UV-vis detector. J Chromatogr B Analyt Technol Biomed Life Sci. 2005;826(1-2):252-6. [PubMed ID: 16140044]. https://doi.org/10.1016/j.jchromb.2005.07.047.
-
10.
Dowling DN, O'Gara F. Metabolites of Pseudomonas involved in the biocontrol of plant disease. Trends Biotechno. 1994;12(4):133-41. https://doi.org/10.1016/0167-7799(94)90091-4.
-
11.
Haas D, Defago G. Biological control of soil-borne pathogens by fluorescent pseudomonads. Nat Rev Microbiol. 2005;3(4):307-19. [PubMed ID: 15759041]. https://doi.org/10.1038/nrmicro1129.
-
12.
Weller DM. Biological control of soilborne plant pathogens in the rhizosphere with bacteria. Annu Rev Phytopathol. 1988;26(1):379-407. https://doi.org/10.1146/annurev.py.26.090188.002115.
-
13.
Lee JY, Moon SS, Hwang BK. Isolation and in vitro and in vivo activity against Phytophthora capsici and Colletotrichum orbiculare of phenazine-1-carboxylic acid from Pseudomonas aeruginosa strain GC-B26. Pest Manag Sci. 2003;59(8):872-82. [PubMed ID: 12916768]. https://doi.org/10.1002/ps.688.
-
14.
Thaines Bodah E. Root rot diseases in plants: A review of common causal agents and management strategies. Agricult Res Technol. 2017;5(3). https://doi.org/10.19080/artoaj.2017.05.555661.
-
15.
Koyyappurath S, Conejero G, Dijoux JB, Lapeyre-Montes F, Jade K, Chiroleu F, et al. Differential responses of vanilla accessions to root rot and colonization by Fusarium oxysporum f. sp. radicis-vanillae. Front Plant Sci. 2015;6:1125. [PubMed ID: 26734032]. [PubMed Central ID: PMC4683197]. https://doi.org/10.3389/fpls.2015.01125.
-
16.
Reddy KRN, Choudary KA, Reddy MS. Antifungal metabolites of Pseudomonas fluorescens isolated from rhizosphere of rice crop. J Mycol Pl Pathol. 2007;37(2):1-5.
-
17.
Prabhukarthikeyan SR, Raguchander T. Antifungal metabolites of Pseudomonas fluorescens against Pythium aphanidermatum. J Pure Appl Microbiol. 2016;10(1):579-84.
-
18.
Abdul-Hussein ZR, Atia SS. Antimicrobial effect of pyocyanin extracted from Pseudomonas aeruginosa. Eur J Exp Biol. 2016;6(3):1-4.
-
19.
Jain R, Pandey A. A phenazine-1-carboxylic acid producing polyextremophilic Pseudomonas chlororaphis (MCC2693) strain, isolated from mountain ecosystem, possesses biocontrol and plant growth promotion abilities. Microbiol Res. 2016;190:63-71. [PubMed ID: 27394000]. https://doi.org/10.1016/j.micres.2016.04.017.
-
20.
Peng H, Zhang P, Bilal M, Wang W, Hu H, Zhang X. Enhanced biosynthesis of phenazine-1-carboxamide by engineered Pseudomonas chlororaphis HT66. Microb Cell Fact. 2018;17(1):117. [PubMed ID: 30045743]. [PubMed Central ID: PMC6060551]. https://doi.org/10.1186/s12934-018-0962-3.
-
21.
Pierson L3, Pierson EA. Metabolism and function of phenazines in bacteria: impacts on the behavior of bacteria in the environment and biotechnological processes. Appl Microbiol Biotechnol. 2010;86(6):1659-70. [PubMed ID: 20352425]. [PubMed Central ID: PMC2858273]. https://doi.org/10.1007/s00253-010-2509-3.
-
22.
Morohoshi T, Wang WZ, Suto T, Saito Y, Ito S, Someya N, et al. Phenazine antibiotic production and antifungal activity are regulated by multiple quorum-sensing systems in Pseudomonas chlororaphis subsp. aurantiaca StFRB508. J Biosci Bioeng. 2013;116(5):580-4. [PubMed ID: 23727350]. https://doi.org/10.1016/j.jbiosc.2013.04.022.
-
23.
Raio A, Reveglia P, Puopolo G, Cimmino A, Danti R, Evidente A. Involvement of phenazine-1-carboxylic acid in the interaction between Pseudomonas chlororaphis subsp. aureofaciens strain M71 and Seiridium cardinale in vivo. Microbiol Res. 2017;199:49-56. [PubMed ID: 28454709]. https://doi.org/10.1016/j.micres.2017.03.003.
-
24.
Mazzola M, Cook RJ, Thomashow LS, Weller DM, Pierson L3. Contribution of phenazine antibiotic biosynthesis to the ecological competence of fluorescent pseudomonads in soil habitats. Appl Environ Microbiol. 1992;58(8):2616-24. [PubMed ID: 1514808]. [PubMed Central ID: PMC195829]. https://doi.org/10.1128/AEM.58.8.2616-2624.1992.
-
25.
Mathur SB, Haware MP, Hampton RO. Identification, significance and transmission of seed borne pathogens. Part of the current plant science and biotechnology in agriculture. World crops: Cool season food legumes. Springer; 1988. p. 351-65. https://doi.org/10.1007/978-94-009-2764-3_32.
-
26.
Marcenaro D, Valkonen JP. Seedborne pathogenic fungi in common bean (Phaseolus vulgaris cv. INTA Rojo) in Nicaragua. PLoS One. 2016;11(12). e0168662. [PubMed ID: 27997595]. [PubMed Central ID: PMC5173241]. https://doi.org/10.1371/journal.pone.0168662.
-
27.
Basak AB, Lee MW. Prevalence and transmission of seed-borne fungi of Maize grown in a farm of Korea. Mycobiology. 2002;30(1). https://doi.org/10.4489/myco.2002.30.1.047.
-
28.
Mancini V, Romanazzi G. Seed treatments to control seedborne fungal pathogens of vegetable crops. Pest Manag Sci. 2014;70(6):860-8. [PubMed ID: 24293285]. https://doi.org/10.1002/ps.3693.
-
29.
Gross H, Loper JE. Genomics of secondary metabolite production by Pseudomonas spp. Nat Prod Rep. 2009;26(11):1408-46. [PubMed ID: 19844639]. https://doi.org/10.1039/b817075b.