Abstract
Background:
The genus Proteus is a Gram-negative bacterium with a unique characteristic of swarming. Mainly three species are involved in initiating urinary tract infections in the community and in immunocompromised patients, particularly in patients going through long-term catheterization. Due to their strong virulence factors like biofilm formations, protease, and hemolysin, they can lead to lengthening infections in affected individuals. Probiotics are live bacteria and yeasts that are beneficial to human health and can be used as an alternative for the control of nosocomial diseases. Lactobacilli are one of the common probiotics mostly found in yogurt and other fermented foods that have been used as a substitute for infection control.Objectives:
The current study was designed to screen potential probiotic bacteria to encounter antibiotic-resistant and virulent Proteus species.Methods:
In the current study, using probiotics, already known antibiotic-resistant isolates (n = 25) of Proteus were processed to characterize their virulence factors and their inhibition. Biofilm formation, protease, and hemolysin activities were studied using different phenotypic detection methods. Further, their virulence genes zapA, flg, hmpA, mrp, and rsbA were explored using their genomic DNA. These isolates were found resistant to different classes of antibiotics, and a strategy was designed to inhibit their growth by using probiotic bacteria isolated from the soil.Results:
Virulence factors first, all isolates were subjected to biofilm detection, and they were 32% (n = 8) strong, 40% (n = 10) moderate, 16% (n = 4) weak, and 12% (n = 3) non-biofilm producers. All isolates were positive for swarming activity by showing a differentiated ring form of growth. Protease activity showed 56% (n = 14) isolates. Only 24% (n = 6) of isolates were positive for hemolysin. Virulence factors and molecular mechanisms were studied, and gene rsbA responsible for swarming was amplified in 17 (68%) Proteus isolates, and mrp responsible for fimbria was detected in 19 (76%) bacterial isolates. Further, these isolates were subjected to flagella, protease, and hemolysin, and it was revealed that flg 11 (44%), 13 (52%) protease coding zapA, and hmA gene coding hemolysin were amplified in 2 (8%) Proteus isolates. Probiotic bacteria isolated from soil samples were probed for antagonistic activity against Proteus species. The probiotic bacteria were identified as Lactobacillus plantarum, Bacillus subtilis, and B. licheniformis. Due to their strong growth inhibitory effects against Proteus, it is crucial to characterize further the metabolites that have shown suppressive results against Proteus.Conclusions:
Findings from the current study will provide new avenues for drug development and also help clinicians manage resistant pathogens in healthcare settings. Probiotic applications for infection control can be useful in treating resistant pathogens. Further purification and characterization of metabolites will provide alternative options for managing resistance issues in microbes.Keywords
1. Background
The genus Proteus is a Gram-negative bacterium that exhibits unique characteristics of swarming. Proteus species are classified as important pathogens of urinary tract infections (UTI) in immunocompromised and hospitalized patients. Among Proteus species, Proteus mirabilis and P. vulgaris are frequently involved in UTIs (1). Individuals suffering from UTI caused by Proteus species often develop bacteremia, cystitis, kidney and bladder stones, and catheter obstruction (2). Infections have a direct correlation with Proteus virulence factors such as fimbria,flagellum, capsules, enzymes (urease, proteases, and deaminase), toxins (hemolysins, Proteus toxic agglutinin (Pta) and endotoxins (3). Hemolysin HpmA has a strong hemolytic activity, and it can damage the soft tissue of the host (4). Proteus species produce extracellular proteolytic enzymes, which cleave antibodies (IgA and IgG) and non-antibody proteins such as gelatin, secretory components, casein, and bovine serum albumin (5).
Bacterial biofilm is an extracellular matrix consisting of DNA, proteins, and carbohydrates, constructed by bacterial communities to form complex structures that adhere to living or non-living surfaces and which restrain the actions of antimicrobial substances (6). Swarming, another virulence factor produced by Proteus species, is characterized by multicellular rafts of elongated, hyper-flagellated swarmer cells that form and move rapidly in concert over solid surfaces. It has been suggested that swarming is important in the pathogenesis of UTIs (7). Probiotic bacteria such as Lactococcus and Lactobacillus are widely distributed in the environment and can prevent the growth of pathogenic microorganisms by producing particular antimicrobial metabolites (8). Due to the emergence of resistance to multiple antimicrobial agents, particularly in Proteus species, has posed a major threat to public health and confined the available drugs option (9). In Pakistan, drug resistance is in a terrible state as numerous studies have revealed up-rise resistance cases in Proteus species. Proteus bacteria cause opportunistic infections and are also the main source of nosocomial infections; there should be proper strategies to restrict their dissemination.
2. Objectives
Due to limited antibiotic options, emphasis should be on exploring new alternatives which can be used to restrain such pathogens. As probiotics have a strong potential to inhibit the growth of pathogenic bacteria in the host using their antagonistic mechanism (10), the current study was designed to screen potential probiotic bacteria to encounter antibiotic-resistant and virulent Proteus species.
