Abstract
Background:
In recent years, the widespread use of antibiotics has resulted in increased rates of antibiotic resistance (ABR). Pseudomonas aeruginosa is one of the most important opportunistic pathogens causing hospital-acquired infections. Pseudomonas aeruginosa has continuously increased resistance to commonly used clinical antimicrobial drugs, bringing great difficulties to clinical treatment.Objectives:
This retrospective study investigated the epidemiological characteristics of P. aeruginosa and changes in ABR over a 5-year period at a hospital in Shandong Province, China.Methods:
Pseudomonas aeruginosa strains were collected from 2015 to 2019. The antimicrobial susceptibility testing employed the Kirby-Bauer disk diffusion method and the broth microdilution method (VITEK-2 compact system), according to the guidelines by the Clinical and Laboratory Standards Institute. Data were analyzed using WHONET 5.6 and SPSS V. 21.0 software.Results:
A total of 3,324 P. aeruginosa strains were isolated from clinical specimens (604, 631, 700, 595, and 794 strains from 2015 to 2019, respectively). The highest P. aeruginosa detection rates were from respiratory tract specimens (72.54%). The highest resistance was seen in aztreonam, followed by ciprofloxacin, levofloxacin, and imipenem. The isolation rates for carbapenem-resistant P. aeruginosa (CRPA) and multidrug-resistant P. aeruginosa (MDRPA) ranged from 15.21 - 18.38% and 17.31 - 27.31%, respectively. Also, the isolation rates for extensively drug-resistant P. aeruginosa (XDRPA) ranged from 1.86 - 3.52%.Conclusions:
The main sources of the P. aeruginosa isolates were older adult patients with chronic respiratory diseases. The isolation rates for CRPA, MDRPA, and XDRPA strains decreased over the 5-year period. However, the drug resistance situation remains a serious concern. Hence, continued infection control and antimicrobial stewardship and basic and clinical research on bacterial resistance are essential.Keywords
Pseudomonas aeruginosa Antibiotic Resistance Carbapenem-resistant Pseudomonas aeruginosa (CRPA) Multidrug-resistant Pseudomonas aeruginosa (MDRPA) Extensively Drug-resistant Pseudomonas aeruginosa (XDRPA)
1. Background
Pseudomonas aeruginosa is a non-fermenting Gram-negative bacillus and one of the most common bacteria causing nosocomial infections. Nosocomial infections by P. aeruginosa can cause blood diseases, respiratory diseases, urinary tract infections, immune disorders, and postoperative complications (1, 2). In recent years, the widespread use of antibiotics has resulted in increased rates of antibiotic resistance (ABR). In particular, the rates of ABR among Acinetobacter baumannii and Klebsiella pneumoniae isolates have increased continuously. Among numerous studies conducted on P. aeruginosa, two studies by Hu et al. have reported decreasing ABR trends in this bacterium (1, 2). According to the National Healthcare Safety Network, carbapenem-resistant P. aeruginosa isolates occur more frequently than carbapenem-resistant K. pneumonia isolates. In most countries, the proportion of carbapenem-resistant P. aeruginosa is between 10% and 50% (3, 4). Unfortunately, the development of new drugs is far slower than the rate of ABR emergence, posing major challenges for the clinical treatment of bacterial infections and a significant threat to human health (4, 5). ABR has become one of the largest public health threats of the 21st century. Therefore, monitoring of ABR is critically important.
2. Objectives
Few studies have analyzed ABR of P. aeruginosa isolates in tandem with patients’ clinical characteristics over a long follow-up period (3-5). Therefore, we investigated ABR among inpatient-derived P. aeruginosa isolates and the clinical characteristics of the affected patients in our hospital from 2015 to 2019. Our overall goal was to identify factors related to ABR and any changes in the epidemiology of P. aeruginosa over time.
3. Methods
3.1. Bacterial Isolates
The bacterial strains were collected from outpatients and inpatients from January 2015 to December 2019 at Weifang People’s Hospital, a provincial regional medical center in Shandong Province, China. Only the first strain from each patient was included in this study. All strains were characterized using a VITEK-2 compact instrument (bioMérieux, France) according to the guidelines from the Clinical and Laboratory Standards Institute (CLSI) (6). All strains were isolated on Columbia blood plates (bioMérieux) prior to being characterized. Single colonies were used to make a 0.5 McFarland turbidity standard suspension. We obtained a written consent from all patients and the ethics committee of Weifang People’s Hospital approved the study.
3.2. Antimicrobial Susceptibility Testing
For antimicrobial susceptibility testing, we used the Kirby-Bauer (K-B) disk diffusion method and broth microdilution method (VITEK-2 compact system), as recommended by the guidelines from the CLSI (6). Disk diffusion testing was performed on Muller-Hinton agar (Oxoid, UK) with the following disks: aztreonam (ATM), cefoperazone-sulbactam (CSL), piperacillin (PRL), and meropenem (MEM), according to the guidelines from the CLSI (6). Broth microdilution testing was performed on the VITEK-2 compact system to determine the minimum inhibitory concentrations (MICs) of the following antibiotics: ceftazidime (CAZ), ciprofloxacin (CIP), levofloxacin (LVX), cefepime (FEP), gentamicin (GEN), amikacin (AMK), tobramycin (TOB), piperacillin-tazobactam (TZP), and imipenem (IPM). Isolates shown to be resistant to IPM and/or MEM were defined as carbapenem-resistant P. aeruginosa (CRPA), and those resistant to three or more drugs class were defined as multidrug-resistant P. aeruginosa (MDRPA). Isolates with extensive drug resistance were defined as extensively drug-resistant P. aeruginosa (XDRPA). Pseudomonas aeruginosa ATCC 27853 and Escherichia coli ATCC25922 were used as controls for ABR.
