Abstract
Background:
Multidrug-resistant (MDR) Acinetobacter baumannii is one of the most common nosocomial pathogens. Antimicrobial peptides (AMPs) have been introduced as a viable alternative to antibiotics in the treatment of MDR pathogens.Objectives:
This study was designed to assess the in vitro pharmacokinetics of the combination of two potent AMPs, LL-37 and oncorhyncin II, against A. baumannii (ATCC19606).Methods:
The synthesized genes of oncorhyncin II and LL-37 were introduced into Escherichia coli BL21 as the expression host. The minimum inhibitory concentration (MIC), time-kills, and growth kinetics of these peptides were used to evaluate their antimicrobial efficiencies against A. baumannii (ATCC19606).Results:
LL-37 and oncorhyncin II recombinant peptides showed MIC of 30.6 and 95.87 µg/mL against A. baumannii, respectively. Additive action was confirmed by combining the generated AMPs at the checkerboard approach. The combination of LL-37 and oncorhyncin II at 2 × MIC resulted in a rapid drop in log10 CFU/mL of A. baumannii in the time-kill and growth kinetic findings studies.Conclusions:
The combination of the produced LL-37 and oncorhyncin II synergizes the bioactivity of the individual peptides. Therefore, these peptides or their combinations might function as novel antibiotics and be used to develop and produce new antimicrobial drugs for the treatment of infections caused by A. baumannii.Keywords
Antimicrobial Peptide (AMP) Recombinant Protein LL-37 Oncorhyncin II Acinetobacter baumannii
1. Background
Acinetobacter baumannii, Gram-negative pathogenic bacteria, is presently one of the most common causes of nosocomial infections in hospitals (1). Bloodstream infections, soft tissue infections, skin infections, ventilator-associated pneumonia, and catheter-associated urinary tract infections are all examples of infections that might occur by A. baumannii (2). In recent years, the overuse of antibiotics, especially polymyxin E (colistin), has led to the development of multidrug-resistant (MDR) A. baumannii. Polymyxin E (colistin) is a cationic peptide antibiotic, the extensive use of which as the last line of treatment in MDR infections has created colistin-resistant strains. These bacteria can develop resistance to the antibiotic polymyxin E through complete loss of lipopolysaccharide (LPS) expression (3-6). In recent years, a rising tendency in A. baumannii MDR infections and a rapidly diminishing number of available treatment choices have compelled researchers to design and develop new antimicrobials.
Antimicrobial peptides (AMPs) are a novel class of chemicals that have the potential to be used as an alternative to conventional antibiotics. Generally, AMPs are amphipathic molecules and a diverse set of short oligopeptides that protect creatures from a wide range of pathogens, such as viruses, bacteria, and parasites (7, 8). However, AMPs, by using a wide spectrum of different mechanisms, such as cell wall destruction or protein/deoxyribonucleic acid suppression of apoptosis promotion, provoke microbial cell death (9-12). Multiple AMPs have been studied in the treatment of bacterial infections and have been found to be potentially helpful. As a result, AMPs, alone or in combination with known antibiotics, might have a therapeutic effect (13, 14).
2. Objectives
This study investigated the in vitro pharmacokinetics of the combination of LL-37 and oncorhyncin II against A. baumannii.
3. Methods
3.1. Expression and Purification of Recombinants LL-37 and Oncorhyncin II
Experiments were conducted against A. baumannii ATCC strains 19606 obtained from BEI resources (Manassas, VA, USA). Polymyxin E was purchased from Sigma-Aldrich (St. Louis, MO, USA). The AMP LL-37 (LLGDFFRKSKEKIGKEFKRIVQRIKDFLRNLVPRTES) and oncorhyncin II (KAVAAKKSPKKAKKPATPKKAAKSPKKVKKPAAAAKKAAKSPKKATKAAKPKAAKKAAKAKKAAPKI) were acquired from Cambridge Biomatik Company (Cambridge, Canada). Then, the plasmid pET32a (Novagen, India) was prepared as expression vector (15, 16). Subsequently, Escherichia coli BL21 (DE3), the common expression host, were applied to proceed recombinant protein expression. To express the target genes, the pET32a-LL-37 and pET32a-oncorhyncin II constructs were transplanted individually to competent bacteria E. coli BL21 (DE3). Competent E. coli BL21 (DE3) cells were effectively isolated after being prepared according to the usual technique (17).
