Abstract
Background:
Wound healing is a complex and overlapping process involving immune cells, cytokines, and growth factors.Objectives:
This study aimed to design and evaluate a novel wound dressing based on postbiotic/chitosan in accelerating wound healing.Methods:
Lactobacillus reuteri PTCC1655 was cultured, and the cell-free supernatant (postbiotic) was obtained by medium centrifugation. The films were prepared using the solvent casting method and evaluated in terms of water absorption index, water vapor transmission rate, and antimicrobial properties. Forty-five male Wistar rats were subjected to a full-thickness excisional wound to assess the wound healing potential. The rats were randomly divided into control, chitosan, and postbiotic groups. The time-course histological and gene expression analysis was performed to compare the dressing efficacy.Results:
The films showed proper water absorption and water vapor transmission rate and inhibited the pathogens commonly associated with wound infection. The postbiotic film improved wound healing by modulating the inflammatory phase, increasing collagen and elastin deposition, and enhancing angiogenesis based on the histological results. The gene expression assay showed that the postbiotic film accelerated wound healing by improving the expression of inflammatory mediators (IL-6 and TNF-α) and anti-inflammatory mediators (TGF-β and VEGF).Conclusions:
The cell-free supernatant/chitosan/polyethylene glycol (CFS/CS/PEG) biodegradable film could be introduced as a novel dressing for cutaneous wound healing. This transparent film enhances cutaneous wound healing by modulating infiltrated immunity cells and expressing inflammatory/anti-inflammatory cytokines.Keywords
1. Background
Skin is the first line of defense that creates a protective barrier for the body through dense layers (1). Surgical cuts, burns, scalds, and regular physical activities generate skin wounds that are susceptible to bacterial infections, resulting in exudate production, excessive inflammation, festering, and hindered wound healing for millions of people worldwide (2). The most common aerobic isolates recovered from this type of illness are Corynebacterium spp., Enterococcus spp., Escherichia coli, Staphylococcus epidermidis, and S. aureus. The most usually isolated anaerobic bacteria are Fusobacterium spp., Porphyromonas spp., Prevotella spp., Bacteroides spp., and Clostridium spp., which are frequently found in polymicrobial communities with a wide range of species (3).
The wound healing process involves the complex and overlapping homeostasis, inflammation, proliferation, and remodeling stages. The complex interplay between immune cells, interleukins, and growth factors promotes wound healing (4, 5). There are many Wound Healing-associated Genes (WHAGs), including Fibroblast Growth Factor 2 (FGF2), Platelet-derived growth factor, B polypeptide (PDGFB), Transforming Growth Factor, Beta 3 (TGFB3), Vascular Endothelial Growth Factor A (VEGFA), Epidermal Growth Factor (EGF), Matrix Metallopeptidase 8 (MMP8), and Matrix Metallopeptidase 3 (MMP3) (6).
Unfortunately, there is no effective universal treatment method for wound healing so, there is an increasing demand for modern treatment strategies. Natural polymers in films, sponges, hydrogels, and hydrocolloid forms are used to design novel wound dressing such as chitosan, alginate, cellulose, pectin, and gelatin (7, 8). Chitosan is a biopolymer containing glucosamine, N-acetylglucosamine, and distilled alkaline products of chitin. This compound has non-toxic, biological, and biodegradable properties, with low cost (9, 10). Considerable research has been undertaken to improve the properties of chitosan for medicinal uses. Chitosan is frequently used as a wound dressing and antibacterial agent (11). For instance, Du et al. created a novel chitosan-based hydrogel by cross-linking hydrocaffeic acid-modified chitosan with hydrophobically modified chitosan, which reduced bacterial wound infection and showed good cell/tissue compatibility (12).
The Food and Medication Administration (FDA, USA) has approved polyethylene glycol (PEG) for drug delivery systems (13). Polyethylene glycol is used due to its biocompatibility, biodegradability, and low toxicity (14). Significant improvement was observed in the mechanical properties of chitosan after combination with PEG. Zhang et al. reported that chitosan/PEG films improved protein adsorption and cell growth (15). Chitosan/PEG has been developed for controlled drug release in films and microsphere forms (16). Wang et al. (17) study revealed that the amount of ciprofloxacin hydrochloride released from chitosan/PEG films was higher than that from PEG films. In addition, the chitosan/PEG membrane was used as an oral drug delivery system, leading to successful drug delivery into the intestine (18). It was also reported that the elasticity of the chitosan/PEG composite membrane was better than that of the chitosan/PEG membrane (19).
There is strong evidence that skin microbiota is intimately coupled with wound healing. These bacteria produce bioactive compounds to regulate immune responses, alter cell infiltration, and express chemokines in wound sites. Historically, fermented foods are used for the improvement of wound healing. Lactic acid bacteria play an essential role in the production of fermented foods. They produce different metabolites that modulate the immune system and show great antimicrobial activity. Lactobacillus reuteri is a probiotic used in the production of cosmetic probiotic products. This bacterium stimulates keratinocytes and alters the expression pattern of cytokines and growth factors involved in the wound healing process (20).
The supernatant of L. reuteri has been shown to stimulate the production of prostaglandin E2 (PGE2) and lead to wound healing (20). There has recently been a surge of interest in postbiotics, the newest members of the biotics family. Postbiotics are functional bioactive chemicals created during fermentation by the metabolic activity of probiotics that have direct or indirect positive effects on the host cells (21). Although postbiotics do not include living microorganisms, they positively impact host health through mechanisms similar to those seen in probiotics, reducing the hazards associated with their consumption. As a result, postbiotics appear to be safe due to the lack of any potential adverse effects for live microorganisms while maintaining similar efficacy to probiotics (22, 23).
2. Objectives
This study aimed to produce a novel wound dressing based on the postbiotic of L. reuteri and chitosan. We loaded the postbiotic of this bacterium in chitosan films to produce a novel dressing. Postbiotic is defined as a “preparation of inanimate microorganisms and/or their components that confers a health benefit on the host.” Chitosan is a natural cationic polymer derived from chitin with an annual production of over 100 billion tons (24, 25). This natural polymer is intensively engaged in wound healing because it has extensive antimicrobial activity and biocompatibility. We used polyethylene glycol (PEG) to enhance the mechanical properties of chitosan films (13, 24).
3. Methods
3.1. Preparation of Cell-free Supernatant of Lactobacillus reuteri
Lactobacillus reuteri PTCC1655 was purchased from the Iranian Research Organization for Science and Technology (IROST). The bacterium was cultured in De Man Rogosa and Sharpe (MRS) broth medium and incubated in a shaker incubator at 50 rpm for 48 h at 35ºC. The supernatant was obtained by centrifugation at 8,000 rpm for 20 min at 4ºC. The supernatant was passed through a 0.2 μm filter and stored at -20ºC (26).