3. Methods
3.1. Culturing of Drug-Resistant Proteus Isolates
The resistant Proteus species (n = 25) were acquired from the Department of Microbiology, Kohat University of Science and Technology, Kohat, Khyber Pakhtunkhwa. Glycerol stock (50%) was refreshed on MacConkey agar and was incubated at 37°C overnight. In order to get pure colonies, the same subculture was on plain growth media, and some basic identification tests were performed to reconfirm the mentioned isolates.
3.2. Phenotypic Study of Virulence Factors
3.2.1. Biofilm Formation
Proteus bacteria were screened quantitatively for biofilm detection using tube assay. A test tube carrying brain heart infusion (BHI) media supplemented with glucose was inoculated with test bacteria and was incubated for 24 hours. Further, after 24 hours, these test tubes were washed with phosphate buffer saline (PBS) and waited to still dried. Then the tubes were stained with crystal violet for 8 - 10 minutes and kept in an inverted position to get dried. In test tubes, the visible line was interpreted as a positive for biofilm potential, and the optical density (OD) was measured at a wavelength of 600 nm (11).
3.2.2. Extracellular Protease Activity
These resistant bacteria were filtered for protease activity on media supplemented with skim milk and NaCl. Proteus fresh inoculums were used for culturing on skim milk agar plates and were incubated at 35°C for 48 hours (12).
3.2.3. Swarming Potential of Proteus
In order to find their swarming potential, Proteus bacteria were cultured on nutrient agar media overnight, and the temperature was maintained at 37ºC. Jansen et al. showed that different fagellated cells were determined with the help of their pattern obtained through swarming (13).
3.2.4. Hemolysin Activity
For detection of hemolysin activity, Agar plates were prepared by adding sheep blood, and the bacterial lawn was prepared by inoculating the plates and were confirmed after incubation for 24 hours for the presence of hemolysis (α, β or γ) or no hemolysis (14).
3.3. Molecular Characterization of the Virulence Genes
Whole bacterial DNA was extracted by the standard Phenol Chloroform Extraction method as described earlier (15) for different genes like zapA, flg, hmpA, mrp, and rsbA under specific PCR conditions.
3.4. PCR Condition for Amplification of Virulence Genes
All genes were amplified through conventional PCR. Each reaction comprised of 12.5 µL Master Mix (10 mM Tris HCl, 50 mM KCl, 1.5 mM MgCl2, 0.5 mM DDT, 5% glycerol, 0.001% Gelatin, 0.05% NP-40, 0.05 mM tween-20, 0.2 mM dNTPs and 25units/µl Taq DNA Polymerase) provided by Gene Link, 0.5 µL of both forward and reverse primers, 1 µL of genomic DNA, and rest of PCR water were added to make the volume 25 µL each. PCR conditions and primer details are provided, as shown in Table 1. Further, the PCR product was run on 1.5% agarose gel, and the product band pattern was analyzed on a gel doc system (FAS-Digi PRO).
Virulence Genes Primers Sequence and Their Tm Value 417
Genes | Sequences 5´-3´ | Amplicon Size (bp) | Annealing Temp. (°C) | Reference |
---|---|---|---|---|
rsbA | (F)5´-TTGAAGGACGCGATCAGACC-3´ | 467 | 59 | (16) |
(R)5´-ACTCTGCTGTCCTGTGGGTA-3´ | ||||
mrp | (F)5´-ATTTCAGGAAACAAAAGATG-3´ | 565 | 40 | (17) |
(R)5´-TTCTTACTGATAAGACATTG-3´ | ||||
flg | (F)5´-AGGATAAATGGCCACATTG-3´ | 417 | 50 | (18) |
(R)5´-CGGCATTGTTAATCGCTTTT-3´ | ||||
zapA | (F)5´-ACCGCAGGAAAACATATAGCCC-3´ | 540 | 61 | (19) |
(R)5´-GCGACTATCTTCCGCATAATCA-3´ | ||||
hmpA | (F)5´-GTTGAGGGGCGTTATCAAGAGTC3´ | 709 | 55 | (20) |
(R)5´-GATAACTGTTTTGCCCTTTTGTGC3´ |
3.5. Screening of Probiotic Bacteria Carrying Antagonistic Activity
Soil samples were collected from different sites in Kohat for screening of probiotic bacteria from May 2019 to June 2019. The pure culture was obtained by serial dilution, and the pure plate method by using nutrient agar. The good diffusion method was used to confirm antagonistic activity toward resistant Proteus isolates. Proteus bacterial lawns were prepared on Muller Hinton agar (MHA) Petri plates. Proper wells were prepared, filled with cell-free filtrate, and plates were incubated at 37°C for overnight (16) along with Escherichia coli (ATCC 25922) used as a reference strain (control). The strong antagonistic activity against Proteus species was selected for further study.
3.6. Morphological Confirmation of Probiotic Bacteria
Isolates carrying strong antagonistic activities against Proteus bacteria were subjected to a Gram staining procedure for further characterization.