3.3. Data Management and Statistical Analysis
Antimicrobial susceptibility testing data were centrally converted into a standard format using WHONET 5.6 (WHO, Switzerland), which was used for data management. SPSS 21.0 software (SPSS Inc., USA) was used for statistical analysis. Results were presented as numbers (percentages) for categorical variables, and the χ2 test was used to compare them. Statistical significance was set at P < 0.05.
4. Results
4.1. Changes in the Pseudomonas aeruginosa Isolation Rate Over Time and Clinical Characteristics of the Patients
A total of 3,324 P. aeruginosa strains (excluding duplicates) were isolated from clinical specimens from 2015 to 2019, resulting in 604, 631, 700, 595, and 794 strains, respectively. The clinical characteristics of the patients with P. aeruginosa bacteremia are shown in Table 1. During this 5-year period, 65 - 67% of the cases were male. Older adults were the main affected population, among whom those aged 61 - 75 years had the highest percentage (30 - 41%), followed by those aged 46 to 60 (24 - 33%) and older than 75 (18 - 22%). Most isolates from the last five years were sourced from patients with P. aeruginosa pneumonia, the infection rate of which was 72.65% (range, 68.07 - 78.97%).
The second most common infection was soft tissue infection (infection rate, 18.32%; range, 13.08 - 22.52%). The third most common infection was urinary tract infection (infection rate, 3.61%; range, 2.69 - 4.97%). Blood flow-related infection was the fourth most common infection (rate, 3.28%; range, 2.15 - 4.53%). While other infections were less common, the incidence of intensive care unit (ICU)-related infections over the last five years was 11.85% (range, 10.71 - 14.07%). Over the last five years, the percentage of patients with malignancy-related diseases was 29.06% (range, 17.72 - 39.66%), those with cerebrovascular diseases was 22.68% (range, 18.38 - 27.15%), and those with pulmonary diseases was 22.65% (range, 17.12 - 27.81%).
Variables | 2015 (N = 604) | 2016 (N = 631) | 2017 (N = 700) | 2018 (N = 595) | 2019 (N = 794) | Total (N = 3324) |
---|---|---|---|---|---|---|
Sex | ||||||
Male | 407 (67.38) | 417 (66.09) | 463 (66.14) | 394 (66.22) | 520 (65.49) | 2201 (66.22) |
Female | 197 (32.62) | 214 (33.91) | 237 (33.86) | 201 (33.78) | 274 (34.51) | 1123 (33.78) |
Age rang | ||||||
0 - 12 | 20 (3.31) | 29 (4.6) | 20 (2.86) | 19 (3.19) | 26 (3.27) | 114 (3.43) |
13 - 30 | 31 (5.13) | 40 (6.34) | 26 (3.71) | 30 (5.04) | 30 (3.78) | 157 (4.72) |
31 - 45 | 55 (9.11) | 46 (7.29) | 66 (9.43) | 63 (10.59) | 69 (8.69) | 299 (9) |
46 - 60 | 194 (32.12) | 168 (26.62) | 200 (28.57) | 163 (27.39) | 191 (24.06) | 916 (27.56) |
61 - 75 | 183 (30.3) | 233 (36.93) | 253 (36.14) | 190 (31.93) | 318 (40.05) | 1177 (35.41) |
> 75 | 121 (20.03) | 115 (18.23) | 135 (19.29) | 130 (21.85) | 160 (20.15) | 661 (19.89) |
Source of bacteremia | ||||||
Primary bacteremia | 13 (2.15) | 17 (2.69) | 26 (3.71) | 17 (2.86) | 36 (4.53) | 109 (3.28) |
Pneumonia | 477 (78.97) | 474 (75.12) | 494 (70.57) | 405 (68.07) | 565 (71.16) | 2415 (72.65) |
Urinary tract infection | 30 (4.97) | 17 (2.69) | 21 (3) | 18 (3.03) | 34 (4.28) | 120 (3.61) |
Intraabdominal infection | 3 (0.5) | 5 (0.79) | 9 (1.29) | 14 (2.35) | 14 (1.76) | 45 (1.35) |
Soft tissue infection | 79 (13.08) | 110 (17.43) | 146 (20.86) | 134 (22.52) | 140 (17.63) | 609 (18.32) |
Catheter related blood | 2 (0.33) | 6 (0.95) | 2 (0.29) | 6 (1.01) | 4 (0.5) | 20 (0.6) |
Cerebrospinal fluid | 0 (0) | 2 (0.32) | 2 (0.29) | 2 (0.34) | 1 (0.13) | 7 (0.21) |