In this method, LL-37 and oncorhyncin II recombinant expressions were induced using E. coli BL21 (DE3) as the expression host, with isopropyl β-D-galactosidase (IPTG) (1 mM) combined with Nutrient Broth media (Nutrient Broth; Merck, Germany) (1.5 X). The cells were extracted after a 4-hour incubation period by centrifugation at 5000 rpm for 20 minutes. Transferring the resultant protein to the (12%) sodium dodecyl sulfate-polyacrylamide gel electrophoresis (SDS-PAGE) proved the induction results (15, 18). Purification was determined using Ni-NTA agarose resin affinity chromatography (Qiagen, Alameda, CA, USA) under denaturing conditions with 8 M urea (18). The quantity of the recombinant proteins was measured by optical density (OD) at 280 nm by spectrophotometry. The quality of the recombinant proteins was analyzed by SDS-PAGE (12% SDS gel) (18, 19).
3.2. Refolding Optimization of Recombinants LL-37 and Oncorhyncin II
Phosphate-buffered saline (PBS) buffers containing different amino acids (arginine 0.1 M + proline 0.1 M) with optimized condition (pH = 7) and PBS buffers containing amino acids (arginine 0.1 M + proline 0.1 M) with pH = 8.5 for LL-37 and oncorhyncin II, respectively, were used for the refolding process and elimination of urea. It should be emphasized that urea prevents proteins from folding actively. The dialysis process was performed at 4°C for 24 hours. The PBS buffer was changed every 2 hours to improve dialysis efficiency. Finally, the dialyzed protein was kept at 4°C for future testing (15, 20). Due to refolded recombinant proteins’ low concentrations in the dialyzer tubes, a 10 kDa pore-size Amicon centrifugal filter (Merck Millipore, Darmstadt, Germany) was applied to increase their concentrations (21).
3.3. Antimicrobial Activities of LL-37 and Oncorhyncin II
The minimum inhibitory concentration (MIC) of LL-37 and oncorhyncin II was determined by the broth microdilution technique based on the Clinical and Laboratory Standards Institute (CLSI) MO7-A10 guidelines (9). Briefly, A. baumannii cultures were diluted in fresh Mueller-Hinton broth (Merck, Germany) overnight to achieve the culture OD at 600 nm reaching 1 × 108 CFU/mL. Then, to achieve 1 × 106 CFU/mL, the suspensions were diluted 1: 100 with Mueller-Hinton broth. Afterward, 50 μL of bacterial suspensions (1×106 CFU/mL) in addition to 50 μL of the two-fold serial dilutions of the dialyzed recombinants LL-37 (245 µg/mL) and oncorhyncin II (767 µg/mL) were placed in 96-well microtiter plates separately. Furthermore, 100 μL of bacterial suspension served as the positive control; nevertheless, 100 μL of sterilized Mueller-Hinton broth served as the negative control.
After 24 hours of incubation at 37°C, 20 μL of resazurin dyes [0.02% (w/v)] was added to each well and incubated again for 2 hours. The concentration of each treatment group in the last blue color well was regarded as the MIC value (10). Polymyxin E MIC against A. baumannii was performed by micro broth dilution according to the CLSI (22, 23). To evaluate the minimum bactericidal concentration (MBC), 100 μL of microplate blue wells related to MIC and the above-mentioned MIC values of LL-37 and oncorhyncin II were cultured on Mueller-Hinton agar. The term MBC is described as the lowest concentration of an antimicrobial agent that kills 99.9% of a certain organism following a 24-hour incubation period at 37°C. The antibacterial activity was characterized using the MBC/MIC ratio (MBC/MIC = 1 or 2 bactericidal, MBC/MIC = 4 or 16 bacteriostatic) (24).
3.4. Evaluation of Synergistic Activities of LL-37 and Oncorhyncin II
Checkerboard assays on 96-well microtiter plates containing LL-37 and Oncorhynchus II in two-fold serial concentrations were operated to evaluate the MICs of target recombinant proteins, either alone or in combination, in compliance with CLSI standards. In the horizontal wells, increasing concentrations of oncorhyncin II were used; nonetheless, increasing concentrations of LL-37 were used in the vertical wells. Bacterial suspensions were made and added to the wells at a 1 × 106 CFU/mL concentration. The recombinant peptides were serially diluted and incubated at 37°C for 18 - 24 hours. The fractional inhibitory concentration (FIC), which was determined as follows, was used to assess synergic interactions:
The fractional inhibitory concentration index (FICI) values were calculated by the following equation:
The results were defined within the following ranges: ≤ 0.5: Synergistic; 0.5 - 0.75: Partial synergy; 0.76 - 1.0: Additive; 1.0 - 4.0: Indifferent; > 4.0: Antagonistic (13, 25).
