Abstract
Background:
The quick diagnosis and early initiation of antibiotic therapy in bacteria-induced infections is of paramount importance. Accordingly, the rapid identification of the causative agent, the short-term results of antibiotic sensitivity, the selection and use of right antibiotics for treatment further highlights the significance of this issue.Objectives:
This study aimed to develop a new susceptibility testing method to provide rapid results in Escherichia coli clinical isolates and report the antibiotic susceptibility test results to clinicians in a short period.Methods:
In the study, one hundred and ten E. coli clinical isolates were tested. In this regard, antibiotics recommended by the "Clinical and Laboratory Standards Institute (CLSI)" for testing the sensitivity of E. coli isolates, including amoxicillin-clavulanate, cefixime, ceftriaxone, ertapenem, ciprofloxacin, gentamicin, trimethoprim-sulfamethoxazole, and nitrofurantoin were tested. For quality control, E. coli ATCC25922, E. coli ATCC35218, Staphylococcus aureus ATCC29213, and E. coli 13846NTCC strains were used. The broth microdilution method recommended by CLSI was used as the reference method. Minimum inhibitory concentration values were determined, and antimicrobial susceptibilities were then determined according to the “European Committee on Antimicrobial Susceptibility Testing (EUCAST)” criteria. In the next phase, the results of the resazurin microplate method (RMM) were compared.Results:
The comparison of the RMM developed in the present study with the reference method revealed that the calculated essential agreement ratios for eight antibiotics varied from 82.72 to 100%, and the categorical agreement values ranged from 95.45 to 100%.Conclusions:
According to the findings, the RMM results were highly in agreement with the results of the reference method. RMM allows the detection of antibiotic susceptibility quickly (e.g., within 5 hours) as such it is preferred, especially for laboratories with limited facilities. However, further multi-center studies are recommended to use this method in routine laboratories.Keywords
1. Background
Escherichia coli is a facultative anaerobe bacteria found in the human digestive system, and it is the most frequently isolated pathogen of the Enterobacteriaceae family (1). Escherichia coli is one of the most common bacteria worldwide, which may cause serious diseases, including urinary tract infection, bloodstream infection, skin infection, otitis media, and diarrhea. Antimicrobial resistance in E. coli is a fundamental problem for the healthcare system, which complicates treatment outcomes, increases treatment costs, and limits treatment options. As documented in many previous studies, E. coli is becoming highly resistant to new and old drugs (2). Antimicrobial resistance poses a significant threat to human health. If the antimicrobial resistance is not controlled, its increase and the "post-antibiotic era" are predicted (3).
The early diagnosis and initiation of antibiotic treatment are of great significance in bacterial infections. Colorimetric methods provide the minimum inhibitory concentration (MIC) values and susceptibility status of antimicrobials in a short time with less material. They need no device and can be easily applied in many laboratories. The main disadvantages of colorimetric methods are difficulty in obtaining antibiotic powders and sometimes in evaluating color change in tests. Accordingly, it is important for the clinician to get antimicrobial susceptibility results quickly for accurate and effective treatment.
2. Objectives
This study aims to develop a new susceptibility testing method providing rapid results and reporting the antibiotic susceptibility test results of E. coli isolates to the clinician quickly.
3. Methods
3.1. Tested Isolates
The study used one hundred and ten clinical isolates of E. coli isolated from clinical samples coming to the Microbiology Laboratory of Ondokuz Mayis University the Medical Faculty Hospital during January - October 2019. The isolates were detected using conventional methods (Gram staining, colony morphology on 5% sheep blood agar and eosin methylene blue agar plates) and MALDI-TOF MS (Vitek MS, Biomeriux, France). E. coli ATCC25922, E. coli ATCC35218 (as control microorganism for beta-lactamase inhibitor combinations containing the only clavulanate), Staphylococcus aureus ATCC 29213, and E. coli 13846NTCC strains were used for quality control. The study isolates were selected from intensive care and other inpatient specimens, considering their susceptibility or resistance to selected antibiotics. Attempts were made to select the susceptibility results of the isolates in numbers close to each other.