3.7. Molecular Characterization of Probiotic Bacteria
For species-level characterization, 16srRNA, universal primers (F) 5'GGAGGCAGCAGTAGGGAATA-3' and (R) 5´-TGACGGGCGGTGAGTACAAG-3' were used for molecular characterization of probiotic bacteria (21). PCR reactions were performed where 94°C was set as initial denaturation for 5 min, followed by 35 cycles, denaturation at 94°C for 1 minute, annealing at 50°C for 40 seconds, polymerization at 72°C for 1 minute 30 seconds, and final extension was carried out at 72°C for 5 minutes. Amplified PCR products were confirmed on a 1.5% agarose gel in TBE buffer stained with 3 µL of ethidium bromide and ran for 30 minutes at 100 voltage and 300 milliampere current. Later, the gel was visualized under a UV transilluminator, and images were saved using the FAS-Digi PRO Gel Doc system.
3.8. Sequencing and Phylogenetic Analysis
Amplified PCR products, after purification, sequencing reactions were performed using a forward primer. For sequence analysis, reactions were carried out at Macrogen (Seoul), South Korea. Sequencing data were deduced using Bioedit software. Phylogenetic analysis was accomplished using Mega X software.
3.9. Statistical Analysis
The data was analyzed through statistical software, SPSS version 16, by using the One-way ANOVA test. The P-value < 0.05 was considered statistically significant.
4. Results
4.1. Culturing of Proteus Species
After inoculating Proteus bacteria on MacConkey differential media and nutrient agar, colonies with a yellowish appearance and bad fishy smell will be scrutinized as Proteus species. After getting pure colonies, samples were deployed for virulence factors investigation.
4.2. Phenotypically Detection of Virulence Factors of Proteus Species
Biofilm formation was evaluated through the tubes method for all isolates, and their optical density (OD) was measured at 570 nm. There were 8 (32%) strong biofilm producers, 10 (40%) moderate biofilm activity, while 4 (16%) were reported as weak biofilm producers, as shown in Figure 1A. In tested isolates only, there were only 3 (12%) isolates that had shown no biofilm activity. Out of 25 isolates, the highest and lowest OD was observed as 0.982 and 0.240, respectively. The protease activity was evaluated on agar plates, and clear zones were observed around Proteus colonies, as depicted in Figure 1B. The total (n = 14) isolates revealed positive protease activity out of (n = 25) isolates. Further, these isolates were checked for swarming activity. Swarming activity was recorded for all isolates by showing differentiated ring-like growth, as presented in Figure 1C. Hemolysis is one of the key factors responsible for pathogenesis in infected individuals. Proteus isolates were examined on the blood agar plate for α, β, and γ hemolysis, as indicated in Figure 1D. In all 15 isolates, only six isolates showed positive hemolytic activity.
Biofilm formation (A) protease activity (B) swarming activity (C) and hemolysin activity (D) of Proteus species.
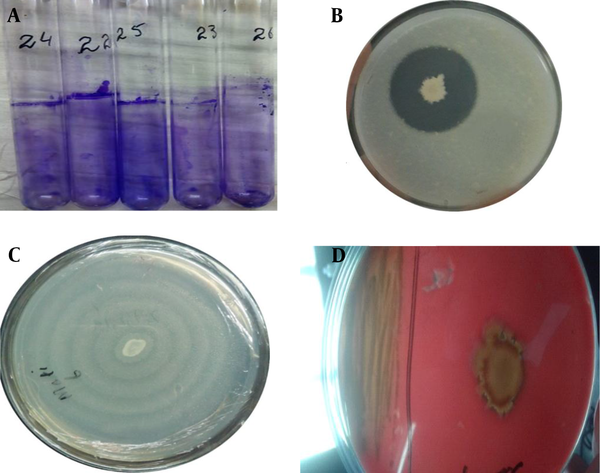
4.3. Molecular Depiction of Virulent Factors of Proteus and Probiotic Bacteria
Further confirmation of virulence factors was followed by the amplification of the genes that are responsible for virulence factor expression. The gene rsbA was amplified in 17 (68%) Proteus strains, mrp in 19 (76%), flg in 11 (44%), zapA in 13 (52%), and hmpA amplified in only 2 (8%) Proteus isolates as shown in Figures 2 and 3.
Genotypic comparison among virulence genes among Proteus isolates.
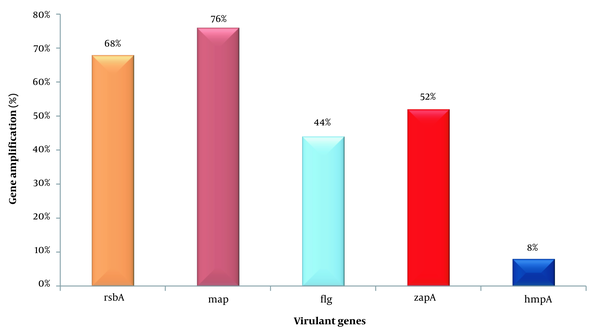
PCR product of (A) rsbA gene, (B) mrp gene, (C) flg gene, (D) zapA gene, (E) hmpA gene, and (F) universal primer gene amplified at different base pairs, i.e., 467 bp, 565 bp, 417 bp, 540 bp, 950 bp respectively using 1 kb marker.