3.5. Time-Kill Assay
The current study used a time-kill experiment to determine the death rate of A. baumannii when treated with target recombinant antimicrobial proteins. A final density of 106 CFU/mL was used to prepare the inoculum. LL-37 and oncorhyncin II were used alone and in combination at 2 × MIC concentrations to determine their individual and combined effects on cell viability. To count the colonies, the samples were obtained at 0, 0.5, 1, 3, 5, 7, 11, and 20 hours after incubation and put into Mueller-Hinton agar. Kinetic tests were conducted using a 1 mL total volume. The synergistic effects and the reduction pattern of viable bacteria cell counts were evaluated after 24 hours of incubation at 37°C. Polymyxin E (1 μg/mL) was utilized as a positive control (13, 14); nevertheless, bacterial culture without additions was employed as a negative control. Antibacterial activity was defined as a decrease of ≥ 1 log10 in comparison to the initial inoculum. In this assay, the diction of ≥ 2 log10 and 1 ≤ log10 ≤ 2 were considered synergistic and additive, respectively. Each test was conducted in triplicate (26, 27).
3.6. Growth Kinetic Assay
The activity evaluation was obtained by the following assay: The mid-log phase bacterial cultures with a turbidity of 0.6 at 600 nm were diluted in Mueller-Hinton broth to obtain 108 CFU/mL concentration of cells (OD 600 nm = 0.2). Afterward, 200 μL of bacterial cultures were poured into single culture tubes, and 2 × MIC concentrations of LL-37 and oncorhyncin II alone or in combination were mixed in particular tubes and incubated at 37°C. The turbidity of these samples was determined with a spectrophotometer (at 600 nm) along the intervals of 0, 1, 3, 5, 7, 11, and 20 hours. The assay was carried out in triplicate (15). Polymyxin E (1 μg/mL) and untreated bacteria were used as positive and negative controls, respectively.
3.7. Statistical Analysis
All results were compared using a two-way analysis of variance. The results with P-values of less than 0.05 were considered statistically significant. Statistical analyses were performed using GraphPad Prism software (version 9).
4. Results
4.1. Expression, Purification, and Refolding of LL-37 and Oncorhyncin II in Escherichia coli
The proteins LL-37 and oncorhyncin II were successfully cloned in the pET32a expression vector. The recombinant proteins LL-37 and oncorhyncin II were successfully produced in E. coli BL21 cells as expression hosts treated with IPTG (1 mM) and were purified under the denaturing condition using nickel affinity chromatography (Ni-NTA; Qiagen, Valencia, Spain; Alameda, CA, USA) and urea 8 M. Subsequently, the quantity and quality of purified proteins were evaluated by SDS-PAGE. The successful expression of LL-37 (28 kDa) and oncorhyncin II (28 kDa) was confirmed by the presence of specific sharp bands indicating the 28 kDa proteins in 12% SDS gel. According to the findings of dialysis in PBS, the combination of arginine (0.1 M) and proline (0.1 M) amino acids at pH = 7 for LL-37 and pH = 8.5 for oncorhyncin II had the maximum protein yield efficiency. At OD 280 nm, protein concentrations were determined using a spectrophotometer (Eppendorf, Germany). Protein concentration was determined using 10 kDa pore-size Amicon centrifugal filters that indicated 245 and 767 μg/mL for LL-37 and oncorhyncin II, respectively.
4.2. Antimicrobial and Synergistic Activities of LL-37 and Oncorhyncin II
The MIC values for the recombinant LL-37 and oncorhyncin II proteins against A. baumannii can be observed in Table 1. Recombinant LL-37 and oncorhyncin II proteins obtained MIC values of 30.6 and 95.87 µg/mL, respectively. The polymyxin E MIC was also 0.5 μg/mL. Table 2 shows the MBC values of each peptide alone and in combination against A. baumannii. It was determined that both LL-37 and oncorhyncin II peptides were bacteriostatic following MBC tests. Checkerboard tests were used to confirm the synergistic activity of the LL-37/oncorhyncin II combination. The FICIs equal to 1 showed that LL-37 and oncorhyncin II were additive against A. baumannii. Table 1 summarizes the values of the FICI for each peptide and in combination.