3.2. Antibiotics and Chemicals
In the study, one of the antibiotic groups recommended by the Clinical and Laboratory Standards Institute (CLSI) for Enterobacteriaceae and eight antibiotics, one parenteral and one oral, were selected from cephalosporins to be used in the study (4). Amoxicillin, ertapenem, ciprofloxacin, trimethoprim, sulfamethoxazole, nitrofurantoin (Sigma-Aldrich, Germany), gentamicin, clavulanate, cefixime, and ceftriaxone (Pharmaceutical Sciences, Turkey) were also tested. Antibiotics were supplied in the form of commercial powder by the manufacturer, and the required amount (4096 μg/mL) was prepared according to the CLSI guideline according to the potency specified in the analysis certificates. It was stored at -80°C to be used later (4). Resazurin (Sigma-Aldrich, Germany) stock solution was prepared at 0.02%, sterilized by passing through a 0.22 μm membrane filter, and stored at 4°C to be used later (4).
3.3. Susceptibility Tests
Susceptibility tests were performed in Cation-Adjusted Mueller-Hinton Broth (CAMHB). The antimicrobials in the were tested at concentration as follows: Amoxicillin-clavulanate 64 - 1 μg/mL, cefixime 8 - 0.125 μg/mL, ceftriaxone 8 - 0.125 μg/mL, ertapenem 4 - 0.06 μg/mL, ciprofloxacin 2 - 0.03 μg/mL, gentamicin 16 - 0.25 μg/mL, trimethoprim-sulfamethoxazole 16 - 0.25 μg/mL, and nitrofurantoin 256 - 4 μg/mL. Broth microdilution (BMD) tests were performed in 96-well U bottom plates. The reference BMD and the Resazurin Microplate Method (RMM) were applied in CAMHB. The indicated concentrations were prepared to be tested for each antibiotic in two-fold dilutions. Two plates, including a reference BMD and an RMM, were prepared for each antibiotic. The plates were stored at -80°C to be used later. The first well was used as the growth control well, and the last well was the sterility control well.
3.4. Reference Method
The BMD method recommended by CLSI was used as a reference method in the study. The pre-made BMD plates were taken out from -80°C before use, thawed, and brought to room temperature, and the test was applied according to the CLSI recommendations. According to the European Committee on Antimicrobial Susceptibility Testing (EUCAST) breakpoints, the results were defined as susceptible or resistant (4, 5).
3.5. Validation
A preliminary study was conducted on the test validation. This study aimed to determine the incubation time after adding bacterial suspension, dilution rate, and resazurin solution to be used in applying the test according to the reference method. Test plates were prepared for antibiotic stock, resazurin stock, and eight antibiotics. Then 50 μL of 0.5, 1, 2, 3 McFarland turbidity bacterial inoculum was inoculated into in each well as undiluted, 1: 10 dilution, 1: 100 dilution, and 1: 1000 dilutions. The plates were incubated at 35°C. During the 4th hour of their incubation, 30 µL of 0.02% resazurin solution was added to the wells, and the plates were incubated for an additional 1 hour. When the color changed from blue to red in the first well (i.e., the growth control well), the test was evaluated, and the minimum inhibitory concentration (MIC) values were determined. Since color transformation was completed during 1 hour, there was no need to extend the incubation time using the resazurin solution. The last well without growth (the last well containing no red color) was considered as the MIC value. The antibiotics were tested with a McFarland 0.5 turbidity 1: 10 diluted bacterial inoculum, and the same MIC values (in ± 1 dilution) among the tested bacterial inoculums were obtained by the reference method.
