Abstract
Background:
Fast and precise detection of SARS-CoV-2 RNA in clinical samples and subsequent quarantine are two critical factors in preventing virus transmission and distribution through the community. The false-negative result is a major problem in the SARS-CoV-2 detection because of the kind of sample (swab sample), sampling error, and sensitivity of PCR test, which can be reduced by a much more sensitive test such as nested PCR.Objectives:
This study aimed to evaluate the false-negative rate among samples that were negative by a real-time PCR test using RT-nested PCR.Methods:
One hundred eighty-four negative samples were included in the study, and nucleic acid was extracted using a commercial kit based on a silica filter column and then subjected to RT-nested PCR using three sets of primers targeting Orf1ab, N, and RdRp regions.Results:
Among 184 negative swab samples for SARS-CoV-2, 27 (14.6%) cases were positive for the Orf1ab gene using RT-nested PCR. The samples were tested using N and RdRp primer sets. Also, seven (3.8%) cases were positive for the N gene, and four (2.1%) cases were positive for the RdRp gene.Conclusions:
The results indicated that RT-nested PCR could be more sensitive than real-time PCR and reduce the false-negative rate.Keywords
1. Background
Severe acute respiratory syndrome Coronavirus 2 (SARS-COV-2) is a virus with positive single-stranded RNA as a genome and is responsible for the worldwide COVID-19 pandemic that started in Wuhan, China. SARS-CoV-2 is a beta-coronavirus of the Coronaviridae family (1). The genome structure and sequence of SARS-CoV-2 indicate high similarity with those of SARS coronavirus 1 and have several structural and nonstructural proteins that have a pivotal role in virus replication, pathogenicity, and transmission (2, 3). RNA synthesis is a necessary process that involves several steps, including genome replication and sub-genomic RNA transcription. Viral RNA synthesis is not a perfect process, and some errors such as point mutation and recombination can occur during the process, which can affect the detection process by PCR detection kits (4).
Some processes, such as sequencing the SARS-CoV-2 genome in patient's samples, provide beneficial information about viral genome characteristics, reducing false negatives, and increasing the sensitivity and specificity of real-time PCR kits (5). Orf 1ab, N, and RdRp are three regions mainly used for virus detection in different PCR diagnostic kits (6). In some studies, the S region was also used for detection, but the false-negative rate increased compared to that in the other regions (7, 8). On the other hand, the sensitivity of the PCR test is another factor that can affect the rate of false negative. Several studies have revealed that real-time PCR is not the best technique to diagnose infectious agents such as SARS-CoV-2. Nested PCR can indicate sensitivity ten times better than real-time polymerase chain reaction (RT-PCR) (9). A decrease in the false-negative rate can help prevent virus transmission through the community and disrupt the replication cycle of the virus.
2. Objectives
This study aimed to evaluate samples of patients with symptoms of COVID-19 but negative real-time PCR results. Of interest was also to test the samples again to detect the presence of viral RNA using the nested PCR method.
3. Methods
One hundred eighty-four nasopharyngeal swab samples of patients with negative SARS-COV-2 PCR results were collected and stored at -80°C in the laboratory. All the cases had negative real-time PCR test results for SARS-CoV-2 but had symptoms like fever, sneezing, myalgia, and headache. All the specimens were tested using a real-time PCR kit (Pishtaz Teb, Iran) that targeted the RdRp and N genes of the detected viral genome.
3.1. Primer Design
The outer set of PCR primers was used for the previous study (10). Inner primers were designed based on first-round products using NCBI primer blast software. All the primers were then checked using in silico PCR and primer blast software. The primers are indicated in Table 1.