3.2. Evaluation of Postbiotic Properties
The protein concentration of the postbiotic was determined by colorimetric QuickStart™ Bradford Protein Assay Kit (DNA Biotech, Iran) using bovine serum albumin as standard (27). The concentrations of organic acids (lactic acid, acetic acid, citric acid, oxalic acid, and succinic acid) and hydrogen peroxide were determined according to Coda et al., using reversed-phase high-performance liquid chromatography (RP-HPLC) (28). The system (HPLC Shimadzu, Nakagyo-Ku, Kyoto, Japan) consisted of a YMC-Triart C18 column (250 × 4.6 mm ID, S-5 um, 12 nm) with the column temperature of 35ºC. The postbiotic (50 µL) was injected into HPLC equipped with a UV absorption detector (Shimadzu) at the 210 nm wavelength. Besides, KH2PO4 (0.009 M) and phosphoric acid with pH 2.06 were used as a mobile phase with the flow rate of 1 mL/min. The organic acids and hydrogen peroxide (Sigma) solutions were prepared in ultrapure water and used as the standard (28).
3.3. Production of Postbiotic Dressing Film
To prepare the CFS/CS/PEG film, the filtrated cell-free supernatant (postbiotic) was diluted in sterile deionized water (40:60), and the pH was adjusted to 3.5 using acetic acid. Then, 1 g of chitosan (Merck, Germany) was added to 50 mL distilled water and stirred overnight to obtain a clear solution. Then, 50 mL of postbiotic and 0.5 mL of glacial acetic acid were added to chitosan, and the solution was stirred at 30°C for 30 min. In the next step, 4 mL of 10% PEG was added to the prepared chitosan-postbiotic-glacial acetic acid mixture and stirred for 2 h at 30ºC. The CFS/CS/PEG homogeneous mixture solutions were prepared by ultrasonic blending for 30 s, followed by vacuum filtration with a sand core funnel. Then, the composite was cast as a thin layer inside preheated sterile glass plates. The plates were incubated at 37°C overnight. The films were neutralized by merging in NaOH (0.1 M) for 10 s and sterilized by 70% ethanol and UV. Finally, the films were dried by placing them in a 40ºC oven for 30 min. We produced a chitosan film (without postbiotic) as the control. All the mentioned steps were performed without postbiotic to prepare the control film.
3.4. Physical Properties of Films
A piece of 2 × 2 cm2 of films was carefully weighed and immersed in normal saline (0.9 wt% NaCl, pH 7.2) at room temperature to determine the swelling index (SI) (29). Blotting surface water was taken at regular intervals, and the films were re-weighed. The SI index was determined using the following formula (29):
Wwet = the weight of the wet film after water absorption; Wdry = the initial weight of the film.
The films' water vapor transmission rate (WVTR) was assessed by the modified wet cup method according to the JIS 1099A standard. Briefly, 50 g of calcium chloride was placed in a beaker (7 cm diameter). The tested films were used to cover the container and incubated at 40 ± 2°C for 1 h in 90 ± 5% relative humidity (29). The WVTR was calculated using the following formula:
W1 = the initial weight; W2 = the final weight after incubation; S = the area of the beaker input covered by the film
3.5. Antimicrobial Properties Films
The antimicrobial properties of films against Pseudomonas aeruginosa PTCC1950, Staphylococcus aureus PTCC1826, and Escherichia coli PTCC1789 (IROST, Iran) were assessed according to Ko et al. (30). The bacteria were cultured in a brain heart infusion (BHI) broth (Difco, USA) medium. After 18 h incubation, the cells were separated by centrifugation at 8,000 rpm for 20 min at 4°C. The cells were re-suspended in Phosphate-buffered Saline (PBS) with pH 7.2 until they reached the predetermined turbidity value (equivalent to 2×105 CFUs/mL). Then, 50 µL of each bacterial suspension was transferred to the films (8 × 8 mm2) and incubated at room temperature for 0, 30, 60, 90, and 120 min. The films were transferred to the stomacher bag, and PBS solution was added to a final volume of 1 mL. After 2 min stomaching, the number of live bacteria was counted by spread culture in BHI agar medium. As a control, indicator cell suspensions were transferred to a filter membrane, and sampling was performed at the same time intervals.
3.6. Wound Healing Properties
3.6.1. Maintenance and Wound Creation in Rats
Forty-five male Wistar rats with 200 - 250 g weight were obtained from the Pasteur Institute of Iran. Under standard conditions, the animals were kept in separate cages, 23 ± 2°C, 50% relative humidity, and a 12 h dark/light cycle. All procedures performed in studies followed the guidelines for the care and use of laboratory animals prepared by the institute of laboratory animal resources (31). After a 10-day acclimatization period, the animals were randomly divided into negative control (with no treatment), chitosan, and postbiotic groups (n = 15). The animals were anesthetized by the intramuscular injection of ketamine (80 mg/kg) and xylazine (10 mg/kg). Their hair was shaved, and the skin was disinfected using povidone-iodine and ethanol (32, 33). A full-thickness excisional wound (1.3 × 1.3 cm2) was created on the dorsal surface of the animals. The films were moistened with sterile distilled water and placed on the wound. It should be noted that the wound was treated only once with the films during the experiment period.
3.6.2. Percentage of Wound Healing
Three rats of each group were killed on days 1, 3, 7, 14, and 21 post-wounding. The wound area was measured using image analyzing software (Image J, NIH, USA), and the percentage of wound healing was calculated by the following formula (34):
Where A0 and An are the wound areas on days 0 and n, respectively.
3.6.3. Histological Examination of Tissues
The wounds and the surrounding healthy tissue were wholly removed and divided into two parts for histological and gene expression analysis. The tissue samples were fixed in paraformaldehyde (4% in PBS, 0.01 M, pH 7.4) (35). Paraffin blocks were prepared based on the Canene-Adams protocol, and the slides with 5 mm thick incisions were prepared and stained with hematoxylin and eosin (H&E) (36). For evaluating the collagen and elastin deposition, the slides were stained by Masson’s Trichrome stains and Orcein stain kit, respectively (37). The comparative scoring of collagen and elastin deposition was performed as follows: - Absence, + discrete, ++ moderate, +++ intense, and ++++ very intense.
3.6.4. Gene Expression During Wound Healing
The wound tissues were snap-freezed in liquid nitrogen to perform gene expression experiments (35). According to the manufacturer's instructions, total RNAs were extracted from the frozen tissue samples using the Universal Purification kit (EURX, Poland). PrimeScript RT reagent kit (Takara Bio, Ohtsu, Japan) was used to synthesize cDNA from a 10 μg RNA sample. Quantitative real-time PCR was performed using SYBR Premix Ex Taq (Takara Bio, USA) (37). The sequences and characteristics of the primers of target genes and β-actin (as internal standard) are given in Table 1. After determining the ΔCT value (ΔCT = CT β-actin – Ct target mRNA), the normalized expression values relative to the calibrator were calculated using formula R = 2–ΔCt.