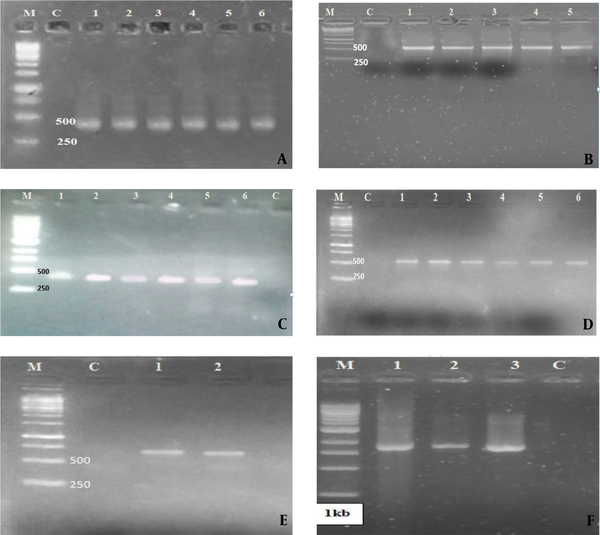
4.4. Soil Samples and Their Antagonistic Effects Against Resistant Strains
Total (n = 34) samples were collected from different sources of plant roots and near areas such as the Botanical Garden, University Cafe extreme, and near the areas of hilly plants screening for antagonistic activity against resistant strains. In total, three bacterial samples were active against resistant strains with the formation of a strong inhibitory zone (Figure 4).
Antagonistic activity of Probiotic bacteria against Proteus species, Positive Sample (PS), Negative Control (NC), and antibiotic were used as Positive Control (PC).
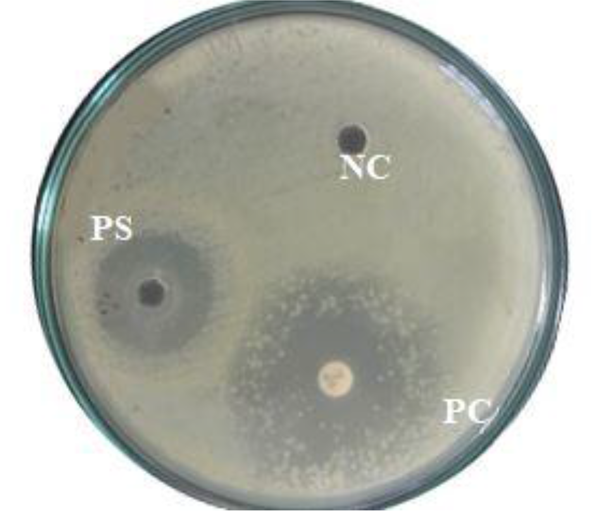
4.5. Morphological Features of Soil Probiotic Bacteria Against Resistant Stains
Further morphological features of positive samples with strong antagonistic activity were checked using Gram staining, and biochemical tests like oxidase, catalase, urease, coagulase, hemolytic activity, motility, and spore formation are shown in Table 2.
Morphological Features of Soil Probiotic Bacteria Against Resistant Stains
Morphological Features of Soil Samples, Species Name | Gramreaction | Motility | Spore | Catalase | Oxidase | Urease | Coagulase | Hemolysis |
---|---|---|---|---|---|---|---|---|
L. plantarum | + | - | - | - | - | - | - | Alpha |
B. subtilis | + | + | + | + | Variable | - | - | Beta |
B. licheniformis | + | + | + | + | Variable | Variable | _ | Gamma |
4.6. Molecular Study and Phylogenetic Analysis of Probiotic Bacteria
Sequence analysis was done for the PCR products of probiotic bacteria. Three different strains of probiotic bacteria were found Lactobacillus plantarum, Bacillus subtilis, and B. licheniformis, which show strong antagonistic activity against Proteus species (Figure 5).
(A), (B), and (C) Phylogenetic tree showing the position of the isolated strains constructed using MEGA X. The nucleotide sequence used for finding the evolutionary relationship was ~ 950 bp. The likelihood method was applied to find evolutionary distances. Each respective tree analysis has 13 nucleotide sequences. All unclear points were deleted for each sequence pair (pairwise removal).