3.6. Resazurin Microplate Method
The test was performed after the previously prepared test plates with antibiotics were taken out from -80°C before testing and brought to room temperature. As in the application of the reference method, the bacterial inoculum was prepared directly from the culture of fresh bacteria grown on blood agar using the colony suspension method, and McFarland 0.5 in saline was adjusted to turbidity. The bacterial suspension, whose turbidity was adjusted according to the McFarland 0.5 standard, was inoculated with CAMHB in 1:10 dilutions to 50 µl in all wells, including growth control wells. The plates were incubated at 35°C for 4 hours. During the 4th hour of incubation, 30 µL of blue-colored 0.02% resazurin solution was added to the wells, and the plates were incubated for an additional 1 hour. At the end of the incubation process, if the color changed from blue to red in the first well (i.e., the growth control well), the test was evaluated, and the MIC values were determined. The last well without growth (the last well containing no red color) was considered as the MIC value (Figure 1). According to the EUCAST breakpoints, the results were defined as susceptible or resistant (5). Further, inconsistent results obtained by the reference method and RMM were repeated.
Resazurin test plate for trimethoprim/sulfamethoxazole
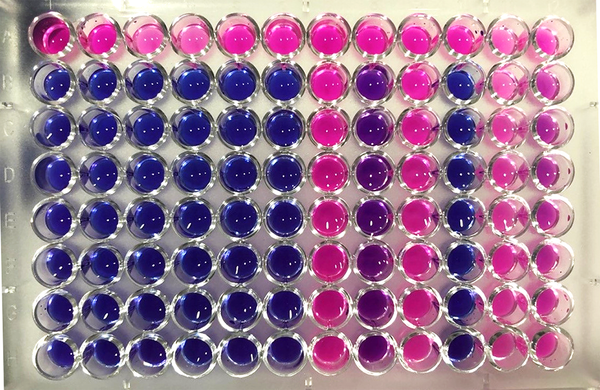
3.7. Statistical Method
A power analysis was performed to determine the number of clinical isolates included in the study. Considering the results of similar studies, when the Type I error was 5%, the study's power was 80% in the calculations in the Minitab program. The number of samples (preparations) required to show the expected frequency of 81.2% and the difference of 0.17 units was 101 samples. In this regard, given the possible data attrition, the number of samples was determined to be at least 106, by increasing the information about 5%, 110 samples were finally included in this study. According to the 'U.S. Food and Drug Administration (FDA)' criteria, the results were evaluated. Accordingly, essential agreement (EA), categorical agreement (CA), very major error (VME), major error (ME), and minor error (mE) were calculated (6).
4. Results
4.1. Isolates
A total of 110 E. coli clinical isolates were used in our study. Eight isolates were isolated from intensive care units, and 102 isolates were taken from samples sent from 22 non-intensive care units. Table 1 shows the distribution of isolated sent from intensive care units and departments.
Distribution of Isolates from Intensive Care/Non-intensive Care
Isolates | Pediatric/Adult Intensive Care | Non-intensive Care | Total |
---|---|---|---|
Urine | 3 | 72 | 75 |
Wound swab | - | 12 | 12 |
Blood | 1 | 5 | 6 |
Abscess | - | 4 | 4 |
Surgical material | - | 4 | 4 |
Tracheal aspirate | 3 | - | 3 |
Sputum | - | 2 | 2 |
Body fluid | 1 | 1 | 2 |
Peritoneal fluid | - | 2 | 2 |
Total | 8 | 102 | 110 |
4.2. Results of Validation Study
The susceptibility results for eight antibiotics and four reference strains tested for the test validation were 100% compatible with the results of the newly-developed RMM. Moreover, the MIC values obtained in tests performed with quality control strains were found to be in the range of acceptable values reported in the CLSI standards.
4.3. Antimicrobial Susceptibility Tests Results
In this study, the MIC results obtained by the BMD method and RMM in 110 clinical isolates for eight antibiotics were evaluated according to the EUCAST breakpoints. When ciprofloxacin results were evaluated according to the FDA criteria, using the automated system, two isolates were determined to be resistant, both of which were found to be susceptible by the reference method and RMM. While one isolate was found to be susceptible by the automated system, it was resistant as both the reference method and RMM determined. One isolate was reported as susceptible by the automated system and RMM; however, it was considered as resistant by the reference method. Another isolate was determined to be susceptible by the automated system and reference method; however, it was resistant as RMM determined. While two isolates were found to be intermediate by the automated system, one of the isolates was susceptible, as determined by both the reference method and RMM, and another one was resistant as both methods specified.