ICU acquired | 85 (14.07) | 72 (11.41) | 77 (11) | 75 (12.61) | 85 (10.71) | 394 (11.85) |
Underlying disease | ||||||
Malignancy | 107 (17.72) | 126 (19.97) | 206 (29.43) | 236 (39.66) | 291 (36.65) | 966 (29.06) |
Hematologic malignancy | 11 (1.82) | 9 (1.43) | 11 (1.57) | 19 (3.19) | 21 (2.64) | 71 (2.14) |
Solid tumor | 96 (15.89) | 117 (18.54) | 195 (27.86) | 217 (36.47) | 270 (34.01) | 895 (26.93) |
Cerebral vascular stroke | 164 (27.15) | 116 (18.38) | 144 (20.57) | 161 (27.06) | 169 (21.28) | 754 (22.68) |
Liver cirrhosis | 3 (0.5) | 4 (0.63) | 2 (0.29) | 5 (0.84) | 7 (0.88) | 21 (0.63) |
Diabetes mellitus | 8 (1.32) | 8 (1.27) | 9 (1.29) | 13 (2.18) | 18 (2.27) | 56 (1.68) |
Cardiovascular diseases | 37 (6.13) | 23 (3.65) | 46 (6.57) | 53 (8.91) | 71 (8.94) | 230 (6.92) |
Chronic obstructive | 31 (5.13) | 28 (4.44) | 42 (6) | 61 (10.25) | 81 (10.2) | 243 (7.31) |
Pulmonary disease | 168 (27.81) | 108 (17.12) | 133 (19) | 163 (27.39) | 181 (22.8) | 753 (22.65) |
Burn | 29 (4.8) | 27 (4.28) | 29 (4.14) | 33 (5.55) | 39 (4.91) | 157 (4.72) |
Digestive system | 18 (2.98) | 37 (5.86) | 19 (2.71) | 26 (4.37) | 37 (4.66) | 137 (4.12) |
Urinary system | 23 (3.81) | 19 (3.01) | 24 (3.43) | 26 (4.37) | 37 (4.66) | 129 (3.88) |
Orthopaedic diseases | 13 (2.15) | 32 (5.07) | 27 (3.86) | 41 (6.89) | 39 (4.91) | 152 (4.57) |
4.2. Changing Trends in Antimicrobial Susceptibility
The antimicrobial susceptibility in P. aeruginosa is shown in Table 2. Except for the low value for GEN in 2018 from 1 to 0.5 μg/mL, the MIC range for the clinical antibacterial drugs used over the past five years showed no changes. In contrast, the MIC50 values for the antimicrobial drugs showed that LVX decreased from 1 to 0.5 μg/mL in 2016 - 2018 and TZP from 8 to 4 μg/mL after 2016, whereas the MIC50 values of other drugs did not change. While the MIC90 values of the clinical drugs CIP, FEP, and TZP did not change over the last five years, the values for GEN, TOB, and AMK aminoglycosides rose in 2016 and declined in 2017, and IPM decreased in 2016 and increased during 2017 - 2018. The resistance rate (95% confidence interval [CI]) trends over the past five years, except for the expansion of IPM, ATM, and CSL in 2016 - 2017, showed a downwards trend for the other antibiotics, and the overall range had a left shift trend. As Figure 1 shows, over the past five years, almost all the ABR rates of the P. aeruginosa isolates were below 20%, except for those of CIP and ATM in 2015 and 2018, which exceed 20%. The highest ABR rate for the P. aeruginosa isolates from our hospital was for ATM, followed by CIP, LVX, and IPM. While the mid-level resistance rates were for PRL, MEM, and CAZ, those for AMK, FEP, GEN, TOB, and TZP were all below 10%.
Changes in Antimicrobial Susceptibility Trends from 2015 to 2019
Agent and Year | Resistance (R%) | Medium (R%) | Sensitive (R%) | Resistance Rate (95% CI) | MIC50 | MIC90 | District Average | MIC Range | P-Value |
---|---|---|---|---|---|---|---|---|---|
VITEK-2 Compact Broth Micro-dilution Method | |||||||||
AMK | 0.114 | ||||||||
2015 | 6.6 | 1.5 | 91.9 | 4.3 - 10.0 | 2 | 8 | 3.179 | 2 - 64 | |
2016 | 5.9 | 0.6 | 93.5 | 3.7 - 9.1 | 2 | 16 | 3.145 | 2 - 64 | |
2017 | 3.6 | 0.8 | 95.7 | 2.1 - 6.1 | 2 | 8 | 2.964 | 2 - 64 | |
2018 | 6.5 | 0 | 93.5 | 4.3 - 9.7 | 2 | 8 | 2.967 | 2 - 64 | |
2019 | 4.3 | 0.6 | 95.2 | 2.5 - 7.1 | 2 | 8 | 3.064 | 2 - 64 | |
CAZ | 0.045 | ||||||||
2015 | 10.9 | 6.7 | 82.4 | 7.8 - 14.9 | 4 | 32 | 4.269 | 1 - 64 | |
2016 | 7.6 | 10.9 | 81.5 | 5.1 - 11.1 | 4 | 16 | 4.689 | 1 - 64 | |
2017 | 7.4 | 11.5 | 81.1 | 5.1 - 10.6 | 4 | 16 | 4.616 | 1 - 64 | |