SARS-CoV-2 Designed Primer for RT-Nested PCR Targeting Orf1ab, N, and RdRp Genes
Gene and Amplicon Size | Primer Sequence |
---|---|
Orf1ab | |
200bp | Outer F: GTTACCTTCTCTTGCCACTGT |
Outer R: TCCTAGCACCATCATCATACA | |
120bp | Inner F: TGACATGGTTGGATATGGTTGA |
Inner R: CATCATCATACACAGTTCTTGC | |
N | |
151bp | Outer F: CCTCTTCTCGTTCCTCATCAC |
Outer R: CTCTCAAGCTGGTTCAATCTGT | |
80bp | Inner F: CAACTCCAGGCAGCAGTAG |
Inner R: CAGCAGCAAAGCAAGAGC | |
RdRp | |
190bp | Outer F: ATCTCACTTGCTGGTTCCTAT |
Outer R: TAGTCCTCACTTCTCTCAAAGA | |
160bp | Inner F: GGTCCTATTCTGGACAATCTAC |
Inner R: GTCCTCACTTCTCTCAAAGAAA |
3.2. RNA Extraction
The extraction process was done using the viral RNA extraction kit (Rojeh, IRAN) according to the manufacturer's instructions. Briefly, 560 µL lysis buffer supplemented with carrier RNA and 140 µL of viral transport media were mixed and incubated at room temperature for 10 min. After incubation, 560 µL ethanol was added to the mixture, which was then transferred to the filter tube after the vortex. The filter columns were washed with wash buffers 1 and 2, and finally, the nucleic acid was eluted into a 60 µL elution buffer.
3.3. RT-Nested PCR
All the extracted negative samples were subjected to cDNA synthesis using First Strand cDNA Synthesis Kit (SinaClon, Iran) according to the manufacturer's instructions. The synthesized cDNA was subjected to the first round of nested PCR with external sets of Orf1ab, N, and RdRp primers to evaluate potential positive samples among the negatives. The PCR condition was as follows: (1) 10 pmol of each primer; (2) 12.5 µL of hot start 2xPCR master mix (amplicon, Denmark); (3) 2.5 µL synthesized cDNA as template; and (4) water were mixed in a PCR tube to complete 25 µL PCR reaction and subjected to 35 cycle PCR with the following program: (1) initial denaturation at 95°C for 2 min; (2) denaturation at 94°C for 30 sec; (3) annealing at 55°C for 30 sec; and (4) extension at 72°C for 30 sec. Second-round PCR was done the same as the first-round except for primers and template measurement. The inner set of primers was used, and 1µL of the first-round PCR product was added to the second-round PCR mix as a template. The second-round PCR products were subjected to electrophoresis on 2% agarose gel for gene-specific amplicon detection. Amplicons with sizes of 120 bp, 80 bp, and 160 bp were detected for Orf1ab, N, and RdRp genes, respectively. A positive real-time PCR result sample was used as a positive control, and distilled water was used as a negative control.
4. Results and Discussion
In previous reports, the Orf1ab region showed better results in SARS-CoV-2 detection (6, 10). Thus, Orf1ab was firstly used to evaluate negative samples. Among 184 negative swab samples from SARS-CoV-2, 27 (14.6%) cases were positive for the Orf1ab gene. These samples were tested by N and RdRp primer sets. Seven (3.8%) cases were positive for the N gene, and four (2.1%) cases were positive for the RdRp gene (Figure 1). Best results in SARS-CoV-2 detection using nested PCR were obtained with an Orf1ab primer. Electrophoresis results of all the genes are observable in Figure 2. SARS-CoV-2 pandemics put high and unpredictable pressure on different aspects of human life, such as health, economy, and social relationships. The best way to control the pandemic based on the WHO recommendations is to consider social distance and vaccination (11). However, fast and precise virus detection is still essential to quarantine infected people and prevent fast transmission in the community (12).