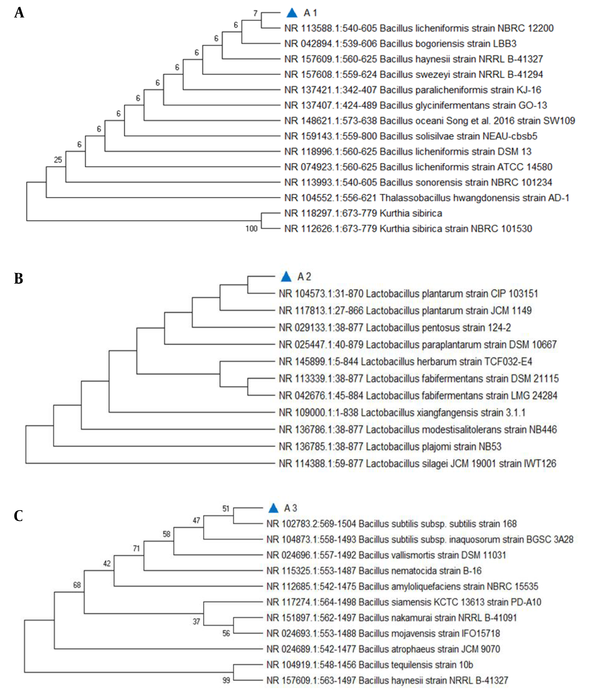
5. Discussion
The genus Proteus has a Gram-negative cell wall composed of heterotrophic, proteolytic rods. Along with other common pathogens, Proteus is regarded as one of the most prevalent pathogens associated with hospital-borne infections. Latterly, the P. myxofaciens genus was moved from Proteus to the Cosenzaea genus (22). Proteus exhibits yellowish, mucoid, convex, and entire margins colonies on MacConkey agar. Swarming and other virulence factors play a main role in their pathogenicity in the infected host. Proteus spp. was detected in many infections, particularly with UTI. Since Proteus spp. is an opportunistic pathogen, even healthy humans can be asymptomatic carriers of the pathogen (19). In the present study, fimbriae (mrp gene) 76% was the predominant virulence factor, followed by Swarming (rsbA gene) 68%, protease (zapA gene) 52%, flagella (flg gene) 44% and hemolysin (hmpA gene) 8% in Proteus spp.
Mrp protein is associated with fimbriae and plays an important role in adhesins at the fimbrial tips (1). In the current findings, mrp expression was observed at 19 (76%), which is in line with previous reports (23). Likewise, current results were higher as compared to earlier reports about Proteus (16, 17). This fluctuation in expression may be due to geographic location and environmental variations. Swarming (rsbA gene) was positive in 68% of Proteus spp, which is in line with already conducted studies of Rather (24). This finding is in contrast to one study in Korea by Pathirana et al. that shows the highest no of the rsbA gene (50%) (16) while higher expression was seen in overall clinical isolates (23). In our study, there were 14 (56%) positive for protease enzyme, which is in accordance with earlier reported cases of Proteus (16). All Proteus isolates were positive for protease in comparison to our findings (25). High expression of flaA gene was detected in (26, 27) and this difference may be due to different habitats or dietary requirements.
The present study was conducted on general Proteus isolates, in which different parameters like the ability of extracellular hemolysin etc., were checked. In a current investigation (n = 6, 24%), isolates were positive for hemolysin on blood agar, which had lower hemolytic activity than already reported cases. One study demonstrated high hemolysin activity for P. mirabilis isolates, which might be crucial, especially in providing iron as a cofactor for bacteria and helping with renal cell destruction (28). Proteus mirabilis has shown high hemolytic activity in the blood (28, 29), which is not agreeable to our findings as in our research, the activity is as low as 24%, which might be the cause of low sample size and of general Proteus isolates. In Brazil, Sanches et al. showed 32% positive results for the hmpA gene in Proteus isolates (30). This finding is similar our results where (n = 24%) isolates were positive for hemolysin. According to (20), higher expression of the hmpA gene was also observed.
Lactobacillus plantarum can inhibit the growth of some Enterobacteriaceae, including Proteus species (31). Probiotics that were isolated from different sources revealed strong antibacterial activities (32). In current findings, L. plantarum also has strong antagonistic activity against Proteus species. Similarly, B. licheniformis has the potential to inhibit the growth of both Gram-positive and Gram-negative bacteria, as reported by Kim et al. (33). It has been revealed that B. licheniformis has strong activity against Micrococcus luteus, considering it as a potential probiotic which supports our findings (34). In the current study, P = 0.0138 < 0.05 showed that the virulence genes were significantly varying in different bacterial isolates (Figure 2). Similarly, the P = 0.0295 < 0.05 (Figure 6) shows virulence factors significantly different from each other phenotypically, which was different from another study (35).
Graphical representation of phenotypically detected virulence factors of Proteus bacteria.
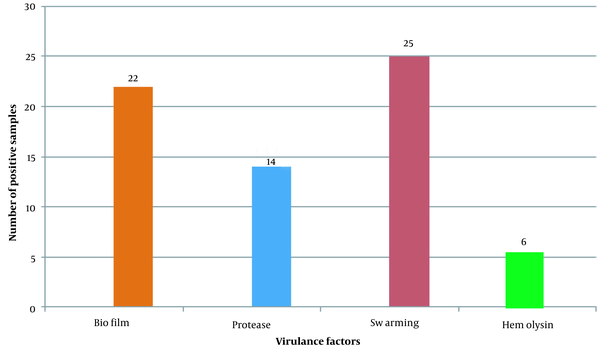
The probiotic bacteria, B. subtilis, may secrete some substances that have strong antimicrobial activity against B. cereus, Listeria monocytogenes, M. luteus, and Staphylococcus aureus, as revealed by earlier reports (36). In the current study, we found different strains of probiotic bacteria that produce inhibitory bacteriocin-like substances for the inhibition of pathogenic bacteria that may be used safely (37, 38). The physio-chemical composition of the bacteria-producing substances like nisin, lacidin, bacilli, subtilin, and pediocin showed high antimicrobial activity, especially B. subtilis (39). That particular probiotic bacteria have also shown strong activity against L. monocytogenes, M. luteus, and B. cereus, reported by Khochamit et al. (40). Similar results have been shown by B. subtilis with strong antagonistic activity against resistant Proteus species.