When gentamicin results were evaluated, while three out of the six isolates were reported as intermediate by the automated system, they were resistant by both the reference method and RMM, the other three isolates were susceptible by both the reference method and RMM. Three isolates were detected as susceptible by the automated system; however, they were resistant as both the reference method and RMM determined. Two isolates were resistant using both the automated system and the reference method; however, RMM reported them as susceptible. The automated system determined an isolate to be resistant, and both the reference method and RMM considered it as susceptible. Although an isolate was found to be susceptible by both the automated system and the reference method, it was resistant in RMM.
Regarding ertapenem, four isolates were susceptible in the automated system. However, they were resistant as the reference method and RMM determined. Although an isolate was found to be resistant by both the automated system and the reference method, it was determined susceptible by the RMM. Three isolates were determined intermediate by the automated system, one of which was considered as resistant by both the reference method and RMM, the other two isolates were found to be susceptible by the reference method and RMM.
Considering ceftriaxone, two isolates were susceptible in the automated system, they were determined as resistant by both the reference method and RMM. Regarding trimethoprim/sulfamethoxazole, seven isolates were susceptible in the automated system; however, they were determined as resistant by both the reference method and RMM. One isolate was reported to be resistant by the automated system; however, it was susceptible by the reference method and the RMM. For nitrofurantoin susceptibility, six isolates were determined as resistant by the automated system. This was while these isolates were susceptible as both the reference method and RMM reported. Moreover, five isolates were found to be resistant by both the automated system and the reference method; however, they were determined as susceptible by RMM. Moreover, one isolate was found to be susceptible by the automated system and resistant by both the reference method and RMM.
In the study, five isolates were resistant to cefixime as reported by the automated system and susceptible as reported by both the reference method and RMM. One isolate was susceptible as determined by the automated system, it was resistant using both the reference method and RMM. Moreover, one isolate was found to be resistant by both the automated system and the reference method, it was susceptible by RMM. For the tested isolates, five isolates were reported to be resistant to amoxicillin/clavulanate by the automated system and susceptible by both the reference method and RMM. Although one isolate was considered as susceptible by the automated system, it was determined as resistant by both the reference method and RMM. While one isolate was found to be resistant by both the automated system and the reference method, it was susceptible by RMM. Table 2 shows the evaluation of antibiotics according to the FDA criteria.
Evaluation of Antibiotic Results According to FDA Criteria
Antibiotics | EA (%) | CA (%) | VME (%) | ME (%) | mE (%) |
---|---|---|---|---|---|
Ciprofloxacin | 97.27 | 98.18 | 1.36 | 2.38 | 0 |
Gentamycin | 94.54 | 97.27 | 5.7 | 1.35 | 0 |
Ertapenem | 93.63 | 99.09 | 5.8 | 0 | 0 |
Ceftriaxone | 98.18 | 100 | 0 | 0 | 0 |
Trimethoprim/ sulfamethoxazole | 100 | 100 | 0 | 0 | 0 |
Nitrofurantoin | 91.81 | 95.45 | 45.45 | 0 | 0 |
Cefixime | 94.54 | 99.09 | 2.17 | 0 | 0 |
Amoxicillin/clavulanate | 82.72 | 96.36 | 6.77 | 0 | 0 |
The EA and CA values were 97.27 and 98.18% for ciprofloxacin, 94.54 and 97.27% for gentamicin, 93.63 and 99.09% for ertapenem, and 98.18 and 100% for ceftriaxone. The EA and CA values were 100 and 100% for trimethoprim/sulfamethoxazole; however, they were 91.81 and 95.45% for nitrofurantoin, 94.54 and 99.09% for cefixime, and 82.72 and 96.36% for amoxicillin/clavulanate. For the eight antibiotics tested in the study, the table shows the EA values of 82.72 - 100%, the CA values of 95.45 - 100%, the VME values of 0 - 45.45%, the ME of values of 0 - 2.38%, and the mE value of 0%.