2018 | 10.7 | 5.3 | 84 | 7.8 - 14.5 | 4 | 32 | 4.888 | 1 - 64 | |
2019 | 10.6 | 8.9 | 80.5 | 7.7 - 14.4 | 4 | 32 | 5.178 | 1 - 64 | |
CIP | 0.000 | ||||||||
2015 | 20.5 | 5.1 | 74.3 | 16.4 - 25.3 | 0.25 | 4 | 0.59 | .25 - 4 | |
2016 | 19.1 | 7.9 | 72.9 | 15.1 - 23.8 | 0.25 | 4 | 0.596 | .25 - 4 | |
2017 | 13.2 | 6.4 | 80.4 | 10.1 - 17.0 | 0.25 | 4 | 0.484 | .25 - 4 | |
2018 | 16.6 | 3.9 | 79.4 | 13.0 - 21.0 | 0.25 | 4 | 0.485 | .25 - 4 | |
2019 | 12.9 | 4.6 | 82.5 | 9.7 - 17.0 | 0.25 | 4 | 0.455 | .25 - 4 | |
FEP | 0.012 | ||||||||
2015 | 8.1 | 5.4 | 86.4 | 5.5 - 11.7 | 2 | 16 | 2.918 | 1 - 64 | |
2016 | 6.5 | 6.8 | 86.8 | 4.2 - 9.8 | 2 | 16 | 3.236 | 1 - 64 | |
2017 | 4.6 | 6.6 | 88.8 | 2.8 - 7.3 | 2 | 16 | 3.004 | 1 - 64 | |
2018 | 9 | 3.9 | 87.1 | 6.3 - 12.6 | 2 | 16 | 3.28 | 1 - 64 | |
2019 | 8.3 | 4.3 | 87.4 | 5.7 - 11.8 | 2 | 16 | 3.23 | 1 - 64 | |
GEN | 0.000 | ||||||||
2015 | 9.4 | 1.8 | 88.8 | 6.6 - 13.2 | 1 | 8 | 1.443 | 1 - 16 | |
2016 | 10.9 | 3.2 | 85.9 | 7.9 - 14.8 | 1 | 16 | 1.638 | 1 - 16 | |
2017 | 6.1 | 2.8 | 91 | 4.0 - 9.1 | 1 | 4 | 1.536 | 1 - 16 | |
2018 | 6.7 | 7.3 | 86 | 4.4 - 9.9 | 1 | 8 | 1.568 | .5 - 16 | |
2019 | 5.1 | 3.7 | 91.2 | 3.1 - 8.1 | 1 | 4 | 1.556 | 1 - 16 | |
IPM | 0.017 | ||||||||
2015 | 16.6 | 6.9 | 76.5 | 12.8 - 21.1 | 2 | 16 | 2.364 | 1 - 16 | |
2016 | 13.2 | 10.6 | 76.2 | 9.9 - 17.4 | 2 | 8 | 2.179 | 1 - 16 | |
2017 | 17.7 | 1.8 | 80.5 | 13.5 - 22.8 | 2 | 16 | 2.57 | 1 - 16 | |
2018 | 14.2 | 0.4 | 85.4 | 10.4 - 19.0 | 2 | 16 | 2.308 | 1 - 16 | |
2019 | 12.2 | 0.8 | 87.1 | 8.6 - 16.9 | 2 | 8 | 2.148 | 1 - 16 | |
LVX | 0.000 | ||||||||
2015 | 18.1 | 5.7 | 76.2 | 14.2 - 22.8 | 1 | 8 | 1.119 | .25 - 8 | |
2016 | 18.2 | 5.3 | 76.5 | 14.3 - 22.8 | 0.5 | 8 | 1.101 | .25 - 8 | |
2017 | 11.2 | 5.6 | 83.2 | 8.3 - 14.8 | 0.5 | 8 | 0.938 | .25 - 8 | |
2018 | 14.6 | 4.2 | 81.2 | 11.2 - 18.8 | 0.5 | 8 | 0.907 | .25 - 8 | |
2019 | 9.5 | 4.9 | 85.7 | 6.7 - 13.2 | 1 | 4 | 0.913 | .25 - 8 | |
TOB | 0.000 | ||||||||
2015 | 8.7 | 0.9 | 90.4 | 6.0 - 12.4 | 1 | 4 | 1.342 | 1 - 16 | |
2016 | 10.9 | 1.2 | 87.9 | 7.9 - 14.8 | 1 | 16 | 1.435 | 1 - 16 | |
2017 | 6.1 | 0.5 | 93.4 | 4.0 - 9.1 | 1 | 1 | 1.225 | 1 - 16 | |
2018 | 10.4 | 0.8 | 88.8 | 7.5 - 14.2 | 1 | 16 | 1.392 | 1 - 16 | |
2019 | 5.7 | 1.7 | 92.6 | 3.6 - 8.8 | 1 | 2 | 1.25 | 1 - 16 | |
TZP | 0.002 | ||||||||
2015 | 7.9 | 14.5 | 77.6 | 5.3 - 11.5 | 8 | 64 | 9.767 | 4 - 128 | |
2016 | 6.2 | 16.9 | 76.9 | 4.0 - 9.5 | 4 | 64 | 9.484 | 4 - 128 | |
2017 | 3.5 | 13.9 | 82.6 | 2.0 - 6.1 | 4 | 64 | 8.198 | 4 - 128 | |
2018 | 8.2 | 7.9 | 84 | 5.5 - 11.9 | 4 | 64 | 8.266 | 4 - 128 | |
2019 | 8.2 | 5.5 | 86.3 | 5.4 - 12.1 | 4 | 64 | 7.795 | 4 - 128 | |
K-B Method | |||||||||
ATM | 0.130 | ||||||||
2015 | 21.3 | 19.1 | 59.6 | 17.3 - 25.9 | |||||
2016 | 17.9 | 13.9 | 68.2 | 12.3 - 25.2 | |||||
2017 | 16.9 | 17.1 | 66 | 13.6 - 20.8 | |||||
2018 | 21.1 | 13.2 | 65.8 | 17.3 - 25.5 | |||||
2019 | 18 | 14.4 | 67.5 | 14.4 - 22.3 | |||||
CSL | 0.081 | ||||||||
2015 | 10.4 | 17.3 | 72.3 | 7.5 - 14.1 | |||||
2016 | 9.8 | 13.4 | 76.8 | 7.1 - 13.3 | |||||
2017 | 13.2 | 13.2 | 73.5 | 8.4 - 19.9 | |||||
2018 | 9.8 | 15.5 | 74.7 | 7.1 - 13.3 | |||||
2019 | 8.8 | 14 | 77.2 | 6.2 - 12.3 | |||||
MEM | 0.061 | ||||||||
2015 | 11.9 | 2.4 | 85.8 | 8.7 - 16.0 | |||||
2016 | 7.5 | 2.3 | 90.2 | 5.2 - 10.7 | |||||
2017 | 9.2 | 1.8 | 89 | 6.8 - 12.4 | |||||
2018 | 10.7 | 2.5 | 86.8 | 7.9 - 14.2 | |||||
2019 | 8.7 | 1.5 | 89.8 | 6.2 - 12.0 | |||||
PIP | 0.116 | ||||||||
2015 | 13.4 | 0 | 86.6 | 10.2 - 17.4 | |||||
2016 | 15.7 | 0 | 84.3 | 12.4 - 19.7 | |||||
2017 | 12.1 | 0 | 87.9 | 9.3 - 15.6 | |||||