5.1. Conclusions
In recent findings, resistant Proteus bacteria were scanned for their virulence profile. It has been established that bacteria with biofilm potential have adopted hostile behavior toward antibiotics, a key strategy to protect and shelter any potential hazards. Due to their protease enzymes, they have the capability to degrade proteins needed for different physiological processes. Moreover, most of these isolates expressed virulence factors like hemolysin, swarming, protease, fimbriae, and flagella. Furthermore, these factors are reconfirmed by amplifying their gene virulence factors such as zapA, flg, hmpA, mrp, and rsbA. Probiotics being isolated from soil have a strong antagonist approach toward virulent Proteus species. Lactobacillus plantarum, B. subtilis, and B. licheniformis were identified as potential probiotics that can be helpful in managing resistant pathogens like Proteus. Characterization of anti-bacterial metabolites will be helpful for further studies. After exposure to probiotic stress, expression patterns of virulence factors can help design new drug targets. These findings will provide new avenues for drug development and also will help clinicians with the management of such pathogens in healthcare centers.
References
-
1.
Coker C, Poore CA, Li X, Mobley HL. Pathogenesis of Proteus mirabilis urinary tract infection. Microbes Infect. 2000;2(12):1497-505. [PubMed ID: 11099936]. https://doi.org/10.1016/s1286-4579(00)01304-6.
-
2.
Himpsl SD, Lockatell CV, Hebel JR, Johnson DE, Mobley HLT. Identification of virulence determinants in uropathogenic Proteus mirabilis using signature-tagged mutagenesis. J Med Microbiol. 2008;57(Pt 9):1068-78. [PubMed ID: 18719175]. https://doi.org/10.1099/jmm.0.2008/002071-0.
-
3.
Alamuri P, Mobley HL. A novel autotransporter of uropathogenic Proteus mirabilis is both a cytotoxin and an agglutinin. Mol Microbiol. 2008;68(4):997-1017. [PubMed ID: 18430084]. https://doi.org/10.1111/j.1365-2958.2008.06199.x.
-
4.
Hamilton AL, Kamm MA, Ng SC, Morrison M. Proteus spp. as putative gastrointestinal pathogens. Clin Microbiol Rev. 2018;31(3). [PubMed ID: 29899011]. [PubMed Central ID: PMC6056842]. https://doi.org/10.1128/CMR.00085-17.
-
5.
Schmidtchen A, Frick IM, Andersson E, Tapper H, Bjorck L. Proteinases of common pathogenic bacteria degrade and inactivate the antibacterial peptide LL-37. Mol Microbiol. 2002;46(1):157-68. [PubMed ID: 12366839]. https://doi.org/10.1046/j.1365-2958.2002.03146.x.
-
6.
De Fanti S. Evolutionary genetics of lactase persistence in Eurasian human populations. Alma Mater Studiorum University of Bologna; 2014.
-
7.
Estifanos H. Isolation and identification of probiotic lactic acid bacteria from curd and in vitro evaluation of its growth inhibition activities against pathogenic bacteria. Afr J Microbiol Res. 2014;8(13):1419-25. https://doi.org/10.5897/ajmr2014.6639.
-
8.
Jones BV, Young R, Mahenthiralingam E, Stickler DJ. Ultrastructure of Proteus mirabilis swarmer cell rafts and role of swarming in catheter-associated urinary tract infection. Infect Immun. 2004;72(7):3941-50. [PubMed ID: 15213138]. [PubMed Central ID: PMC427392]. https://doi.org/10.1128/IAI.72.7.3941-3950.2004.
-
9.
Fazeli MR, Vaghari E, Jamalifar H, Samadi N. Antimicrobial activity of Lactobacillus plantarum strains isolated from fermented olives origin. Q Sci Res J Med Plants. 2009;8(31):14-8.
-
10.
Cohen-Nahum K, Saidel-Odes L, Riesenberg K, Schlaeffer F, Borer A. Urinary tract infections caused by multi-drug resistant Proteus mirabilis: Risk factors and clinical outcomes. Infection. 2010;38(1):41-6. [PubMed ID: 19998053]. https://doi.org/10.1007/s15010-009-8460-5.
-
11.
Hassan M, Sanchez B, Yu J. Financial development and economic growth: New evidence from panel data. Q Rev Econ Fin. 2011;51(1):88-104. https://doi.org/10.1016/j.qref.2010.09.001.
-
12.
Ali N, Ullah N, Qasim M, Rahman H, Khan SN, Sadiq A, et al. Molecular characterization and growth optimization of halo-tolerant protease producing Bacillus Subtilis Strain BLK-1.5 isolated from salt mines of Karak, Pakistan. Extremophiles. 2016;20(4):395-402. [PubMed ID: 27114252]. https://doi.org/10.1007/s00792-016-0830-1.