5. Discussion
Escherichia coli is one of the most common causes of hospital and community-acquired bacterial infections in humans, including urinary tract infections, enteric infections, and systemic infections. Antimicrobial resistance is a global problem, for which some measures need to be adopted at local, national, and global scales. Antibiotic management and effective infection control procedures would contribute to preserving the efficacy of older antibiotics (7). It is often challenging to determine the infectious agent and the most appropriate antibiotic to be used and initiate treatment on the same day. Determining antimicrobial susceptibility by traditional antimicrobial susceptibility test (AST) methods may take several days; therefore, clinicians use broad-spectrum antibiotics to initiate treatment and prevent the further spread of the disease. This approach may arouse antibiotic resistance. The increasing frequency of antibiotic resistance is a globally emerging problem, and the lack of new antibiotics requires the improvement of current diagnostic tools and rapid antibiotic susceptibility testing (8).
Antibiotic resistance continues to emerge and pose difficulties in treatment, especially for Gram-negative bacteria. Determining antibiotic resistance using growth-independent methods is expected to shorten the time required to start treatment (9, 10). The AST results are documented to be effective for the timely application of effective antimicrobial treatment; hence, clinicians should have access to them as quickly as possible. To be more specific, the increase in multidrug-resistant microorganisms highlights the need for rapid AST and the rapid detection of certain resistance mechanisms. In this regard, it has been emphasized that immunochromatographic analyses can be performed by DNA amplification methods and colorimetric or MALDI-TOF MS-based tests. However, the main disadvantage of these tests is that a negative result in resistance detection does not necessarily mean susceptibility. Some alternative resistance mechanisms may also cause microbial resistance, thereby resulting in treatment failure. While rapid phenotypic AST allows the independent and precise categorization of resistance mechanisms, time is required for microorganisms to begin to reproduce, and economic and easy-to-use fast AST technologies may have the opportunity to enter a broad diagnostic routine in the future (11).
In a study, polymyxin B activity against 70 carbapenems resistant Enterobacteriaceae (CRE) clinical isolates was determined using Etest® and BMD. In this study, 49 and 36% of the CRE isolates were Klebsiella spp. and Enterobacter spp., respectively. Polymyxin B susceptibility was significantly higher in Etest® when compared to BMD (97 versus 77%; p 0.0001). The isolates are predominantly Klebsiella spp. (34/70; 49%), followed by Enterobacter spp. (25/70; 36%), E. coli (5/70; 7%), Citrobacter spp. (5/70; 7%). Furthermore, and Providencia retrained (1/70; 1%). Moreover, CA was 80%; however, EA was as low as 10%. While false susceptibility was never observed in Etest® (according to the reference BMD), VME was high (88%). It was stated that reporting false susceptibility by Etest® may cause clinically inappropriate antibiotic use and treatment failure, as such it is not recommended to use Etest® as a susceptibility test for polymyxin B routinely (12).
The studies using resazurin as an indicator in the literature have considered enterococci, yeasts, Pseudomonas aeruginosa, Mycobacterium tuberculosis, and S. aureus. However, there are no studies on the E. coli isolates. In a study using RMM, vancomycin susceptibilities in the clinical isolates of enterococci were compared using BMD. Moreover, CA and EA were 100%, and mE, ME, and VME were 0% (13). Nitrate reductase analysis (NRA) and resazurin microplate analysis (REMA) colorimetric methods are developed to detect vancomycin and oxacillin-resistant S. aureus in < 6 hours. The agreement and discrepancy between BMD, REMA, and NRA were compared for S. aureus. The oxacillin and vancomycin MIC results obtained by BMD were compared with those reported by REMA and NRA. In this regard, EA and AA for oxacillin were 86.5and 100% in REMA and 91.6 and 100% in NRA. For vancomycin, EA and AA were 81.2% in REMA and 98.9% in NRA, and 63.5 and 98.9% in NRA. While the mE values were 1.04% in REMA and NRA, no ME or VME was noticed. The oxacillin and vancomycin susceptibility results obtained by REMA and NRA were in concordance with the standard method (14).