Fractional Inhibitory Concentration Values of LL-37 in Combination with Oncorhyncin II against Acinetobacter baumannii (ATCC 19606)
Microorganism | Minimum Inhibitory Concentration (µg/mL) | Combination (µg/mL) | Minimum Inhibitory Concentration | Synergism | ||||
---|---|---|---|---|---|---|---|---|
LL-37a | FICa | Oncorhyncin IIb | FICb | LL-37c | Oncorhyncin IId | FICI | Additive | |
Acinetobacter baumannii | 30.6 | 0.5 | 95.87 | 0.5 | 15.3 | 47.93 | 1 |
Minimum Bactericidal Concentration Values of LL-37 and Oncorhyncin II Antimicrobial Proteins against Acinetobacter baumannii (ATCC 19606)
Microorganism | Minimum Bactericidal Concentration (µg/mL) | Minimum Bactericidal Concentration Combination (µg/mL) | ||||||
---|---|---|---|---|---|---|---|---|
LL-37a | Oncorhyncin IIa | LL-37b | Oncorhyncin IIb | |||||
Acinetobacter baumannii | MBC | MBC/MIC | MBC | MBC/MIC | MBC | MBC/MIC | MBC | MBC/MIC |
> 122.5 | > 4 | > 383.5 | > 4 | > 61.25 | > 4 | > 191.75 | > 4 | |
Interpretation | Bacteriostatic | Bacteriostatic | Bacteriostatic | Bacteriostatic |
4.3. Time-Kill Kinetics
Figure 1 shows the findings of the time-kill kinetics and synergy of LL-37 and oncorhyncin II against A. baumannii as measured by the changes in log10 CFU/mL of viable cells. At 2 × MIC of each combination, the number of viable cells against A. baumannii reduced dramatically, with more than 3 log10 CFU/mL reductions by LL-37 within 3 hours and more than 5 log10 CFU/mL reductions by oncorhyncin II within 11 hours. LL-37 also induced total cell death after 11 hours. Simultaneously, the combination of LL-37/oncorhyncin II resulted in a substantial drop in CFU after 3 hours and completed cell death after 7 hours. In addition, polymyxin E decreased log10 CFU/mL after 11 hours and did not significantly change the number of colonies over time. The results confirmed the reduction in the antibacterial efficiency of polymyxin E over time. Although the statistical analysis showed a very significant difference among tested treatments (P-values < 0.0001), there was no significant difference between polymyxin E and oncorhyncin II.
Bacterial-killing kinetics of LL-37 and oncorhyncin II at 2 × minimum inhibitory concentration against Acinetobacter baumannii; control (open triangles), A. baumannii + polymyxin E 1 μg/mL (open squares), LL-37 61.2 μg/mL (filled triangles), oncorhyncin II 191.74 μg/mL (filled circles), and combination of LL-37 30.6 μg/mL and oncorhyncin II 95.86 μg/mL (filled squares).
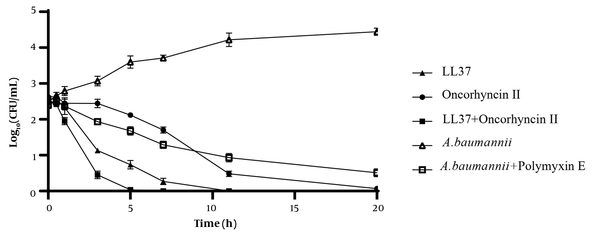
4.4. Growth Kinetics
For the purpose of determining the mode of action of LL-37 and oncorhyncin II against A. baumannii, the turbidity of the bacterial culture was shown at 2 × MIC of LL-37, and oncorhyncin II was measured over time using a spectrophotometer. As shown in Figure 2, LL-37 reduced the turbidity of A. baumannii suspensions by 50% and 100% after 1 and 5 hours, respectively. After 5 hours, oncorhyncin II lowered cell turbidity by over 95%. The combination of LL-37 and oncorhyncin II was much more effective in reducing the turbidity of A. baumannii suspensions, with a 100 % decrease in cell turbidity after 3 hours. In addition, the examination of polymyxin E at 2 × MIC as a control decreased cell turbidity after 11 hours. There were significant differences among all tested groups (P-values < 0.05).
Bacterial growth kinetics of LL-37 and oncorhyncin II at 2 × minimum inhibitory concentration against Acinetobacter baumannii; control (open triangles), A. baumannii + polymyxin E 1 μg/mL (open squares), LL-37 61.2 μg/mL (filled triangles), oncorhyncin II 191.74 μg/mL (filled circles), and combination of LL-37 30.6 μg/mL and oncorhyncin II 95.86 μg/mL (filled squares).
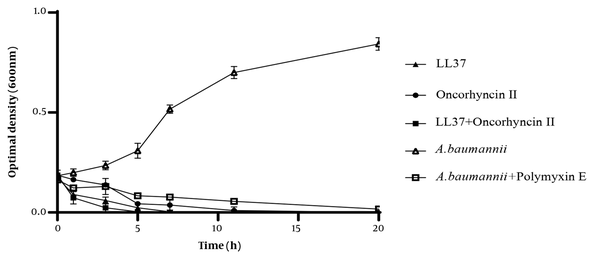
5. Discussion
The AMPs are considered a promising novel class of naturally derived therapeutic agents with broad antibacterial activities that can be advocated as possible replacements for antibiotics. The AMPs are polypeptides having cationic characteristics and fewer than 100 amino acids (28). The cationic AMPs interact with LPS in the outer membrane of Gram-negative bacteria to have a direct effect on the bacterial membrane. The destabilization of bacterial structure caused by the binding of AMP permits the intracellular absorption of antibiotics (29). There are a number of studies in the scientific literature on AMPs indicating synergistic effects with commercial antibiotics against MDR bacterial strains (13, 30).