-
13.
Jansen AM, Lockatell CV, Johnson DE, Mobley HL. Visualization of Proteus mirabilis morphotypes in the urinary tract: the elongated swarmer cell is rarely observed in ascending urinary tract infection. Infect Immun. 2003;71(6):3607-13. [PubMed ID: 12761147]. [PubMed Central ID: PMC155743]. https://doi.org/10.1128/IAI.71.6.3607-3613.2003.
-
14.
Hasan R, Acharjee M, Noor R. Prevalence of vancomycin resistant Staphylococcus aureus (VRSA) in methicillin resistant S. aureus (MRSA) strains isolated from burn wound infections. Ci Ji Yi Xue Za Zhi. 2016;28(2):49-53. [PubMed ID: 28757721]. [PubMed Central ID: PMC5442891]. https://doi.org/10.1016/j.tcmj.2016.03.002.
-
15.
Golani D, Sonin O, Snovsky G, David L, Tadmor-Levi R. The occurrence of the peacock bass (Cichla kelberi Kullander and Ferreira 2006) in Lake Kinneret (Sea of Galilee), Israel. BioInvasions Records. 2019;8(3):706-11. https://doi.org/10.3391/bir.2019.8.3.28.
-
16.
Pathirana H, De Silva BCJ, Wimalasena S, Hossain S, Heo GJ. Comparison of virulence genes in Proteus species isolated from human and pet turtle. Iran J Vet Res. 2018;19(1):48-52. [PubMed ID: 29805463]. [PubMed Central ID: PMC5960773].
-
17.
Bahrani FK, Mobley HL. Proteus mirabilis MR/P fimbrial operon: genetic organization, nucleotide sequence, and conditions for expression. J Bacteriol. 1994;176(11):3412-9. [PubMed ID: 7910820]. [PubMed Central ID: PMC205519]. https://doi.org/10.1128/jb.176.11.3412-3419.1994.
-
18.
Ram P, Rao V, Rao S, Subramanyam KV, Srinivas K. Prevalence and virulence gene profiles of Proteus mirabilis isolated from animal, human and water samples in Krishna District, Andhra Pradesh, India. Pharma Innov J. 2019;8(6):19-23.
-
19.
Sun Y, Wen S, Zhao L, Xia Q, Pan Y, Liu H, et al. Association among biofilm formation, virulence gene expression, and antibiotic resistance in Proteus mirabilis isolates from diarrhetic animals in Northeast China. BMC Vet Res. 2020;16(1):1-10. [PubMed ID: 32503535]. [PubMed Central ID: PMC7275385]. https://doi.org/10.1186/s12917-020-02372-w.
-
20.
Cestari SE, Ludovico MS, Martins FH, da Rocha SP, Elias WP, Pelayo JS. Molecular detection of HpmA and HlyA hemolysin of uropathogenic Proteus mirabilis. Curr Microbiol. 2013;67(6):703-7. [PubMed ID: 23884594]. https://doi.org/10.1007/s00284-013-0423-5.
-
21.
Nazish A, Khattak B, Ali Khan T, Ahmad I, Ullah R; Fozia, et al. Antinematode activity of Abomasum bacterial culture filtrates against Haemonchus contortus in small ruminants. Animals (Basel). 2021;11(6). [PubMed ID: 34205748]. [PubMed Central ID: PMC8235536]. https://doi.org/10.3390/ani11061843.
-
22.
Giammanco GM, Grimont PAD, Grimont F, Lefevre M, Giammanco G, Pignato S. Phylogenetic analysis of the genera Proteus, Morganella and Providencia by comparison of rpoB gene sequences of type and clinical strains suggests the reclassification of Proteus myxofaciens in a new genus, Cosenzaea gen. nov., as Cosenzaea myxofaciens comb. nov. Int J Syst Evol Microbiol. 2011;61(Pt 7):1638-44. [PubMed ID: 20709916]. https://doi.org/10.1099/ijs.0.021964-0.
-
23.
Hussein EI, Al-Batayneh K, Masadeh MM, Dahadhah FW, Al Zoubi MS, Aljabali AA, et al. Assessment of pathogenic potential, virulent genes profile, and antibiotic susceptibility of proteus mirabilis from urinary tract infection. Int J Microbiol. 2020;2020:1231807. [PubMed ID: 32089693]. [PubMed Central ID: PMC7029293]. https://doi.org/10.1155/2020/1231807.
-
24.
Rather PN. Swarmer cell differentiation in Proteus mirabilis. Environ Microbiol. 2005;7(8):1065-73. [PubMed ID: 16011745]. https://doi.org/10.1111/j.1462-2920.2005.00806.x.
-
25.
Li X, Mobley HL. Vaccines for Proteus mirabilis in urinary tract infection. Int J Antimicrob Agents. 2002;19(6):461-5. [PubMed ID: 12135833]. https://doi.org/10.1016/s0924-8579(02)00102-4.
-
26.