Primers Sequence and Specification for Evaluation of Gene Expression During Wound Healing
Gene | Tm (°C) | Primes Sequence | Amp. Size (bp) |
---|---|---|---|
TGF-β | 84.9 | F: 5′- CCTGGATACCAACTATTGCTTCAG -3′ | 133 |
R: 5′- CAGACAGAAGTTGGCATGGTAG -3′ | |||
TNF-α | 83.63 | F: 5′- CCAGGAGAAAGTCAGCCTCCT -3′ | 87 |
R: 5′- TCATACCAGGGCTTGAGCTCA -3′ | |||
IL-6 | 84.32 | F: 5′- CGAAAGTCAACTCCATCTGCC-3′ | 74 |
R: 5′- GGCAACTGGCTGGAAGTCTCT-3′ | |||
VEGF | 81.6 | F: 5′- ATCATGCGGATCAAACCTCACC - 3′ | 80 |
R: 5′- GGTCTGCATTCACATCTGCTATGC- 3′ | |||
β -actin | 86.5 | F: 5′- AGAGCTATGAGCTGCCTGACG -3′ | 230 |
R: 5′- CTGCATCCGGTCAGCGATAC -3′ |
3.7. Statistical Analysis
The statistical analysis of quantitative variables was performed using Graphpad Prism 8 software with one-way ANOVA and t-test at a significance level of P-value = 0.05.
4. Results
4.1. Characterization of Postbiotic
The results showed that organic acids comprised 47% of the supernatant (Figure 1). Out of the five different organic acids, lactic acid was produced in the highest concentration (21.86%). The acetic acid, citric acid, and oxalic acid concentrations were 19.18, 3.41, and 3.091%, respectively. Succinic acid was not detected. The H2O2 concentration was 8.175%. The total protein concentration of the supernatant was 39.39%.
The concentration of organic acids in cell-free supernatant of Lactobacillus reuteri. LA: lactic acid (21.86%), AA: acetic acid (19.18%), CA: citric acid (3.41%), OA: oxalic acid (3.091%), and SA: succinic acid (not detected).
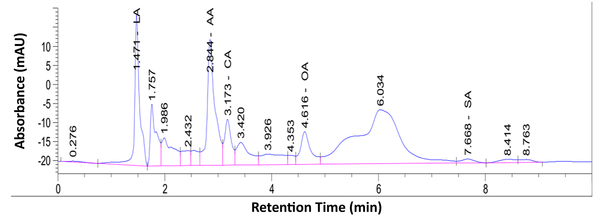
4.2. Chemical Properties of Dressings
Our results showed that the maximum SI was achieved within 180 min for both films (1,115% and 1,088% for chitosan and postbiotic films, respectively) (Figure 2). The postbiotic in the film did not affect the water absorbance property (P = 0.844). The films gradually absorbed water, and there was no rapid disintegration in their structures. From the 180th min onwards, the films did not absorb water. The WVTR was 2.290 kg/m2/day for the chitosan film and 2.186 kg/m2/day for the postbiotic film. Thus, the postbiotic did not alter the ability to transmit vapor (P = 0.630).
A, Electron micrographs of chitosan film; B, Electron micrographs of postbiotic film; and C, Swelling index of composite films of chitosan and postbiotic films in 300 min.
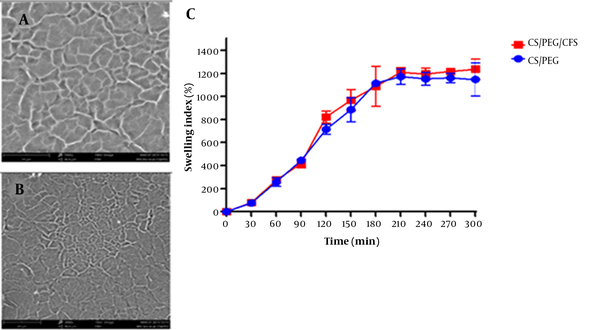
4.3. Antimicrobial Properties of Films
The postbiotic film had higher antimicrobial properties against E. coli and S. aureus (P = 0.0012 and P = 0.0411, respectively) (Figure 3). The chitosan film did not inhibit the growth of P. aeruginosa, while the postbiotic film significantly reduced its growth (P = 0.002).
The bacterial growth expressed as the logarithm of the number of colonies grown in the presence of CS/PEG and CFS/CS/PEG films.
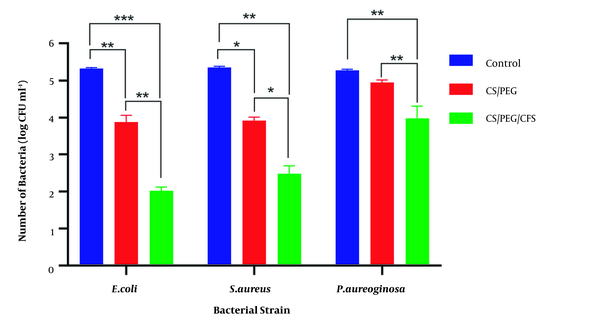
4.4. Improvement of Wound Healing Process
The healing process was assayed on days 1, 3, 7, 14, and 21. The results showed the complete closure of the postbiotic-covered wound within 14 days. The wound was completely closed in the control and chitosan groups after 21 and 16 days, respectively (Table 2 and Figure 4). Neutrophils, macrophages, and fibroblasts were evaluated during wound healing (Table 3 and Figure 5). Our results showed that the maximum number of neutrophils in both treated groups reached faster on day 1 and reduced in the following days. The number of macrophages in the treated groups reached the maximum on day 3, while it was significantly higher in the postbiotic group than in the chitosan group (P = 0.102). Fibroblasts did not differ significantly among the three groups on the first day (P = 0.182). Their number in the treated groups reached the peak on day 7, which was significantly higher than in the control group (P < 0.0001). On day 7, collagen and elastin deposition started in the treated groups and increased gradually (Table 2 and Figure 4). The accumulation of these fibers was higher in the postbiotic group than in the other group.