To explore alternative therapies against A. baumannii, the pharmacokinetics and synergistic effects of LL-37 and oncorhyncin II recombinants were studied for the first time in the current investigation. LL-37 and oncorhyncin II are strong AMPs with diverse antimicrobial activities against Gram-positive and Gram-negative bacteria (31). In the current study, recombinant LL-37 and oncorhyncin II were effectively generated using the E. coli DE3 subspecies of BL21 as the expression host. Following purification processes, the recombinant proteins were readily recovered by nickel affinity chromatography with a purity of more than 70% (32). It has been confirmed in previous studies that the amino acids arginine and proline might increase the performance of proteins that have been reassembled utilizing a dialysis technique (15, 18). It is considered very valuable that the final produced recombinant LL-37 and oncorhyncin II in this study were effective against Gram-negative bacteria, including A. baumannii.
Several investigations have shown that LL-37 or its truncated analogs have antimicrobial activity against A. baumannii clinical and reference strains, consistent with the findings of the present study (30, 33, 34) Antibacterial, antibiofilm, and kinetics activities of LL-37 and its fragments (i.e., KR-12, KR-20, and KS-30) against A. baumannii reference strain were tested by Feng et al. In contrast to the findings of the present study, the MIC for ATCC 19606 in the aforementioned study was lower (16 μg/mL vs. 30.6 μg/mL) (14). Additionally, numerous investigations have indicated that ATCC 19606 strains of A. baumannii were more susceptible to LL-37 than clinical strains (30, 34, 35). Although oncorhyncin II’s antibacterial activity on A. baumannii has not yet been reported, numerous investigations have proven that histone-like proteins and peptides are effective against a wide range of significant fish diseases, including certain species of Vibrio and Aeromonas genera (e.g., Aeromonas salmonicida, A. hydrophila, V. anguillarum, V. alginolyticus, and V. salmonicida) (36-38).
According to a study conducted by Jacobsen et al., histone H1.2 had antimicrobial activity against the Pseudomonas aeruginosa reference strain and the burn wounds bacterial isolates (39). Moreover, Gram-negative bacteria, such as E. coli and L. anguillarum, were sensitive to a histone H1-derived oncorhyncin II protein that was isolated from the skin of rainbow trout (6). In another study, histone H1 was isolated as an AMP from the Atlantic salmon (Salmo salar) liver and was found to be effective against E. coli D31 (40). Synergy therapy could be used to enhance the antibacterial efficiencies of AMP against bacteria to expand the reducing cytotoxicity, the emergence inhibition of resistant bacterial mutants during therapy, antimicrobial or bactericidal spectrum, and achieving synergistic antimicrobial activity. For this purpose, the FICI was calculated using the checkerboard format and assessed synergy between LL-37 and oncorhyncin II. This technique yielded a FICI = 1 against A. baumannii (ATCC19606), which is indicative of an additive effect.
The outcomes of time-kill and growth-kinetic studies particularly validated the results of the checkerboard assay, revealing that the combination of recombinant peptides LL-37/oncorhyncin II is more efficient than either peptide alone and causes the death of A. baumannii in a shorter time. On A. baumannii, LL-37 was surprisingly more effective than oncorhyncin II. The use of polymyxin E as a control in these trials showed that the combination of LL-37 and oncorhyncin II is more effective than commercial antibiotics in killing A. baumannii in a shorter time. Furthermore, polymyxin E works better than oncorhyncin II on the desired bacterium in the early hours, although its effectiveness decreases over time. In agreement with the findings of the current study, the findings of prior investigations in this area have proven that the combination of LL-37 with other AMPs (7) or conventional antibiotics (30) has synergistic activity against A. baumannii (MDR) and sensitive and resistant P. aeruginosa strains.
5.1. Conclusions
LL-37 and oncorhyncin II recombinants were examined for the first time in this study for their pharmacokinetics and synergistic effects on A. baumannii. The findings of MIC, checkerboard, time-kill, and growth kinetics tests revealed that these peptides have potentially strong and quick antibacterial action against A. baumannii. Therefore, AMPs could be used as novel antibiotics, either alone or in combination with each other or as an adjunct to known antibiotics, to control and treat A. baumannii infections. The aforementioned findings should be taken into consideration when optimizing antibiotic therapy. Furthermore, clinical trials are required to determine the genuine worth of these peptides’ alternative therapies and therapeutic potential.
Acknowledgements
References
-
1.
Breslow JM, Meissler JJ, Hartzell RR, Spence PB, Truant A, Gaughan J, et al. Innate immune responses to systemic Acinetobacter baumannii infection in mice: neutrophils, but not interleukin-17, mediate host resistance. Infect Immun. 2011;79(8):3317-27. [PubMed ID: 21576323]. [PubMed Central ID: PMCPmc3147579]. https://doi.org/10.1128/iai.00069-11.
-
2.