2018 | 16.6 | 0 | 83.4 | 13.2 - 20.7 | |||||
2019 | 15.7 | 0 | 84.3 | 12.3 - 19.7 |
Trends in Pseudomonas aeruginosa antibiotic resistance rates from 2015 to 2019
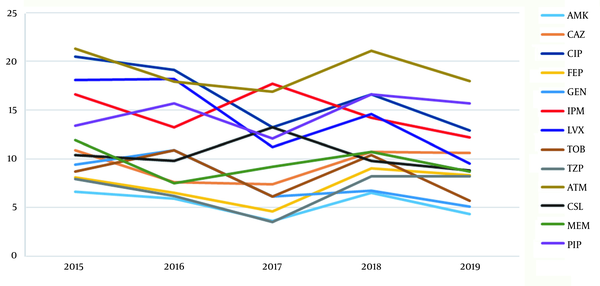
4.3. Drug Sensitivity and Changes in CRPA, MDRPA, and XDRPA
By analyzing the clinical isolates for CRPA (Figure 2), we found that the drug resistance rates of the CRPA strains between 2015 and 2019 were 18.31%, 15.21%, 15.29%, 17.11%, and 15.74%, respectively. No statistical differences were seen for the values between 15% and 20%. The resistance rates for the MDRPA strains isolated between 2015 and 2019 were 25.99%, 27.31%, 21%, 17.31%, and 18.51%, respectively. The overall downwards trend, especially in 2018, was not statistically significant, even though it rose in 2019. The resistance rates for the XDRPA strains isolated between 2015 and 2019 were 15.73, 17.59%, 13%, 12.1%, and 10.2%, respectively (P > 0.05). After 2016, the trend decreased annually, indicating a statistically significant decrease.
Resistance trends for CRPA, MDRPA, and XDRPA from 2015 to 2019
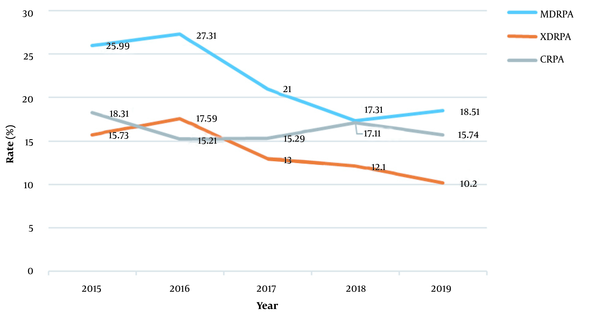
5. Discussion
According to the US Centers for Disease Control and Prevention (CDC), P. aeruginosa accounted for 7.1% of hospital infections in 2011 (6, 7), a value not too dissimilar from that of the EPINE survey in Spain where P. aeruginosa was the second most common cause of hospital-acquired infections (10.5%) in 2016 (8). The increased deterioration of lung function seen in patients with chronic P. aeruginosa infections indicates that this bacterium causes high morbidity and mortality (9, 10). By analyzing the infection characteristics of P. aeruginosa at our hospital over the past five years, we found that the isolates showed an increasing trend of ABR, which is consistent with the trends for P. aeruginosa in China and other countries (11, 12). The characteristics of the infected patients showed that the proportion of male patients was larger than that of females, especially for elderly males over 60 years of age (ABR range, 48 - 63%), indicating that P. aeruginosa infection plays a very important role in infections in elderly males. This finding is consistent with other recent studies from around the world (13, 14).
Data from the isolates and patients over the last five years showed that infections caused by P. aeruginosa were mainly pneumonia (infection rate range, 68.07 - 78.97%). Soft tissue infections came next (infection rate range, 13.08 - 22.52%), followed by urinary tract infections (infection rate range, 2 - 4.97%) and blood flow-related infections (infection rate range, 2.15 - 4.53%). The incidence of ICU infections over the same period was 10.71 - 14.07%. Because P. aeruginosa is a conditional pathogenic bacterium, the normal respiratory tract and digestive tract, among other anatomical sites, can be colonized, especially in the elderly and in patients with poor immunity and susceptibility to opportunistic infections. Hence, the proportion of elderly patients with pulmonary infections was the highest in our hospital, a finding consistent with the data from China and abroad (1, 5). In this study, soft tissue infections were the second commonest source of isolates, a finding similar to the data of Li et al. (15), but different from the data by other studies (16-20). This discrepancy might be caused by the presence of P. aeruginosa in the natural environment, or by patients with skin and mucous membrane damage such as burns from surgery, and other patients are also prone to nosocomial soft tissue infections.