Wound Healing, Collagen, and Elastin Deposition in Control, Chitosan, and Postbiotic Groups a
Groups, Day | Control | Chitosan | Postbiotic |
---|---|---|---|
Wound healing, mean ± SEM % | |||
1 | 8.75 ± 0.41 c | 23.38 ± 1.69 b | 32.25 ± 1.31 a |
3 | 30.75 ± 1.37 c | 49.38 ± 0.75 b | 59.63 ± 1.51 a |
7 | 56.67 ± 0.84 c | 75.17 ± 0.70 b | 87.50 ± 0.88 a |
12 | 72.25 ± 1.31 c | 91.75 ± 0.48 b | 99.13 ± 0.12 a |
14 | 78.50 ± 1.50 b | 95.50 ± 0.87 b | 100 a |
16 | 86.16 ± 0.94 b | 99.63 ± 0.10 a | 100 a |
21 | 94.36 ± 1.05 a | 100 a | 100 a |
Collagen deposition | |||
1 | - | - | - |
3 | - | - | - |
7 | ++ | + | - |
12 | ++ | + | - |
14 | +++ | ++ | + |
16 | +++ | ++ | + |
21 | ++++ | +++ | ++ |
Elastin deposition | |||
1 | - | - | - |
3 | + | + | - |
7 | ++ | + | - |
12 | ++ | + | - |
14 | ++++ | ++ | + |
16 | ++++ | ++ | + |
21 | ++++ | +++ | +++ |
Wound images
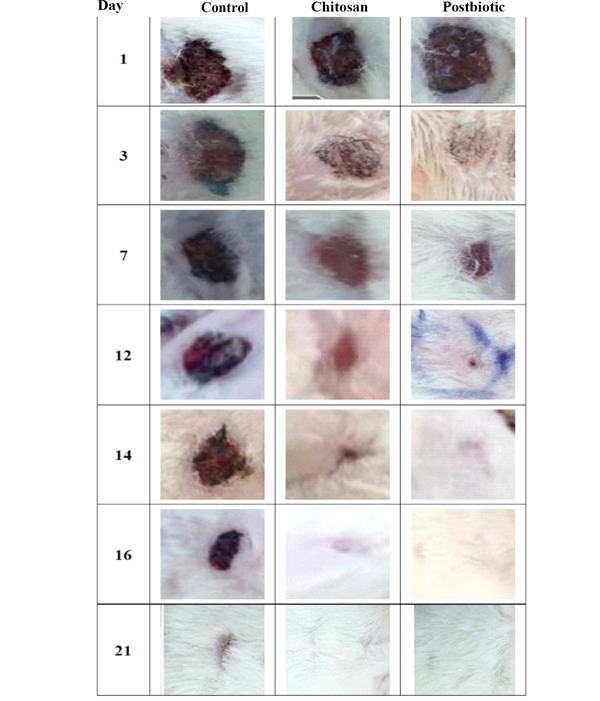
Mean ± SEM of Neutrophils, Macrophages, and Fibroblasts in Wound Healing Process a
Day | Neutrophils | Macrophages | Fibroblasts | ||||||
---|---|---|---|---|---|---|---|---|---|
Control | Chitosan | Postbiotic | Control | Chitosan | Postbiotic | Control | Chitosan | Postbiotic | |
1 | 10.62 ± 0.54 b | 15.54 ± 0.52 a | 14.94 ± 0.53 a | 2.84 ± 0.25 a | 3.45 ± 0.46 a | 3.35 ± 0.28 a | 2.13 ± 0.32 a | 2.83 ± 0.31 a | 3.02 ± 0.36 a |
3 | 16.71 ± 1.11 a | 9.14 ± 0.51 b | 6.29 ± 0.68 b | 3.29 ± 0.52 c | 10.14 ± 0.63 b | 12.71 ± 0.47 a | 2.14 ± 0.40 b | 4.14 ± 046 a | 4.86 ± 0.67 a |
7 | 7.86 ± 0.86 a | 3.14 ± 0.59 b | 2.86 ± 0.51 b | 18.14 ± 0.60 a | 4.86 ± 0.40 b | 4.29 ± 0.42 b | 3.14 ± 0.46 b | 17.29 ± 0.61 a | 18.71 ± 0.52 a |
14 | 5.57 ± 0.78 a | 2.14 ± 0.34 b | 1.86 ± 0.40 b | 10.29 ± 0.68 a | 3.30 ± 0.44 b | 2.57 ± 0.37 b | 10.14 ± 0.60 a | 7.86 ± 0.51 b | 6.14 ± 0.74 b |
21 | 3.43 ± 0.43 a | 2.00 ± 0.31 b | 1.857 ± 0.26 b | 4.57 ± 0.37 a | 2.14 ± 0.34 b | 1.71 ± 0.29 b | 7.14 ± 0.26 a | 5.29 ± 0.42 b | 4.14 ± 0.63 b |
Histological evaluation of wounds treated with CS/PEG and CSF/CS/PEG and untreated (NC) on days 1, 3, 7, 14, and 21 post-wounding.
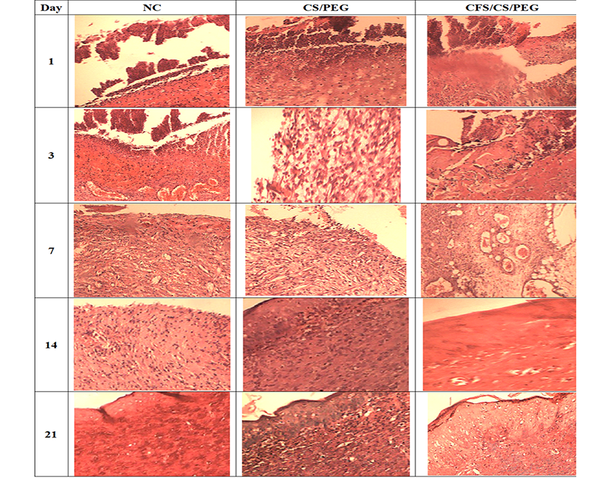
4.5. Changes in Gene Expression During Wound Healing
In the control group, the expression of TGF-β reached its peak on day 3 and remained constant afterward. In both dressing-treated groups, the expression of TGF-β was enhanced and increased gradually from day 1 to 21. Interestingly, the TGF- β level was significantly higher in the postbiotic group than in the chitosan group after day 3 until the end of the experiment (Table 4). The VEGF expression was the same on day 1 in all groups (P = 0.746). In the following days, its expression enhanced significantly in dressing-treated groups. Surprisingly, the postbiotic caused a higher VEGF expression than the chitosan film. In both dressing-treated groups, the maximum concentration of this cytokine was observed on day 14 (Table 4).