The rapid colorimetric efficiency of the NRA and REMA methods were compared to determine the methicillin resistance in S. aureus quickly. The cefoxitin MICs of all isolates included in the study were determined using NRA, REMA, and reference BMD. Compared to the reference method, CA and EA were 100% in NRA and 99.6% in REMA. No ME, mE, or VME was detected in the concerned method. The MIC results were obtained during 5 hours in the NRA method and during 6 hours in the REMA method (15). Polymyxin resistance in Acinetobacter baumannii and P. aeruginosa isolates was determined by a method using a resazurin-based stain (PrestoBlue). The results were compared using BMM. The method’s sensitivity was 100%, and the specificity was 95% (16).
Resazurin microplate analysis evaluated performance in tuberculosis drugs, sensitivity; While the specificity is between 94 - 98% for isoniazid, 95 - 98% for rifampicin, 87 - 96% for ethambutol, 88 - 95% for streptomycin; 95 - 98% for isoniazid, 98 - 99% for rifampicin, 81 - 89% for ethambutol, 87 - 93% for streptomycin (17). A new colorimetric test kit, StaResMet®, was compared with the VITEK2 Compact automated system and reference BMD to detect methicillin resistance in S. aureus isolates. The positive and negative predictive values, specificity, and sensitivity of the new colorimetric StaResMet® kit were 100% (18). In the new resazurin fast AST, where the thin platinum film is used as the bio-electrode, ampicillin, kanamycin, and tetracycline susceptibilities were tested using Klebsiella pneumoniae ATCC700603, E. coli ATCC25922 strain, and the results were compared with those of the disk diffusion method. The new electrochemical method and the disk diffusion method results were compatible (8).
The rapid ResaPolymixin test performance developed for detecting colistin resistance (ColR) in A. baumannii was also evaluated. Among the rapid ResaPolimiksin NP test results and the reference liquid microdilution method, there were sensitivity and specificity of 93.3%, CA of 95.1%, VME of 1.2%, and ME of 3.7%. Positive and negative predictive values were also 92.3 and 97.7%, respectively (19). A device including pathogen identification was developed to determine the antibiotic treatment to be used in urinary tract infections (UTIs). It was revealed that the method detecting 13 types of uropathogens could identify types of UTI pathogens in artificial urine samples during 20 minutes and determine the results of AST for eight antibiotics during 3 - 6 hours. The researchers predicted that this device could be widely used in treating UTIs and be a model for diagnosing and treating other diseases (20).
A general method was developed to identify bacterial species causing infection and determine their antibiotic susceptibility. They determined the antimicrobial susceptibilities of E. coli for ciprofloxacin and trimethoprim in UTIs with 100% accuracy during 3.5 hours. They compared the results with those of the disk diffusion test and found the specificity and sensitivity of their test to be 100% (21). It was pointed out that the use of resistance detection techniques such as PCR or MALDI-TOF MS only for gene detection is essential, and the performance of these tests against new or undefined resistance mechanisms should also be determined (22). StaResMet® kit was evaluated for the rapid detection of methicillin resistance in S. aureus isolates. Methicillin-resistant S. aureus (MRSA) and methicillin-sensitive S. aureus (MSSA) isolates were tested, and the MRSA and MSSA isolates were identified with 100% accuracy. The StaResMet® kit detects MRSA isolates quickly and reliably and could be a valuable option for microbiology laboratories with limited resources (23).
The reference BMD method should be used as a gold standard to compare commercial antimicrobial susceptibility test results. Moreover, although the agar dilution and disk diffusion method are among the reference CLSI methods, they were developed against BMM. In the present study, a liquid microdilution-based rapid colorimetric method was developed (24). This study has some limitations. The number of clinical isolates and antibiotics could have been increased. Moreover, multi-centered studies should be performed to test RMM for E. coli isolates.