The data from the P. aeruginosa-infected patients showed that the proportion with malignant tumors was nearly 30%, while those with cerebrovascular diseases and pulmonary diseases exceeded 20%. Pseudomonas aeruginosa infections were closely related to the immunity status and activity of the patients, and in this respect they are similar to the study by Huang et al. in the Taiwan Province of China (5). Thus, P. aeruginosa infections are mostly related to respiratory diseases, and this type of infection was at the forefront of ICU, a finding consistent with those of Huang et al. (5) and Feng et al. (21). Such infections are especially common in people with chronic diseases and in elderly patients may be related to many factors, such as decreased immunity in the elderly, long hospitalization times, and more interventional treatments (1, 21-23).
Table 1 shows that the MIC range of the clinical drugs did not change over the past five years. This indicates that, in terms of drug concentration, the antibiotic sensitivity range of P. aeruginosa was stable. The changing trend in MIC50 and MIC90 values was also mainly stable over time, except that the values for some of the drugs decreased. According to the changing trend for all the resistance rates (95% CI) across the past five years, the range had a downwards trend. Comparison of three values (resistance 95% CI, MIC50, and MIC90) and the average values for the hospital district from 2015 to 2019 showed that the change in drug resistance for all drug types had little fluctuations, and the overall drug resistance rate showed a downwards trend (5).
Drug sensitivity testing showed that the resistance rates were almost all lower than 20% during the study period, except that the drug resistance rates for CIP and ATM in 2015 and 2018 exceeded 20% against an overall decreasing trend. The drug resistance rate decreased significantly after 2016. The highest overall resistance rate was for ATM, followed by CIP and LVX, and the lowest drug resistance rate was for AMK, followed by TZP, and TOB. Our results showed that P. aeruginosa was more resistant to ATM and quinolones, but was more sensitive to PRL, TZP, and aminoglycosides, a finding mainly consistent with the monitoring data from CHINET reported by Hu et al. (1, 2). Another study (3) reported that the resistance rate of P. aeruginosa to AMK is low in Chongqing and other parts of China, which is perhaps related to the substrate specificity of aminoglycoside-modifying enzyme-mediated drug resistance, and the frequency of using this drug by clinicians is also lower than that for other drugs. Because of the high nephrotoxicity of AMK, it is mainly used in combination with other antibiotics to treat P. aeruginosa infections to reduce possible side effects. In our previous study (24), we found that the most resistant genes in the variable region of the class I integrons are aminoglycoside resistance genes, which suggests that antibiotics should be used in combination to prevent drug resistance.
By analyzing changes in the resistance rate of P. aeruginosa to the same drugs across different years, we found that the drug resistance rate for ATM between 2016 and 2019 was significantly lower than that in 2015 (P < 0.05). The resistance rate for IPM was also lower than that in 2015, but there was no significant difference (P > 0.05). The remaining 10 drugs showed a trend of having the lowest resistance rates in 2019, which is consistent with previous studies (3-5, 15). This might be related to the antibiotic stewardship initiative campaign launched by the Chinese government in 2016 (3). It is generally believed that carbapenem resistance in P. aeruginosa is mainly related to the production of carbapenase and deletion or changes in the OprD2 outer membrane protein (14). It has been reported that the carbapenem resistance rate in P. aeruginosa is positively correlated with the use of such drugs.
The resistance rate for the P. aeruginosa clinical isolates was significantly lower than that for the unrestricted use of carbapenem antibiotics (25). In addition, some studies have shown that the primary bacteremia infection rate caused by XDRPA is higher than that caused by non-XDRPA (19). Although the XDRPA infection rate in our hospital was maintained below 5% in recent years, its continued monitoring is warranted. By analyzing the resistant patterns of P. aeruginosa, we found that CRPA strains in the past five years remained at 15 - 20%. MDRPA strains and XDRPA strains decreased from 27.31 to 18.51% and from 17.59% to 10.2%, respectively (Figure 2). Although the rates for these isolates were similar to those reported previously, the rates showed a statistically significant decrease, which did not correspond with those from other studies (25-30). Essentially, the trend appears to be a function of the Chinese government’s antibiotic stewardship initiative campaign.
5.1. Conclusions
The main sources of P. aeruginosa isolates in this study were elderly patients with chronic respiratory diseases. Pseudomonas aeruginosa resistance rates for 12 commonly used antibiotics decreased over time. According to the obtained results, the isolation rate for CRPA, MDRPA, and XDRPA strains decreased over the past five years. It was a surprising discovery, while the drug resistance situation remains problematic. This study combined the ABR analysis and the pathogenic characteristics of P. aeruginosa. However, this was a single-center retrospective study. In the future, we plan to conduct a multicenter study to compare differences in ABR and the pathogenic characteristics of P. aeruginosa.
References
-
1.
Hu F, Guo Y, Yang Y, Zheng Y, Wu S, Jiang X, et al. Resistance reported from China antimicrobial surveillance network (CHINET) in 2018. Eur J Clin Microbiol Infect Dis. 2019;38(12):2275-81. [PubMed ID: 31478103]. https://doi.org/10.1007/s10096-019-03673-1.
-
2.
Hu FP, Guo Y, Zhu D-M, Wang F, Jiang X-F, Xu Y-C, et al. Resistance trends among clinical isolates in China reported from CHINET surveillance of bacterial resistance, 2005–2014. Clin Microbiol Infect. 2016;22:9-14.