Mean ± SEM of Gene Expression in Wound Healing Process a
Groups | Day | ||||
---|---|---|---|---|---|
1 | 3 | 7 | 14 | 21 | |
TGF-β | |||||
Control | 0.581 ± 0.055 a | 0.981 ± 0.011 b | 0.986 ± 0.017 c | 0.979 ± 0.014 c | 0.969 ± 0.025 c |
Chitosan | 0.919 ± 0.067 a | 1.242 ± 0.019 a | 1.777 ± 0.026 b | 2.387 ± 0.076 b | 3.288 ± 0.03 b |
Postbiotic | 0.871 ± 0.022 a | 1.285 ± 0.046 a | 2.049 ± 0.062 a | 3.616 ± 0.035 a | 3.772 ± 0.039 a |
VEGF | |||||
Control | 0.520 ± 0.020 a | 0.980 ± 0.014 c | 0.96 ± 0.022 c | 0.975 ± 0.012 c | 0.983 ± 0.019 c |
Chitosan | 0.705 ± 0.065 a | 1.330 ± 0.010 b | 1.620 ± 0.020 b | 2.540 ± 0.030 b | 2.865 ± 0.035 b |
Postbiotic | 0.800 ± 0.100 a | 1.485 ± 0.015 a | 1.755 ± 0.025 a | 3.025 ± 0.095 a | 3.205 ± 0.067 a |
TNF-α | |||||
Control | 0.465 ± 0.045 b | 0.732 ± 0.011 b | 0.864 ± 0.013 a | 0.631 ± 0.020 a | 0.753 ± 0.037 a |
Chitosan | 0.986 ± 0.005 a | 0.615 ± 0.007 a | 0.778 ± 0.018 a | 0.965 ± 0.022 a | 0.645 ± 0.044 a |
Postbiotic | 0.857 ± 0.034 a | 0.826 ± 0.024 a | 0.662 ± 0.021 a | 0.832 ± 0.028 a | 0.589 ± 0.037 a |
IL-6 | |||||
Control | 0.829 ± 0.040 a | 0.983 ± 0.008 a | 0.983 ± 0.008 a | 0.983 ± 0.008 a | 0.983 ± 0.008 a |
Chitosan | 0.728 ± 0.030 a | 0.921 ± 0.009 a, b | 0.863 ± 0.010 b | 0.811 ± 0.010 b | 0.763 ± 0.012 b |
Postbiotic | 0.775 ± 0.077 a | 0.879 ± 0.016 b | 0.834 ± 0.013 b | 0.765 ± 0.015 b | 0.695 ± 0.032 b |
The maximum expression of TNF-α in dressing-treated groups was observed on day 1, which was significantly higher than in the control group (P = 0.0088 and P = 0.0033, respectively). In the control group, this cytokine expression peaked on day 3 and became constant afterward. After day 3, the level of TNF-α expression was the same in all groups (Table 4). The examination of IL-6 expression levels among different groups showed no difference on day 1 (P = 0.491). Expression of this cytokine increased significantly from day 1 to 3 in all groups. In the subsequent days, the level of this interleukin remained constant (Table 4).
5. Discussion
Lactic acid bacteria (LAB) are traditionally used to treat wounds in the far east due to their alleged antimicrobial activity (38, 39). They have antibacterial properties because of the secretion of metabolites such as bacteriocins, organic acids, fatty acids, and H2O2 (25). The H2O2 concentration of our postbiotic was 8.175%, which is lethal to pathogenic bacteria. Besides, L. reuteri improves the skin-tight barrier by directly affecting keratinocytes and also helps maintain skin integrity and health. The postbiotic based on this bacterium also inhibits the proliferation of pathogens, interferes with quorum sensing, and reduces the growth of pathogens (25, 40).
In this study, we used the postbiotic of L. reuteri to synthesize a novel dressing based on chitosan and PEG to improve the wound healing process. Chitosan is a biocompatible polymer with extensive antimicrobial capacity and is a good candidate for artificial skin preparation. This polymer has been confirmed to stimulate macrophages, increase cell proliferation, accelerate blood clotting, and block nerves to reduce pain at the site of trauma (41). Chitosan inhibits the penetration of inflammatory cells, increases the proliferation of fibroblasts, and helps organize collagen. Zhang et al. showed that PEG increased protein uptake and improved adhesion, cell growth, and proliferation in chitosan-based wound dressings (15). Therefore, in this study, we used PEG to improve the physicochemical properties of the dressing.
One of the key points in preparing wound dressing is exudate-controlled absorption. During the early stages of wound inflammation, inflammatory mediators such as histamine and bradykinin may cause vasodilation of the arteries and exudate production. Acute wound fluid stimulates innate immune cells to accelerate wound healing. In contrast, chronic wound fluid stops cell proliferation due to the high proteinase concentration and disintegrates growth factors involved in the healing process. Therefore, the proper absorption of exudates is essential in evaluating wound dressing. Another important factor influencing the performance of wound dressing is the WVTR. If the WVTR is high, the wound surface can dry out, which delays the healing process. Low WVTR causes the maceration of the wound and adjacent tissues, increasing the water vapor pressure and making the wound painful. The amount of WVTR for healthy skin is 0.279 kg/m2/day, which can increase to 5.138 kg/m2/day in the case of first-degree burns. Based on the investigations of Queen et al. (42), wound dressings with the transfer rate of 2 - 2.5 kg/m2/day could be suitable for keeping the wound moist and prevent from getting dry. In this experiment, both synthesized wound dressings showed appropriate WA and WVTR. Surprisingly, the postbiotic in structure of film did not alter these properties.
In 2016, Alemdaroglu et al. used chitosan gel formulations containing plant enzymes to treat burn wounds in rats. Their research discovered that chitosan gel, including plant enzymes, efficiently treated burn wounds (43). Our results revealed that a CS-PEG formulation containing L. reuteri postbiotic exhibited an effective therapeutic impact on wounds. Besides, two studies demonstrated that combining bioactive chemicals with chitosan gels could help treat a variety of wounds. The therapeutic efficacy of Bacillus subtilis sp. natto, L. reuteri, and L. fermentum cold cream on rat wounds was investigated by Golkar et al. (21). According to their findings, the formulation of Bacillus subtilis sp. natto and L. reuteri resulted in complete wound healing on day 14. The current study also found that using a CS/PEG film containing the L. reuteri postbiotic on day 14 could result in full wound closure. Zoghi et al. (44) found that the CS/PEG/glycerol film containing minocycline had suitable WVTR properties. Besides, CS/PEG/glycerol had good antimicrobial properties against S. aureus, P. aeruginosa, and B. subtilis. According to our findings, the produced film (CFS/CS/PEG) has suitable antibacterial and mechanical properties that make it acceptable for wound healing. These studies suggest that chitosan and polyethylene glycol are excellent ingredients for wound healing gels.
Neutrophils and macrophages are the first cells recruited to the lesion area. They produce bioactive compounds, but in case of long-time persistence, they can lead to extensive tissue damage, complicating the repair process. The presence of fibroblasts marks the proliferative phase. Fibroblasts decompose fibrin clots by producing matrix metalloproteinase, causing angiogenesis and extensive collagen deposition, eventually leading to the restoration of skin barrier function (45-47). Our results showed that the postbiotic films enhanced wound healing by accelerating the entry of neutrophils and macrophages and then limiting their infiltration in the following days. Fibroplasia was more rapid in the postbiotic group and associated with a more significant reduction in the wound area and greater and faster deposition of collagen and elastin filaments in ECM, which was consistent with the results of Poutahidis et al. (48). The films may improve the repair process and increase cell proliferation by immune-mediated toll-like receptors (TLRs). As known, TLRs are found on neutrophils, fibroblasts, monocytes, and macrophages and are required for wound healing. Besides, TLRs can inhibit fibroblast migration while boosting matrix metalloproteinases (MMPs), which help regulate wound healing (49).