The most reliable and fast technique to detect the SARS-CoV-2 viral genome is real-time PCR, which is based on detecting different viral genes such as RdRp, N, S, Orf1ab, and E. Among them, Orf1ab, N, and RdRp are very frequent in different real-time PCR kits (13). One of the recurring problems in the SARS-CoV-2 detection is the false-negative rate. This rate highly causes sample kind (swab sample), sampling site, sampling technique, and sensitivity of PCR kits (13-16). In this study, 184 samples with negative real-time PCR test results were collected and tested again using nested PCR as a much more sensitive technique than real-time PCR to evaluate possible positive samples among negative real-time PCR samples.
Rate of positive samples using nested PCR among samples with negative real-time PCR results

Nested PCR results. A, Orf1ab gene with 120 bp PCR product; B, N gene with 80 bp PCR product; C, RdRp gene with 160 bp PCR product. 100 bp ladder used for electrophoresis.
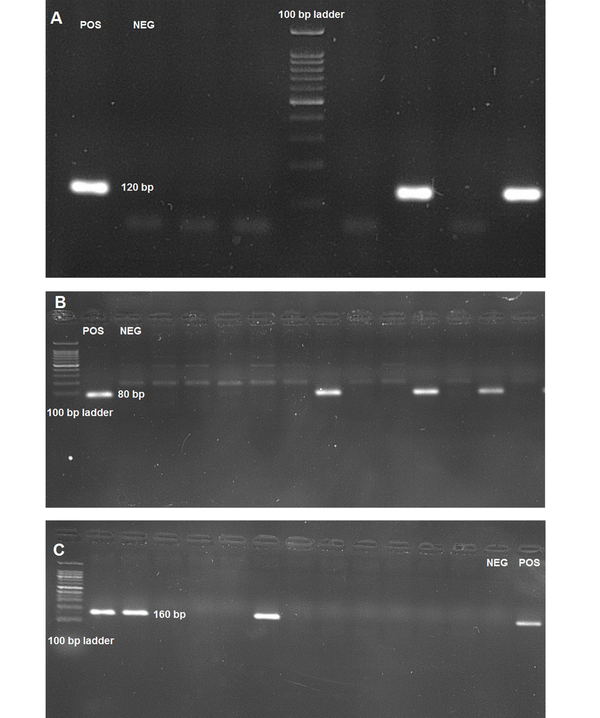
Our results showed that 14.6% (27 samples) of the 184 cases with negative real-time PCR test results were positive with nested PCR using the Orf1ab primer set. Among the 27 samples, seven (3.8%) cases were positive with the N primer set, and four (2.1%) cases were positive using the RdRp primer set. These results indicated a 14.6% false negative in real-time PCR results of the Pishtaz Teb SARS-CoV-2 detection kit. Our results are in line with the results from Davda et al.'s study. They reported 13% false-negative among samples with negative real-time PCR results (17). Also, Wang et al. reported 14 positive real-time PCR results among 156 samples (8.97%) with negative real-time PCR results (9). The difference between the results can be because of differences in real-time PCR kit sensitivity, PCR instrument detection sensitivity, and region of viral genome targeting to primer design.
Wang et al. (9), in their study, used a Sansure qPCR kit for virus detection. In this kit, Orf1ab and N regions were targeted for primer design, but in the Pishtaz Teb real-time PCR kit used in this study, RdRp and N genes were targeted for primer design and detection. Some studies revealed that Orf1ab was a better choice than RdRp for primer design and indicated a better positivity rate, which is in line with our results (18). These results reveal that nested PCR can be a good alternative to real-time PCR because it can be up to 10 times more sensitive than PCR (9). The most significant negative aspect of the nested PCR technique is the risk of contamination. Other studies resolved this problem using a single tube real-time nested PCR (19-21). These approaches can decrease the false-negative rate of SARS-CoV-2 detection, thereby decreasing the transmission rate. Sample size, use of only three genes for virus detection, and sequencing could be mentioned as limitations of the study. Sequencing could be considered to detect possible point mutations, insertion, and deletions affecting PCR kit detection.
5. Conclusions
RT-nested PCR can be more sensitive than real-time PCR and reduce the false-negative rate.