Howard A, O'Donoghue M, Feeney A, Sleator RD. Acinetobacter baumannii: an emerging opportunistic pathogen. Virulence. 2012;3(3):243-50. [PubMed ID: 22546906]. [PubMed Central ID: PMCPmc3442836]. https://doi.org/10.4161/viru.19700.
-
3.
López-Rojas R, Jiménez-Mejías ME, Lepe JA, Pachón J. Acinetobacter baumannii resistant to colistin alters its antibiotic resistance profile: a case report from Spain. J Infect Dis. 2011;204(7):1147-8. [PubMed ID: 21881133]. https://doi.org/10.1093/infdis/jir476.
-
4.
Valencia R, Arroyo LA, Conde M, Aldana JM, Torres MJ, Fernández-Cuenca F, et al. Nosocomial outbreak of infection with pan-drug-resistant Acinetobacter baumannii in a tertiary care university hospital. Infect Control Hosp Epidemiol. 2009;30(3):257-63. [PubMed ID: 19199531]. https://doi.org/10.1086/595977.
-
5.
Adams MD, Nickel GC, Bajaksouzian S, Lavender H, Murthy AR, Jacobs MR, et al. Resistance to colistin in Acinetobacter baumannii associated with mutations in the PmrAB two-component system. Antimicrob Agents Chemother. 2009;53(9):3628-34. [PubMed ID: 19528270]. [PubMed Central ID: PMCPmc2737849]. https://doi.org/10.1128/aac.00284-09.
-
6.
Beceiro A, Llobet E, Aranda J, Bengoechea JA, Doumith M, Hornsey M, et al. Phosphoethanolamine modification of lipid A in colistin-resistant variants of Acinetobacter baumannii mediated by the pmrAB two-component regulatory system. Antimicrob Agents Chemother. 2011;55(7):3370-9. [PubMed ID: 21576434]. [PubMed Central ID: PMCPmc3122444]. https://doi.org/10.1128/aac.00079-11.
-
7.
Spencer JJ, Pitts RE, Pearson RA, King LB. The effects of antimicrobial peptides WAM-1 and LL-37 on multidrug-resistant Acinetobacter baumannii. Pathog Dis. 2018;76(2). [PubMed ID: 29370365]. https://doi.org/10.1093/femspd/fty007.
-
8.
Govender T, Dawood A, Esterhuyse AJ, Katerere DR. Antimicrobial properties of the skin secretions of frogs. S Afr J Sci. 2012;108(5/6). https://doi.org/10.4102/sajs.v108i5/6.795.
-
9.
Seefeldt AC, Nguyen F, Antunes S, Pérébaskine N, Graf M, Arenz S, et al. The proline-rich antimicrobial peptide Onc112 inhibits translation by blocking and destabilizing the initiation complex. Nat Struct Mol Biol. 2015;22(6):470-5. [PubMed ID: 25984971]. https://doi.org/10.1038/nsmb.3034.
-
10.
Dangel A, Ackermann N, Abdel-Hadi O, Maier R, Önder K, Francois P, et al. A de novo-designed antimicrobial peptide with activity against multiresistant Staphylococcus aureus acting on RsbW kinase. Faseb j. 2013;27(11):4476-88. [PubMed ID: 23901070]. https://doi.org/10.1096/fj.13-234575.
-
11.
Jenssen H, Hamill P, Hancock RE. Peptide antimicrobial agents. Clin Microbiol Rev. 2006;19(3):491-511. [PubMed ID: 16847082]. [PubMed Central ID: PMCPmc1539102]. https://doi.org/10.1128/cmr.00056-05.
-
12.
Yeung AT, Gellatly SL, Hancock RE. Multifunctional cationic host defence peptides and their clinical applications. Cell Mol Life Sci. 2011;68(13):2161-76. [PubMed ID: 21573784]. https://doi.org/10.1007/s00018-011-0710-x.
-
13.
Gopal R, Kim YG, Lee JH, Lee SK, Chae JD, Son BK, et al. Synergistic effects and antibiofilm properties of chimeric peptides against multidrug-resistant Acinetobacter baumannii strains. Antimicrob Agents Chemother. 2014;58(3):1622-9. [PubMed ID: 24366740]. [PubMed Central ID: PMCPmc3957903]. https://doi.org/10.1128/aac.02473-13.
-
14.
Feng X, Sambanthamoorthy K, Palys T, Paranavitana C. The human antimicrobial peptide LL-37 and its fragments possess both antimicrobial and antibiofilm activities against multidrug-resistant Acinetobacter baumannii. Peptides. 2013;49:131-7. [PubMed ID: 24071034]. https://doi.org/10.1016/j.peptides.2013.09.007.
-
15.
Jafari SF, Ghaznavi-Rad E, Fahimirad S, Abtahi H. Recombinant oncorhyncin II effect on the treatment of methicillin-resistant Staphylococcus aureus skin infection. Jundishapur J Microbiol. 2020;13(4). https://doi.org/10.5812/jjm.95948.