Hashemikia et al. (50) developed a ciprofloxacin-loaded chitosan/polyethylene oxide/silica formulation and showed that it promotes accelerated wound healing in rats. They also discovered that the number of neutrophils and macrophages at the wound site rose in the early stages of wound development and then decreased. The current study's findings also revealed that the wound film (postbiotic/CS/PEG) causes a quick increase in neutrophils and macrophages. Compared to the Hashemikia study, the postbiotic/CS/PEG film resulted in more neutrophils and macrophages. Although the formulations utilized in these two experiments differed, they were both based on chitosan (50).
Moreover, TGF-β is one of the essential cytokines involved in wound healing that causes proliferation, differentiation, and chemotaxis of immune cells. It stimulates the formation of granulation tissues, angiogenesis, and collagen synthesis and deposition. Increased TGF-β expression in the postbiotic group caused faster immune cells infiltration and faster onset of inflammation and proliferation phases (51, 52). Also, VEGF has proangiogenic activity and is influential in neovascularization and granulation tissue formation. Besides, VEGF activates the microvascular endothelial cells (ECs) and promotes fibroblast migration (53). As shown, the postbiotic film enhanced VEGF expression during the wound healing process, which was reflected in faster wound closure and healing. Also, TNF-α is the other inflammatory cytokine that stimulates inflammation and angiogenesis (45, 54). The enhancement of TNF-α expression in the postbiotic groups logically led to faster infiltration of inflammatory cells in wound tissue.
In addition, IL-6 is a local regulator of the inflammatory process that acts as a systemic signal. A notable point was a significant increase in the expression of IL-6 cytokine in the control group from day 3 to the end of the experiment. Despite the constant expression of this cytokine, the number of macrophages and monocytes decreased significantly in all groups by the end of the 21 days. This could be due to the production of other modulating cytokines such as IL-10 by M2 macrophages. By inhibiting inflammatory responses, these cytokines stimulated fibroplasia and led to the synthesis of the collagen-rich extracellular matrix. It seems that the low level of IL-6 in dressing-treated groups is one of the reasons for the shorter inflammatory phase and quicker proliferative phase in these groups.
5.1. Conclusions
In conclusion, this study established a straightforward approach for constructing the CFS/CS/PEG film. The findings revealed that the postbiotic did not affect the water vapor absorption of wound dressings. Furthermore, the water vapor transmission test revealed that this formulation could be used as a dressing for wounds or burns with low exudate. The comparison of postbiotic/CS/PEG treatment with CS/PEG (without postbiotic) treatment and no treatment rat groups revealed accelerated wound healing. Our results showed that the CFS/CS/PEG film overregulated the expression of cytokines and chemokines during the healing process. Therefore, increased interleukins produced faster neutrophil and macrophage uptake and accelerated the inflammatory phase, resulting in faster wound healing. Besides, the CFS/CS/PEG film accelerated the deposition of collagen and elastin in the extracellular matrix and promoted the integrity of the wound area. As a whole, the findings suggest that the newly developed postbiotic formulation could be used as an adjunctive therapy to aid in wound healing.
Acknowledgements
References
-
1.
Ahuja A, Gupta J, Gupta R. Miracles of herbal phytomedicines in treatment of skin disorders: natural healthcare perspective. Infect Disord Drug Targets. 2021;21(3):328-38. [PubMed ID: 32568024]. https://doi.org/10.2174/1871526520666200622142710.
-
2.
Yin M, Wan S, Ren X, Chu CC. Development of inherently antibacterial, biodegradable, and biologically active chitosan/pseudo-protein hybrid hydrogels as biofunctional wound dressings. ACS Appl Mater Interfaces. 2021;13(12):14688-99. [PubMed ID: 33739108]. https://doi.org/10.1021/acsami.0c21680.
-
3.
Bertesteanu S, Triaridis S, Stankovic M, Lazar V, Chifiriuc MC, Vlad M, et al. Polymicrobial wound infections: pathophysiology and current therapeutic approaches. Int J Pharm. 2014;463(2):119-26. [PubMed ID: 24361265]. https://doi.org/10.1016/j.ijpharm.2013.12.012.
-
4.
Han G, Ceilley R. Chronic Wound Healing: A Review of current management and treatments. Adv Ther. 2017;34(3):599-610. [PubMed ID: 28108895]. [PubMed Central ID: PMC5350204]. https://doi.org/10.1007/s12325-017-0478-y.
-
5.
Landen NX, Li D, Stahle M. Transition from inflammation to proliferation: a critical step during wound healing. Cell Mol Life Sci. 2016;73(20):3861-85. [PubMed ID: 27180275]. [PubMed Central ID: PMC5021733]. https://doi.org/10.1007/s00018-016-2268-0.
-
6.
Yanai H, Budovsky A, Tacutu R, Barzilay T, Abramovich A, Ziesche R, et al. Tissue repair genes: the TiRe database and its implication for skin wound healing. Oncotarget. 2016;7(16):21145-55. [PubMed ID: 27049721]. [PubMed Central ID: PMC5008274]. https://doi.org/10.18632/oncotarget.8501.
-
7.
Naseri-Nosar M, Ziora ZM. Wound dressings from naturally-occurring polymers: A review on homopolysaccharide-based composites. Carbohydr Polym. 2018;189:379-98. [PubMed ID: 29580422]. https://doi.org/10.1016/j.carbpol.2018.02.003.
-
8.
Anjum S, Arora A, Alam MS, Gupta B. Development of antimicrobial and scar preventive chitosan hydrogel wound dressings. Int J Pharm. 2016;508(1-2):92-101. [PubMed ID: 27163526]. https://doi.org/10.1016/j.ijpharm.2016.05.013.
-
9.
Zhang C, Hui D, Du C, Sun H, Peng W, Pu X, et al. Preparation and application of chitosan biomaterials in dentistry. Int J Biol Macromol. 2021;167:1198-210. [PubMed ID: 33202273]. https://doi.org/10.1016/j.ijbiomac.2020.11.073.
-
10.
Khajavian M, Vatanpour V, Castro-Munoz R, Boczkaj G. Chitin and derivative chitosan-based structures - Preparation strategies aided by deep eutectic solvents: A review. Carbohydr Polym. 2022;275:118702. [PubMed ID: 34742428]. https://doi.org/10.1016/j.carbpol.2021.118702.
-
11.
Sánchez-Machado DI, López-Cervantes J, Martínez-Ibarra DM, Escárcega-Galaz AA, Vega-Cázarez CA. The use of chitosan as a skin-regeneration agent in burns injuries: A review. e-Polymers. 2022;22(1):75-86. https://doi.org/10.1515/epoly-2022-0011.
-
12.
Du X, Liu Y, Yan H, Rafique M, Li S, Shan X, et al. Anti-infective and pro-coagulant chitosan-based hydrogel tissue adhesive for sutureless wound closure. Biomacromolecules. 2020;21(3):1243-53. [PubMed ID: 32045224]. https://doi.org/10.1021/acs.biomac.9b01707.