-
3.
Feng W, Huang Q, Wang Y, Yuan Q, Li X, Xia P, et al. Changes in the resistance and epidemiological characteristics of Pseudomonas aeruginosa during a ten-year period. J Microbiol Immunol Infect. 2021. [PubMed ID: 31628088]. https://doi.org/10.1016/j.jmii.2019.08.017.
-
4.
Liu L, Liu B, Li Y, Zhang W. Successful control of resistance in Pseudomonas aeruginosa using antibiotic stewardship and infection control programs at a Chinese university hospital: a 6-year prospective study. Infect Drug Resist. 2018;11:637-46. [PubMed ID: 29750044]. [PubMed Central ID: PMC5936004]. https://doi.org/10.2147/IDR.S163853.
-
5.
Huang YC, Wu PF, Lin YT, Wang FD. Comparison of clinical characteristics of bacteremia from Elizabethkingia meningoseptica and other carbapenem-resistant, non-fermenting Gram-negative bacilli at a tertiary medical center. J Microbiol Immunol Infect. 2019;52(2):304-11. [PubMed ID: 30097388]. https://doi.org/10.1016/j.jmii.2018.06.007.
-
6.
Clinical and Laboratory Standards Institute. Performance Standards for Antimicrobial Susceptibility Testing; Twenty ninth informational supplement. Clin Lab Stand Ins. 2019.
-
7.
Katoh H, Yasumoto H, Shimizu M, Hamaoka S, Kinoshita M, Akiyama K. IV immunoglobulin for acute lung injury and bacteremia in Pseudomonas aeruginosa pneumonia. Crit Care Med. 2016;44. e12e24. [PubMed ID: 26317571]. https://doi.org/10.1097/CCM.0000000000001271.
-
8.
Ruiz-Garbajosa P, Cantón R. Epidemiology of antibiotic resistance in Pseudomonas aeruginosa. Implications for empiric and definitive therapy. Rev Esp Quimioter. 2017;30(Suppl 1):8-12. [PubMed ID: 28882007].
-
9.
Lam JC, Somayaji R, Surette MG, Rabin HR, Parkins MD. Reduction in Pseudomonas aeruginosa sputum density during a cystic fibrosis pulmonary exacerbation does not predict clinical response. BMC Infect Dis. 2015;15:145. [PubMed ID: 25887462]. [PubMed Central ID: PMC4392784]. https://doi.org/10.1186/s12879-015-0856-5.
-
10.
Chin M, De Zoysa M, Slinger R, Gaudet E, Vandemheen KL, Chan F, et al. Acute effects of viral respiratory tract infections on sputum bacterial density during CF pulmonary exacerbations. J Cyst Fibros. 2015;14(4):482-9. [PubMed ID: 25544473]. [PubMed Central ID: PMC7105172]. https://doi.org/10.1016/j.jcf.2014.11.009.
-
11.
Djordjevic ZM, Folic MM, Jankovic SM. Previous antibiotic exposure and antimicrobial resistance patterns of Acinetobacter spp. and Pseudomonas aeruginosa isolated from patients with nosocomial infections. Balkan Med J. 2017;34(6):527-33. [PubMed ID: 29215335]. [PubMed Central ID: PMC5785657]. https://doi.org/10.4274/balkanmedj.2016.1844.
-
12.
Djordjevic Z, Folic MM, Zivic Z, Markovic V, Jankovic SM. Nosocomial urinary tract infections caused by Pseudomonas aeruginosa and Acinetobacter species: sensitivity to antibiotics and risk factors. Am J Infect Control. 2013;41(12):1182-7. [PubMed ID: 23726656]. https://doi.org/10.1016/j.ajic.2013.02.018.
-
13.
Aloush V, Navon-Venezia S, Seigman-Igra Y, Cabili S, Carmeli Y. Multidrug-resistant Pseudomonas aeruginosa: risk factors and clinical impact. Antimicrob Agents Chemother. 2006;50(1):43-8. [PubMed ID: 16377665]. [PubMed Central ID: PMC1346794]. https://doi.org/10.1128/AAC.50.1.43-48.2006.
-
14.
Zavascki AP, Carvalhaes CG, Picao RC, Gales AC. Multidrug-resistant Pseudomonas aeruginosa and Acinetobacter baumannii: resistance mechanisms and implications for therapy. Expert Rev Anti Infect Ther. 2010;8(1):71-93. [PubMed ID: 20014903]. https://doi.org/10.1586/eri.09.108.
-
15.
Li L, Dai JX, Xu L, Chen ZH, Li XY, Liu M, et al. Antimicrobial resistance and pathogen distribution in hospitalized burn patients: A multicenter study in Southeast China. Medicine (Baltimore). 2018;97(34). e11977. [PubMed ID: 30142829]. [PubMed Central ID: PMC6113017]. https://doi.org/10.1097/MD.0000000000011977.
-
16.
European Centre for Disease Prevention and Control. Antimicrobial resistance surveillance in Europe 2015: Annual Report of the European Antimicrobial Resistance Surveillance Network (EARS-Net). Stockholm. 2017.
-
17.
Cabot G, Ocampo-Sosa AA, Tubau F, Macia MD, Rodriguez C, Moya B, et al. Overexpression of AmpC and efflux pumps in Pseudomonas aeruginosa isolates from bloodstream infections: prevalence and impact on resistance in a Spanish multicenter study. Antimicrob Agents Chemother. 2011;55(5):1906-11. [PubMed ID: 21357294]. [PubMed Central ID: PMC3088238]. https://doi.org/10.1128/AAC.01645-10.