Acknowledgements
References
-
1.
Harrison AG, Lin T, Wang P. Mechanisms of SARS-CoV-2 transmission and pathogenesis. Trends Immunol. 2020;41(12):1100-15. [PubMed ID: 33132005]. [PubMed Central ID: PMC7556779]. https://doi.org/10.1016/j.it.2020.10.004.
-
2.
Segreto R, Deigin Y. The genetic structure of SARS-CoV-2 does not rule out a laboratory origin: SARS-COV-2 chimeric structure and furin cleavage site might be the result of genetic manipulation. Bioessays. 2021;43(3). e2000240. [PubMed ID: 33200842]. [PubMed Central ID: PMC7744920]. https://doi.org/10.1002/bies.202000240.
-
3.
Kadam SB, Sukhramani GS, Bishnoi P, Pable AA, Barvkar VT. SARS-CoV-2, the pandemic coronavirus: Molecular and structural insights. J Basic Microbiol. 2021;61(3):180-202. [PubMed ID: 33460172]. [PubMed Central ID: PMC8013332]. https://doi.org/10.1002/jobm.202000537.
-
4.
Kottier SA, Cavanagh D, Britton P. Experimental evidence of recombination in coronavirus infectious bronchitis virus. Virology. 1995;213(2):569-80. [PubMed ID: 7491781]. [PubMed Central ID: PMC7131336]. https://doi.org/10.1006/viro.1995.0029.
-
5.
Moore SC, Penrice-Randal R, Alruwaili M, Randle N, Armstrong S, Hartley C, et al. Amplicon-based detection and sequencing of SARS-CoV-2 in nasopharyngeal swabs from patients with COVID-19 and identification of deletions in the viral genome that encode proteins involved in interferon antagonism. Viruses. 2020;12(10):1164. [PubMed ID: 33066701]. [PubMed Central ID: PMC7602519]. https://doi.org/10.3390/v12101164.
-
6.
Rahbari R, Moradi N, Abdi M. rRT-PCR for SARS-CoV-2: Analytical considerations. Clin Chim Acta. 2021;516:1-7. [PubMed ID: 33485902]. [PubMed Central ID: PMC7826022]. https://doi.org/10.1016/j.cca.2021.01.011.
-
7.
Wang R, Hozumi Y, Yin C, Wei GW. Mutations on COVID-19 diagnostic targets. Genomics. 2020;112(6):5204-13. [PubMed ID: 32966857]. [PubMed Central ID: PMC7502284]. https://doi.org/10.1016/j.ygeno.2020.09.028.
-
8.
Zhou P, Yang XL, Wang XG, Hu B, Zhang L, Zhang W, et al. A pneumonia outbreak associated with a new coronavirus of probable bat origin. Nature. 2020;579(7798):270-3. [PubMed ID: 32015507]. [PubMed Central ID: PMC7095418]. https://doi.org/10.1038/s41586-020-2012-7.
-
9.
Wang J, Cai K, Zhang R, He X, Shen X, Liu J, et al. Novel one-step single-tube nested quantitative real-time pcr assay for highly sensitive detection of SARS-CoV-2. Anal Chem. 2020;92(13):9399-404. [PubMed ID: 32438806]. [PubMed Central ID: PMC7299395]. https://doi.org/10.1021/acs.analchem.0c01884.
-
10.
Mollaei HR, Afshar AA, Kalantar-Neyestanaki D, Fazlalipour M, Aflatoonian B. Comparison five primer sets from different genome region of COVID-19 for detection of virus infection by conventional RT-PCR. Iran J Microbiol. 2020;12(3):185-93. [PubMed ID: 32685113]. [PubMed Central ID: PMC7340604].
-
11.
World Health Organization. Advice for the public: Coronavirus disease (COVID-19). Geneva, Switzerland: World Health Organization; 2021, [updated 10 May 2022; cited 1 Oct 2021]. Available from: https://www.who.int/emergencies/diseases/novel-coronavirus-2019/advice-for-public.