Al-Mayahi FS. Phenotypic and Molecular detection of Virulence factors in Proteus mirabilis isolated from different clinical sources. Bas J Vet Res. 2017;16(1):369-88.
-
27.
Ali HH, Yousif MG. Detection of some virulence factors genes of Proteus mirablis that isolated from urinary tract infection. IJAR. 2015;3(1):156-63.
-
28.
Mishra M, Thakar YS, Pathak AA. Haemagglutination, haemolysin production and serum resistance of proteus and related species isolated from clinical sources. Indian J Med Microbiol. 2001;19(2):5-11. [PubMed ID: 17664798].
-
29.
Mobley HL, Chippendale GR. Hemagglutinin, urease, and hemolysin production by Proteus mirabilis from clinical sources. J Infect Dis. 1990;161(3):525-30. [PubMed ID: 2179424]. https://doi.org/10.1093/infdis/161.3.525.
-
30.
Sanches MS, Baptista AAS, de Souza M, Menck-Costa MF, Koga VL, Kobayashi RKT, et al. Genotypic and phenotypic profiles of virulence factors and antimicrobial resistance of Proteus mirabilis isolated from chicken carcasses: potential zoonotic risk. Braz J Microbiol. 2019;50(3):685-94. [PubMed ID: 31049879]. [PubMed Central ID: PMC6863274]. https://doi.org/10.1007/s42770-019-00086-2.
-
31.
Oldak A, Zielinska D, Rzepkowska A, Kolozyn-Krajewska D. Comparison of antibacterial activity of lactobacillus plantarum strains isolated from two different kinds of regional cheeses from poland: Oscypek and korycinski cheese. Biomed Res Int. 2017;2017:6820369. [PubMed ID: 28626762]. [PubMed Central ID: PMC5463104]. https://doi.org/10.1155/2017/6820369.
-
32.
Rana D, Gupta KK. Evaluation of antagonistic activities of Bacillus spp. against certain bacteria of medical importance. Arch Agriculture Environ Sci. 2017;2(4):353-6. https://doi.org/10.26832/24566632.2017.020419.
-
33.
Kim Y, Cho JY, Kuk JH, Moon JH, Cho JI, Kim YC, et al. Identification and antimicrobial activity of phenylacetic acid produced by Bacillus licheniformis isolated from fermented soybean, Chungkook-Jang. Curr Microbiol. 2004;48(4):312-7. [PubMed ID: 15057459]. https://doi.org/10.1007/s00284-003-4193-3.
-
34.
Shobharani P, Padmaja RJ, Halami PM. Diversity in the antibacterial potential of probiotic cultures Bacillus licheniformis MCC2514 and Bacillus licheniformis MCC2512. Res Microbiol. 2015;166(6):546-54. [PubMed ID: 26100933]. https://doi.org/10.1016/j.resmic.2015.06.003.
-
35.
Danilo de Oliveira W, Lopes Barboza MG, Faustino G, Yamanaka Inagaki WT, Sanches MS, Takayama Kobayashi RK, et al. Virulence, resistance and clonality of Proteus mirabilis isolated from patients with community-acquired urinary tract infection (CA-UTI) in Brazil. Microb Pathog. 2021;152:104642. [PubMed ID: 33246088]. [PubMed Central ID: PMC7938216]. https://doi.org/10.1016/j.micpath.2020.104642.
-
36.
Olga E, Nina G, Irina M, Mariya D, Tatiana E, Eugene R, et al. Antimicrobial properties of the probiotic strain bacillus subtilis 534. Int Arch Med Microbiol. 2019;2(1). https://doi.org/10.23937/iamm-2017/1710008.
-
37.
Barboza-Corona JE, Vazquez-Acosta H, Bideshi DK, Salcedo-Hernandez R. Bacteriocin-like inhibitor substances produced by Mexican strains of Bacillus thuringiensis. Arch Microbiol. 2007;187(2):117-26. [PubMed ID: 17031616]. https://doi.org/10.1007/s00203-006-0178-5.
-
38.
Hammami I, Jaouadi B, Bacha AB, Rebai A, Bejar S, Nesme X, et al. Bacillus subtilis bacteriocin Bac 14B with a broad inhibitory spectrum: Purification, amino acid sequence analysis, and physicochemical characterization. Biotechnol Bioprocess Eng. 2012;17(1):41-9. https://doi.org/10.1007/s12257-010-0401-8.
-
39.
Joseph B, Dhas B, Hena V, Raj J. Bacteriocin from Bacillus subtilis as a novel drug against diabetic foot ulcer bacterial pathogens. Asian Pac J Trop Biomed. 2013;3(12):942-6. [PubMed ID: 24093784]. [PubMed Central ID: PMC3805101]. https://doi.org/10.1016/S2221-1691(13)60183-5.
-
40.
Khochamit N, Siripornadulsil S, Sukon P, Siripornadulsil W. Antibacterial activity and genotypic-phenotypic characteristics of bacteriocin-producing Bacillus subtilis KKU213: potential as a probiotic strain. Microbiol Res. 2015;170:36-50. [PubMed ID: 25440998]. https://doi.org/10.1016/j.micres.2014.09.004.