-
13.
Mishra SK, Mary DS, Kannan S. Copper incorporated microporous chitosan-polyethylene glycol hydrogels loaded with naproxen for effective drug release and anti-infection wound dressing. Int J Biol Macromol. 2017;95:928-37. [PubMed ID: 27984151]. https://doi.org/10.1016/j.ijbiomac.2016.10.080.
-
14.
Sta Agueda JRH, Chen Q, Maalihan RD, Ren J, da Silva IGM, Dugos NP, et al. 3D printing of biomedically relevant polymer materials and biocompatibility. MRS Commun. 2021;11(2):1-16. [PubMed ID: 33936866]. [PubMed Central ID: PMC8075026]. https://doi.org/10.1557/s43579-021-00038-8.
-
15.
Zhang M, Li XH, Gong YD, Zhao NM, Zhang XF. Properties and biocompatibility of chitosan films modified by blending with PEG. Biomaterials. 2002;23(13):2641-8. https://doi.org/10.1016/s0142-9612(01)00403-3.
-
16.
Buranachai T, Praphairaksit N, Muangsin N. Chitosan/polyethylene glycol beads crosslinked with tripolyphosphate and glutaraldehyde for gastrointestinal drug delivery. AAPS PharmSciTech. 2010;11(3):1128-37. [PubMed ID: 20652459]. [PubMed Central ID: PMC2974148]. https://doi.org/10.1208/s12249-010-9483-z.
-
17.
Wang Q, Dong Z, Du Y, Kennedy JF. Controlled release of ciprofloxacin hydrochloride from chitosan/polyethylene glycol blend films. Carbohydr Polym. 2007;69(2):336-43. https://doi.org/10.1016/j.carbpol.2006.10.014.
-
18.
Wang J, Hon M. Preparation of poly (ethylene glycol)/chitosan membranes by a glucose-mediating process and in vitro drug release. J Appl Polym Sci. 2005;96(4):1083-94. https://doi.org/10.1002/app.21506.
-
19.
Cai M, Zhang J, Chen Y, Cao J, Leng M, Hu S, et al. Preparation and characterization of chitosan composite membranes crosslinked by carboxyl-capped poly(ethylene glycol). Chinese J Polym Sci. 2014;32(2):236-44. https://doi.org/10.1007/s10118-014-1373-5.
-
20.
Castiblanco GA, Yucel-Lindberg T, Roos S, Twetman S. Effect of Lactobacillus reuteri on cell viability and pge2 production in human gingival fibroblasts. Probiotics Antimicrob Proteins. 2017;9(3):278-83. [PubMed ID: 28028690]. https://doi.org/10.1007/s12602-016-9246-6.
-
21.
Golkar N, Ashoori Y, Heidari R, Omidifar N, Abootalebi SN, Mohkam M, et al. A novel effective formulation of bioactive compounds for wound healing: preparation, in vivo characterization, and comparison of various postbiotics cold creams in a rat model. Evid Based Complement Alternat Med. 2021;2021:8577116. [PubMed ID: 34917159]. [PubMed Central ID: PMC8670929]. https://doi.org/10.1155/2021/8577116.
-
22.
Aguilar-Toalá JE, Garcia-Varela R, Garcia HS, Mata-Haro V, González-Córdova AF, Vallejo-Cordoba B, et al. Postbiotics: An evolving term within the functional foods field. Trends Food Sci Technol. 2018;75:105-14. https://doi.org/10.1016/j.tifs.2018.03.009.
-
23.
Zolkiewicz J, Marzec A, Ruszczynski M, Feleszko W. Postbiotics-A Step Beyond Pre- and Probiotics. Nutrients. 2020;12(8). [PubMed ID: 32717965]. [PubMed Central ID: PMC7468815]. https://doi.org/10.3390/nu12082189.
-
24.
Bano I, Arshad M, Yasin T, Ghauri MA, Younus M. Chitosan: A potential biopolymer for wound management. Int J Biol Macromol. 2017;102:380-3. [PubMed ID: 28412341]. https://doi.org/10.1016/j.ijbiomac.2017.04.047.
-
25.
Lukic J, Chen V, Strahinic I, Begovic J, Lev-Tov H, Davis SC, et al. Probiotics or pro-healers: the role of beneficial bacteria in tissue repair. Wound Repair Regen. 2017;25(6):912-22. [PubMed ID: 29315980]. [PubMed Central ID: PMC5854537]. https://doi.org/10.1111/wrr.12607.
-
26.
Maghsood F, Mirshafiey A, Farahani MM, Modarressi MH, Jafari P, Motevaseli E. Dual effects of cell free supernatants from Lactobacillus acidophilus and Lactobacillus rhamnosus GG in regulation of MMP-9 by up-regulating TIMP-1 and down-regulating CD147 in PMA-differentiated THP-1 cells. Cell J (Yakhteh). 2018;19(4):559.
-
27.
Bradford MM. A rapid and sensitive method for the quantitation of microgram quantities of protein utilizing the principle of protein-dye binding. Anal Biochem. 1976;72(1-2):248-54. https://doi.org/10.1016/0003-2697(76)90527-3.
-
28.
Coda R, Cassone A, Rizzello CG, Nionelli L, Cardinali G, Gobbetti M. Antifungal activity of Wickerhamomyces anomalus and Lactobacillus plantarum during sourdough fermentation: identification of novel compounds and long-term effect during storage of wheat bread. Appl Environ Microbiol. 2011;77(10):3484-92. [PubMed ID: 21441340]. [PubMed Central ID: PMC3126437]. https://doi.org/10.1128/AEM.02669-10.
-
29.
Lee Y, Chang J, Yang M, Chien C, Lai W. Acceleration of wound healing in diabetic rats by layered hydrogel dressing. Carbohydr Polym. 2012;88(3):809-19. https://doi.org/10.1016/j.carbpol.2011.12.045.
-
30.
Ko S, Janes ME, Hettiarachchy NS, Johnson MG. Physical and chemical properties of edible films containing nisin and their action against listeria monocytogenes. J Food Sci. 2001;66(7):1006-11. https://doi.org/10.1111/j.1365-2621.2001.tb08226.x.
-
31.
Albus U. Guide for the Care and Use of Laboratory Animals. 8th ed. London, England: SAGE Publications; 2012.
-
32.
Barreto RS, Quintans JS, Barreto AS, Albuquerque-Junior RL, Galvao JG, Gonsalves JK, et al. Improvement of wound tissue repair by chitosan films containing (-)-borneol, a bicyclic monoterpene alcohol, in rats. Int Wound J. 2016;13(5):799-808. [PubMed ID: 25471005]. [PubMed Central ID: PMC7949920]. https://doi.org/10.1111/iwj.12385.
-
33.