-
16.
Fernandes JM, Molle G, Kemp GD, Smith VJ. Isolation and characterisation of oncorhyncin II, a histone H1-derived antimicrobial peptide from skin secretions of rainbow trout, Oncorhynchus mykiss. Dev Comp Immunol. 2004;28(2):127-38. [PubMed ID: 12969798]. https://doi.org/10.1016/s0145-305x(03)00120-4.
-
17.
Sivashanmugam A, Murray V, Cui C, Zhang Y, Wang J, Li Q. Practical protocols for production of very high yields of recombinant proteins using Escherichia coli. Protein Sci. 2009;18(5):936-48. [PubMed ID: 19384993]. [PubMed Central ID: PMCPmc2771296]. https://doi.org/10.1002/pro.102.
-
18.
Fahimirad S, Ghaznavi-Rad E, Abtahi H, Sarlak N. Antimicrobial activity, stability and wound healing performances of chitosan nanoparticles loaded recombinant LL37 antimicrobial peptide. Int J Pept Res Ther. 2021;27(4):2505-15. https://doi.org/10.1007/s10989-021-10268-y.
-
19.
Yu H, Ma Z, Meng S, Qiao S, Zeng X, Tong Z, et al. A novel nanohybrid antimicrobial based on chitosan nanoparticles and antimicrobial peptide microcin J25 with low toxicity. Carbohydr Polym. 2021;253:117309. [PubMed ID: 33278958]. https://doi.org/10.1016/j.carbpol.2020.117309.
-
20.
Yamaguchi H, Miyazaki M. Refolding techniques for recovering biologically active recombinant proteins from inclusion bodies. Biomolecules. 2014;4(1):235-51. [PubMed ID: 24970214]. [PubMed Central ID: PMCPmc4030991]. https://doi.org/10.3390/biom4010235.
-
21.
Satei P, Ghaznavi-Rad E, Fahimirad S, Abtahi H. Recombinant production of Trx-Ib-AMP4 and Trx-E50-52 antimicrobial peptides and antimicrobial synergistic assessment on the treatment of methicillin-resistant Staphylococcus aureus under in vitro and in vivo situations. Protein Expr Purif. 2021;188:105949. [PubMed ID: 34324967]. https://doi.org/10.1016/j.pep.2021.105949.
-
22.
Clinical and Laboratory Standards Institute. M07-A10 Methods for Dilution Antimicrobial Susceptibility Tests for Bacteria That Grow Aerobically. Wayne, USA: Clinical and Laboratory Standards Institute; 2018.
-
23.
Schaub IG, Hauber FD. A biochemical and serological study of a group of identical unidentifiable gram-negative bacilli from human sources. J Bacteriol. 1948;56(4):379-85. [PubMed ID: 16561584]. [PubMed Central ID: PMCPmc518597]. https://doi.org/10.1128/jb.56.4.379-385.1948.
-
24.
Elshikh M, Ahmed S, Funston S, Dunlop P, McGaw M, Marchant R, et al. Resazurin-based 96-well plate microdilution method for the determination of minimum inhibitory concentration of biosurfactants. Biotechnol Lett. 2016;38(6):1015-9. [PubMed ID: 26969604]. [PubMed Central ID: PMC4853446]. https://doi.org/10.1007/s10529-016-2079-2.
-
25.
Lora-Tamayo J, Murillo O, Bergen PJ, Nation RL, Poudyal A, Luo X, et al. Activity of colistin combined with doripenem at clinically relevant concentrations against multidrug-resistant Pseudomonas aeruginosa in an in vitro dynamic biofilm model. J Antimicrob Chemother. 2014;69(9):2434-42. [PubMed ID: 24833752]. https://doi.org/10.1093/jac/dku151.
-
26.
Aghazadeh H, Memariani H, Ranjbar R, Pooshang Bagheri K. The activity and action mechanism of novel short selective LL-37-derived anticancer peptides against clinical isolates of Escherichia coli. Chem Biol Drug Des. 2019;93(1):75-83. [PubMed ID: 30120878]. https://doi.org/10.1111/cbdd.13381.
-
27.
Maisetta G, Batoni G, Esin S, Florio W, Bottai D, Favilli F, et al. In vitro bactericidal activity of human beta-defensin 3 against multidrug-resistant nosocomial strains. Antimicrob Agents Chemother. 2006;50(2):806-9. [PubMed ID: 16436752]. [PubMed Central ID: PMCPmc1366902]. https://doi.org/10.1128/aac.50.2.806-809.2006.
-
28.
Ganz T. Defensins: antimicrobial peptides of innate immunity. Nat Rev Immunol. 2003;3(9):710-20. [PubMed ID: 12949495]. https://doi.org/10.1038/nri1180.