5.1. Conclusions
The provision of the AST results in the shorted time is of paramount importance for critical patient groups, for whom antibiotic treatment should be initiated early. Although AST in automated systems provides results quickly, they are high-cost tests preferred by large laboratories. The present study aimed to address such shortcomings as such the proposed method allowed the detection of antibiotic susceptibilities quickly (e.g., during 5 hours). Since the cost of the proposed RMM is relatively low compared to automated systems, hospitals and laboratories with limited facilities can afford it.
References
-
1.
Findik D. Infectious diseases and microbiology. 4th ed. Istanbul, Turkey: Nobel Medical Bookstores; 2017.
-
2.
Tuem KB, Gebre AK, Atey TM, Bitew H, Yimer EM, Berhe DF. Drug resistance patterns of Escherichia coli in Ethiopia: A meta-analysis. Biomed Res Int. 2018;2018:4536905. [PubMed ID: 29854757]. [PubMed Central ID: PMC5960519]. https://doi.org/10.1155/2018/4536905.
-
3.
Reed TAN, Krang S, Miliya T, Townell N, Letchford J, Bun S, et al. Antimicrobial resistance in Cambodia: A review. Int J Infect Dis. 2019;85:98-107. [PubMed ID: 31176035]. https://doi.org/10.1016/j.ijid.2019.05.036.
-
4.
Clinical and Laboratory Standards Institute. Performance Standards for Antimicrobial Susceptibility Testing. Wayne, USA: Clinical and Laboratory Standards Institute; 2017.
-
5.
The European Committee on Antimicrobial Susceptibility Testing. Routine and extended internal quality control for MIC determination and disk diffusion as recommended by EUCAST. Basel, Switzerland: European Society of Clinical Microbiology and Infectious Disease; 2020.
-
6.
U.S. Food and Drug Administration. Class II Special Controls Guidance Document: Antimicrobial Susceptibility Test (AST) Systems. Maryland, USA: Center for Devices and Radiological Health; 2009.
-
7.
Pitout JD. Extraintestinal pathogenic Escherichia coli: An update on antimicrobial resistance, laboratory diagnosis and treatment. Expert Rev Anti Infect Ther. 2012;10(10):1165-76. [PubMed ID: 23199402]. https://doi.org/10.1586/eri.12.110.
-
8.
Mishra P, Singh D, Mishra KP, Kaur G, Dhull N, Tomar M, et al. Rapid antibiotic susceptibility testing by resazurin using thin film platinum as a bio-electrode. J Microbiol Methods. 2019;162:69-76. [PubMed ID: 31103460]. https://doi.org/10.1016/j.mimet.2019.05.009.
-
9.
Durupınar B. New trends in resistance to antibiotics. Klimik Journal. 2001;14(2):47-56.
-
10.
Charretier Y, Schrenzel J. Mass spectrometry methods for predicting antibiotic resistance. Proteomics Clin Appl. 2016;10(9-10):964-81. [PubMed ID: 27312049]. https://doi.org/10.1002/prca.201600041.
-
11.
Idelevich EA, Becker K. How to accelerate antimicrobial susceptibility testing. Clin Microbiol Infect. 2019;25(11):1347-55. [PubMed ID: 31055166]. https://doi.org/10.1016/j.cmi.2019.04.025.
-
12.
Kulengowski B, Ribes JA, Burgess DS. Polymyxin B Etest(R) compared with gold-standard broth microdilution in carbapenem-resistant Enterobacteriaceae exhibiting a wide range of polymyxin B MICs. Clin Microbiol Infect. 2019;25(1):92-5. [PubMed ID: 29674129]. https://doi.org/10.1016/j.cmi.2018.04.008.
-
13.
Coban AY, Darka O, Tasdelen Fisgin N, Cihan CC, Bilgin K, Akgunes A, et al. The resazurin microplate method for rapid detection of vancomycin resistance in enterococci. J Chemother. 2005;17(4):361-6. [PubMed ID: 16167513]. https://doi.org/10.1179/joc.2005.17.4.361.
-
14.