-
18.
Sader HS, Farrell DJ, Flamm RK, Jones RN. Antimicrobial susceptibility of Gram-negative organisms isolated from patients hospitalised with pneumonia in US and European hospitals: results from the SENTRY Antimicrobial Surveillance Program, 2009-2012. Int J Antimicrob Agents. 2014;43(4):328-34. [PubMed ID: 24630306]. https://doi.org/10.1016/j.ijantimicag.2014.01.007.
-
19.
Montero M, Sala M, Riu M, Belvis F, Salvado M, Grau S, et al. Risk factors for multidrug-resistant Pseudomonas aeruginosa acquisition. Impact of antibiotic use in a double case-control study. Eur J Clin Microbiol Infect Dis. 2010;29(3):335-9. [PubMed ID: 20033749]. https://doi.org/10.1007/s10096-009-0850-1.
-
20.
Fernandez-Barat L, Ferrer M, De Rosa F, Gabarrus A, Esperatti M, Terraneo S, et al. Intensive care unit-acquired pneumonia due to Pseudomonas aeruginosa with and without multidrug resistance. J Infect. 2017;74(2):142-52. [PubMed ID: 27865895]. https://doi.org/10.1016/j.jinf.2016.11.008.
-
21.
Feng W, Sun F, Wang Q, Xiong W, Qiu X, Dai X, et al. Epidemiology and resistance characteristics of Pseudomonas aeruginosa isolates from the respiratory department of a hospital in China. J Glob Antimicrob Resist. 2017;8:142-7. [PubMed ID: 28216097]. https://doi.org/10.1016/j.jgar.2016.11.012.
-
22.
Haeili M, Ghodousi A, Nomanpour B, Omrani M, Feizabadi MM. Drug resistance patterns of bacteria isolated from patients with nosocomial pneumonia at Tehran hospitals during 2009-2011. J Infect Dev Ctries. 2013;7(4):312-7. [PubMed ID: 23592640]. https://doi.org/10.3855/jidc.2604.
-
23.
Yayan J, Ghebremedhin B, Rasche K. Antibiotic resistance of Pseudomonas aeruginosa in pneumonia at a single university hospital center in Germany over a 10-year period. PLoS One. 2015;10(10). e0139836. [PubMed ID: 26430738]. [PubMed Central ID: PMC4592231]. https://doi.org/10.1371/journal.pone.0139836.
-
24.
Liu M, Ma J, Jia W, Li W. Antimicrobial resistance and molecular characterization of gene cassettes from class 1 integrons in Pseudomonas aeruginosa strains. Microb Drug Resist. 2020;26(6):670-6. [PubMed ID: 32407190]. [PubMed Central ID: PMC7307683]. https://doi.org/10.1089/mdr.2019.0406.
-
25.
Pakyz AL, Oinonen M, Polk RE. Relationship of carbapenem restriction in 22 university teaching hospitals to carbapenem use and carbapenem-resistant Pseudomonas aeruginosa. Antimicrob Agents Chemother. 2009;53(5):1983-6. [PubMed ID: 19273670]. [PubMed Central ID: PMC2681502]. https://doi.org/10.1128/AAC.01535-08.
-
26.
Horcajada JP, Montero M, Oliver A, Sorli L, Luque S, Gomez-Zorrilla S, et al. Epidemiology and treatment of multidrug-resistant and extensively drug-resistant Pseudomonas aeruginosa infections. Clin Microbiol Rev. 2019;32(4). [PubMed ID: 31462403]. [PubMed Central ID: PMC6730496]. https://doi.org/10.1128/CMR.00031-19.
-
27.
Pena C, Cabot G, Gomez-Zorrilla S, Zamorano L, Ocampo-Sosa A, Murillas J, et al. Influence of virulence genotype and resistance profile in the mortality of Pseudomonas aeruginosa bloodstream infections. Clin Infect Dis. 2015;60(4):539-48. [PubMed ID: 25378459]. https://doi.org/10.1093/cid/ciu866.
-
28.
Walkty A, Lagace-Wiens P, Adam H, Baxter M, Karlowsky J, Mulvey MR, et al. Antimicrobial susceptibility of 2906 Pseudomonas aeruginosa clinical isolates obtained from patients in Canadian hospitals over a period of 8 years: Results of the Canadian Ward surveillance study (CANWARD), 2008-2015. Diagn Microbiol Infect Dis. 2017;87(1):60-3. [PubMed ID: 28336136]. https://doi.org/10.1016/j.diagmicrobio.2016.10.003.
-
29.
Sader HS, Castanheira M, Duncan LR, Flamm RK. Antimicrobial susceptibility of Enterobacteriaceae and Pseudomonas aeruginosa isolates from United States medical centers stratified by infection type: results from the international network for optimal resistance monitoring (INFORM) surveillance program, 2015-2016. Diagn Microbiol Infect Dis. 2018;92(1):69-74. [PubMed ID: 29789189]. https://doi.org/10.1016/j.diagmicrobio.2018.04.012.
-
30.
Magill SS, Edwards JR, Bamberg W, Beldavs ZG, Dumyati G, Kainer MA, et al. Multistate point-prevalence survey of health care-associated infections. N Engl J Med. 2014;370(13):1198-208. [PubMed ID: 24670166]. [PubMed Central ID: PMC4648343]. https://doi.org/10.1056/NEJMoa1306801.