-
29.
Geitani R, Ayoub Moubareck C, Touqui L, Karam Sarkis D. Cationic antimicrobial peptides: alternatives and/or adjuvants to antibiotics active against methicillin-resistant Staphylococcus aureus and multidrug-resistant Pseudomonas aeruginosa. BMC Microbiol. 2019;19(1):54. [PubMed ID: 30849936]. [PubMed Central ID: PMCPmc6408789]. https://doi.org/10.1186/s12866-019-1416-8.
-
30.
Neshani A, Zare H, Akbari Eidgahi MR, Kamali Kakhki R, Safdari H, Khaledi A, et al. LL-37: Review of antimicrobial profile against sensitive and antibiotic-resistant human bacterial pathogens. Gene Reports. 2019;17:100519. https://doi.org/10.1016/j.genrep.2019.100519.
-
31.
Leszczyńska K, Namiot A, Janmey PA, Bucki R. Modulation of exogenous antibiotic activity by host cathelicidin LL-37. Apmis. 2010;118(11):830-6. [PubMed ID: 20955455]. [PubMed Central ID: PMCPmc3386844]. https://doi.org/10.1111/j.1600-0463.2010.02667.x.
-
32.
Miragaia M. Factors contributing to the evolution of Meca-mediated β-lactam resistance in staphylococci: update and new insights from whole genome sequencing (WGS). Front Microbiol. 2018;9:2723. [PubMed ID: 30483235]. [PubMed Central ID: PMCPmc6243372]. https://doi.org/10.3389/fmicb.2018.02723.
-
33.
Guo Y, Wang L, Lei J, Xu J, Han L. Antimicrobial and antibiofilm activity of human cationic antibacterial peptide (Ll-37) and its analogs against Pan-Drug-resistant Acinetobacter baumannii. Jundishapur J Microbiol. 2017;10(3). https://doi.org/10.5812/jjm.35857.
-
34.
Mohamed MF, Brezden A, Mohammad H, Chmielewski J, Seleem MN. A short D-enantiomeric antimicrobial peptide with potent immunomodulatory and antibiofilm activity against multidrug-resistant Pseudomonas aeruginosa and Acinetobacter baumannii. Sci Rep. 2017;7(1):6953. [PubMed ID: 28761101]. [PubMed Central ID: PMCPmc5537347]. https://doi.org/10.1038/s41598-017-07440-0.
-
35.
García-Quintanilla M, Pulido MR, Moreno-Martínez P, Martín-Peña R, López-Rojas R, Pachón J, et al. Activity of host antimicrobials against multidrug-resistant Acinetobacter baumannii acquiring colistin resistance through loss of lipopolysaccharide. Antimicrob Agents Chemother. 2014;58(5):2972-5. [PubMed ID: 24566189]. [PubMed Central ID: PMCPmc3993257]. https://doi.org/10.1128/aac.02642-13.
-
36.
Robinette D, Wada S, Arroll T, Levy MG, Miller WL, Noga EJ. Antimicrobial activity in the skin of the channel catfish Ictalurus punctatus: characterization of broad-spectrum histone-like antimicrobial proteins. Cell Mol Life Sci. 1998;54(5):467-75. [PubMed ID: 9645227]. https://doi.org/10.1007/s000180050175.
-
37.
Birkemo GA, Lüders T, Andersen Ø, Nes IF, Nissen-Meyer J. Hipposin, a histone-derived antimicrobial peptide in Atlantic halibut (Hippoglossus hippoglossus L.). Biochim Biophys Acta. 2003;1646(1-2):207-15. [PubMed ID: 12637028]. https://doi.org/10.1016/s1570-9639(03)00018-9.
-
38.
Lüders T, Birkemo GA, Nissen-Meyer J, Andersen Ø, Nes IF. Proline conformation-dependent antimicrobial activity of a proline-rich histone h1 N-terminal Peptide fragment isolated from the skin mucus of Atlantic salmon. Antimicrob Agents Chemother. 2005;49(6):2399-406. [PubMed ID: 15917539]. [PubMed Central ID: PMCPmc1140541]. https://doi.org/10.1128/aac.49.6.2399-2406.2005.
-
39.
Jacobsen F, Baraniskin A, Mertens J, Mittler D, Mohammadi-Tabrisi A, Schubert S, et al. Activity of histone H1.2 in infected burn wounds. J Antimicrob Chemother. 2005;55(5):735-41. [PubMed ID: 15772144]. https://doi.org/10.1093/jac/dki067.
-
40.
Richards RC, O'Neil DB, Thibault P, Ewart KV. Histone H1: an antimicrobial protein of Atlantic salmon (Salmo salar). Biochem Biophys Res Commun. 2001;284(3):549-55. [PubMed ID: 11396934]. https://doi.org/10.1006/bbrc.2001.5020.