-
12.
Rong XM, Yang L, Chu HD, Fan M. Effect of delay in diagnosis on transmission of COVID-19. Math Biosci Eng. 2020;17(3):2725-40. [PubMed ID: 32233563]. https://doi.org/10.3934/mbe.2020149.
-
13.
Yang J, Han Y, Zhang R, Zhang R, Li J. Comparison of analytical sensitivity of SARS-CoV-2 molecular detection kits. Int J Infect Dis. 2021;111:233-41. [PubMed ID: 34428543]. [PubMed Central ID: PMC8379823]. https://doi.org/10.1016/j.ijid.2021.08.043.
-
14.
Espy MJ, Uhl JR, Sloan LM, Buckwalter SP, Jones MF, Vetter EA, et al. Real-time PCR in clinical microbiology: applications for routine laboratory testing. Clin Microbiol Rev. 2006;19(1):165-256. [PubMed ID: 16418529]. [PubMed Central ID: PMC1360278]. https://doi.org/10.1128/CMR.19.1.165-256.2006.
-
15.
Lippi G, Lima-Oliveira G, Brocco G, Bassi A, Salvagno GL. Estimating the intra- and inter-individual imprecision of manual pipetting. Clin Chem Lab Med. 2017;55(7):962-6. [PubMed ID: 27816957]. https://doi.org/10.1515/cclm-2016-0810.
-
16.
Lippi G, von Meyer A, Cadamuro J, Simundic AM, European Federation of Clinical C, Laboratory Medicine Working Group for Preanalytical P. PREDICT: a checklist for preventing preanalytical diagnostic errors in clinical trials. Clin Chem Lab Med. 2020;58(4):518-26. [PubMed ID: 31758854]. https://doi.org/10.1515/cclm-2019-1089.
-
17.
Davda JN, Frank K, Prakash S, Purohit G, Vijayashankar DP, Vedagiri D, et al. An inexpensive rt-pcr endpoint diagnostic assay for SARS-CoV-2 using nested PCR: direct assessment of detection efficiency of RT-qPCR tests and suitability for surveillance. bioRxiv. 2020;Preprint.
-
18.
Niu P, Lu R, Zhao L, Wang H, Huang B, Ye F, et al. Three novel real-time RT-PCR assays for detection of COVID-19 virus. China CDC Wkly. 2020;2(25):453-7. [PubMed ID: 34594677]. [PubMed Central ID: PMC8393056]. https://doi.org/10.46234/ccdcw2020.116.
-
19.
Zhang Y, Dai C, Wang H, Gao Y, Li T, Fang Y, et al. Analysis and validation of a highly sensitive one-step nested quantitative real-time polymerase chain reaction assay for specific detection of severe acute respiratory syndrome coronavirus 2. Virol J. 2020;17(1):197. [PubMed ID: 33371898]. [PubMed Central ID: PMC7768088]. https://doi.org/10.1186/s12985-020-01467-y.
-
20.
Yip CC, Sridhar S, Leung KH, Ng AC, Chan KH, Chan JF, et al. Development and evaluation of novel and highly sensitive single-tube nested real-time RT-PCR assays for SARS-CoV-2 detection. Int J Mol Sci. 2020;21(16):5674. [PubMed ID: 32784770]. [PubMed Central ID: PMC7461039]. https://doi.org/10.3390/ijms21165674.
-
21.
Meza-Robles C, Barajas-Saucedo CE, Tiburcio-Jimenez D, Mokay-Ramirez KA, Melnikov V, Rodriguez-Sanchez IP, et al. One-step nested RT-PCR for COVID-19 detection: A flexible, locally developed test for SARS-CoV2 nucleic acid detection. J Infect Dev Ctries. 2020;14(7):679-84. [PubMed ID: 32794453]. https://doi.org/10.3855/jidc.12726.