Wang L, Qin W, Zhou Y, Chen B, Zhao X, Zhao H, et al. Transforming growth factor β plays an important role in enhancing wound healing by topical application of Povidone-iodine. Sci Rep. 2017;7(1):1-8. https://doi.org/10.1038/s41598-017-01116-5.
-
34.
Mehrabani M, Seyyedkazemi SM, Nematollahi MH, Jafari E, Mehrabani M, Mehdipour M, et al. Accelerated burn wound closure in mice with a new formula based on traditional medicine. Iran Red Crescent Med J. 2016;18(11). https://doi.org/10.5812/ircmj.26613.
-
35.
Selzner M, Rudiger HA, Sindram D, Madden J, Clavien PA. Mechanisms of ischemic injury are different in the steatotic and normal rat liver. Hepatology. 2000;32(6):1280-8. [PubMed ID: 11093735]. https://doi.org/10.1053/jhep.2000.20528.
-
36.
Canene-Adams K. Preparation of formalin-fixed paraffin-embedded tissue for immunohistochemistry. Methods in enzymology. 2013/11/05 ed. Elsevier; 2013. p. 225-33.
-
37.
Kornhauser SI. Orcein and elastic fibers. Stain Technol. 1952;27(3):131-4. [PubMed ID: 14958504]. https://doi.org/10.3109/10520295209105073.
-
38.
Li D, Ni K, Pang H, Wang Y, Cai Y, Jin Q. Identification and antimicrobial activity detection of lactic Acid bacteria isolated from corn stover silage. Asian-Australas J Anim Sci. 2015;28(5):620-31. [PubMed ID: 25924957]. [PubMed Central ID: PMC4412991]. https://doi.org/10.5713/ajas.14.0439.
-
39.
Halper J, Leshin LS, Lewis SJ, Li WI. Wound healing and angiogenic properties of supernatants from Lactobacillus cultures. Exp Biol Med (Maywood). 2003;228(11):1329-37. [PubMed ID: 14681548]. https://doi.org/10.1177/153537020322801111.
-
40.
Bian L, Molan A, Maddox I, Shu Q. Antimicrobial activity of Lactobacillus reuteri DPC16 supernatants against selected food borne pathogens. World J Microbiol Biotechnol. 2011;27(4):991-8. https://doi.org/10.1007/s11274-010-0543-z.
-
41.
Ahmed S, Ikram S. Chitosan based scaffolds and their applications in wound healing. Achiev Life Sci. 2016;10(1):27-37. https://doi.org/10.1016/j.als.2016.04.001.
-
42.
Queen D, Gaylor JD, Evans JH, Courtney JM, Reid WH. The preclinical evaluation of the water vapour transmission rate through burn wound dressings. Biomaterials. 1987;8(5):367-71. [PubMed ID: 3676423]. https://doi.org/10.1016/0142-9612(87)90007-x.
-
43.
Alemdaroglu C, Degim Z, Celebi N, Zor F, Ozturk S, Erdogan D. An investigation on burn wound healing in rats with chitosan gel formulation containing epidermal growth factor. Burns. 2006;32(3):319-27. [PubMed ID: 16527411]. https://doi.org/10.1016/j.burns.2005.10.015.
-
44.
Zoghi N, Fouani MH, Bagheri H, Nikkhah M, Asadi N. Characterization of minocycline loaded chitosan/polyethylene glycol/glycerol blend films as antibacterial wound dressings. J Appl Polym Sci. 2021;138(32). https://doi.org/10.1002/app.50781.
-
45.
Ritsu M, Kawakami K, Kanno E, Tanno H, Ishii K, Imai Y, et al. Critical role of tumor necrosis factor-α in the early process of wound healing in skin. J Dermatol Dermatol Surg. 2017;21(1):14-9. https://doi.org/10.1016/j.jdds.2016.09.001.
-
46.
Tagliari E, Campos LF, Campos AC, Costa-Casagrande TA, Noronha L. Effect of probiotic oral administration on skin wound healing in rats. Arq Bras Cir Dig. 2019;32(3). e1457. [PubMed ID: 31826084]. [PubMed Central ID: PMC6902899]. https://doi.org/10.1590/0102-672020190001e1457.
-
47.
Bainbridge P. Wound healing and the role of fibroblasts. J Wound Care. 2013;22(8):407-8. 410-12. [PubMed ID: 23924840]. https://doi.org/10.12968/jowc.2013.22.8.407.
-
48.
Poutahidis T, Kearney SM, Levkovich T, Qi P, Varian BJ, Lakritz JR, et al. Microbial symbionts accelerate wound healing via the neuropeptide hormone oxytocin. PLoS One. 2013;8(10). e78898. [PubMed ID: 24205344]. [PubMed Central ID: PMC3813596]. https://doi.org/10.1371/journal.pone.0078898.
-
49.
Mokhtari Y, Pourbagheri-Sigaroodi A, Zafari P, Bagheri N, Ghaffari SH, Bashash D. Toll-like receptors (TLRs): An old family of immune receptors with a new face in cancer pathogenesis. J Cell Mol Med. 2021;25(2):639-51. [PubMed ID: 33336901]. [PubMed Central ID: PMC7812258]. https://doi.org/10.1111/jcmm.16214.
-
50.
Hashemikia S, Farhangpazhouh F, Parsa M, Hasan M, Hassanzadeh A, Hamidi M. Fabrication of ciprofloxacin-loaded chitosan/polyethylene oxide/silica nanofibers for wound dressing application: In vitro and in vivo evaluations. Int J Pharm. 2021;597:120313. [PubMed ID: 33540002]. https://doi.org/10.1016/j.ijpharm.2021.120313.
-
51.
Savari R, Shafiei M, Galehdari H, Kesmati M. Expression of VEGF and TGF-β genes in skin wound healing process induced using phenytoin in male rats. Jundishapur J Health Sci. 2019;11(1). https://doi.org/10.5812/jjhs.86041.
-
52.
Ebrahiminaseri A, Sadeghizadeh M, Moshaii A, Asgaritarghi G, Safari Z. Combination treatment of dendrosomal nanocurcumin and low-level laser therapy develops proliferation and migration of mouse embryonic fibroblasts and alter TGF-beta, VEGF, TNF-alpha and IL-6 expressions involved in wound healing process. PLoS One. 2021;16(5). e0247098. [PubMed ID: 33956815]. [PubMed Central ID: PMC8101758]. https://doi.org/10.1371/journal.pone.0247098.
-
53.
Moreira HR, Marques AP. Vascularization in skin wound healing: where do we stand and where do we go? Curr Opin Biotechnol. 2022;73:253-62. [PubMed ID: 34555561]. https://doi.org/10.1016/j.copbio.2021.08.019.
-
54.
Zhang C. The role of inflammatory cytokines in endothelial dysfunction. Basic Res Cardiol. 2008;103(5):398-406. [PubMed ID: 18600364]. [PubMed Central ID: PMC2705866]. https://doi.org/10.1007/s00395-008-0733-0.