Coban AY, Bozdogan B, Cihan CC, Cetinkaya E, Bilgin K, Darka O, et al. Two new colorimetric methods for early detection of vancomycin and oxacillin resistance in Staphylococcus aureus. J Clin Microbiol. 2006;44(2):580-2. [PubMed ID: 16455915]. [PubMed Central ID: PMC1392707]. https://doi.org/10.1128/JCM.44.2.580-582.2006.
-
15.
Coban AY. Rapid determination of methicillin resistance among Staphylococcus aureus clinical isolates by colorimetric methods. J Clin Microbiol. 2012;50(7):2191-3. [PubMed ID: 22518854]. [PubMed Central ID: PMC3405631]. https://doi.org/10.1128/JCM.00471-12.
-
16.
Lescat M, Poirel L, Tinguely C, Nordmann P. A Resazurin Reduction-Based Assay for Rapid Detection of Polymyxin Resistance in Acinetobacter baumannii and Pseudomonas aeruginosa. J Clin Microbiol. 2019;57(3). [PubMed ID: 30567745]. [PubMed Central ID: PMC6425163]. https://doi.org/10.1128/JCM.01563-18.
-
17.
Coban AY, Deveci A, Sunter AT, Palomino JC, Martin A. Resazurin microtiter assay for isoniazid, rifampicin, ethambutol and streptomycin resistance detection in Mycobacterium tuberculosis: Updated meta-analysis. Int J Mycobacteriol. 2014;3(4):230-41. [PubMed ID: 26786621]. https://doi.org/10.1016/j.ijmyco.2014.09.002.
-
18.
Soysal M, Yilmaz Coban A. Evaluation of StaResMet® for Rapid Detection of Methicillin Resistance in Clinical Isolates of Staphylococcus aureus. Klimik Dergisi/Klimik Journal. 2017;30(2):64-7. https://doi.org/10.5152/kd.2017.16.
-
19.
Germ J, Poirel L, Kisek TC, Spik VC, Seme K, Premru MM, et al. Evaluation of resazurin-based rapid test to detect colistin resistance in Acinetobacter baumannii isolates. Eur J Clin Microbiol Infect Dis. 2019;38(11):2159-62. [PubMed ID: 31372908]. https://doi.org/10.1007/s10096-019-03657-1.
-
20.
Dong T, Zhao X. Rapid identification and susceptibility testing of uropathogenic microbes via immunosorbent ATP-bioluminescence assay on a microfluidic simulator for antibiotic therapy. Anal Chem. 2015;87(4):2410-8. [PubMed ID: 25584656]. https://doi.org/10.1021/ac504428t.
-
21.
Mezger A, Gullberg E, Goransson J, Zorzet A, Herthnek D, Tano E, et al. A general method for rapid determination of antibiotic susceptibility and species in bacterial infections. J Clin Microbiol. 2015;53(2):425-32. [PubMed ID: 25411178]. [PubMed Central ID: PMC4298551]. https://doi.org/10.1128/JCM.02434-14.
-
22.
Pulido MR, Garcia-Quintanilla M, Martin-Pena R, Cisneros JM, McConnell MJ. Progress on the development of rapid methods for antimicrobial susceptibility testing. J Antimicrob Chemother. 2013;68(12):2710-7. [PubMed ID: 23818283]. https://doi.org/10.1093/jac/dkt253.
-
23.
Milletli Sezgin F, Vural A, Kiraz A, Tanriverdi Cayci Y, Coban C, Bozdogan B, et al. Same-day detection of methicillin resistance in Staphylococcus aureus isolates by StaResMet® Kit. Jundishapur J Microbiol. 2017;10(11). https://doi.org/10.5812/jjm.14937.
-
24.
Humphries RM, Ambler J, Mitchell SL, Castanheira M, Dingle T, Hindler JA, et al. CLSI methods development and standardization working group best practices for evaluation of antimicrobial susceptibility tests. J Clin Microbiol. 2018;56(4). [PubMed ID: 29367292]. [PubMed Central ID: PMC5869819]. https://doi.org/10.1128/JCM.01934-17.