Abstract
Background:
Carbapenem-resistant Pseudomonas aeruginosa is an endemic problem in several countries, notably Turkey.Objectives:
This study aimed to investigate the underlying mechanisms contributing to the carbapenem resistance phenotype and enhance the in-vitro activity of ceftolozane-tazobactam (C/T) and ceftazidime-avibactam (C/A) against carbapenem-resistant P. aeruginosa strains.Methods:
A total of 114 carbapenem-resistant P. aeruginosa strains were isolated from different types of clinical specimens. The tested antibiotics were evaluated using the antibiotic disk diffusion method. Additionally, C/T and C/A were tested using the gradient test method. The efficacy of phenylalanine-arginine-β-naphthylamide (PAβN) as efflux pump inhibitors was assessed to determine their ability to reduce meropenem minimum inhibitory concentrations. Polymerase chain reaction (PCR) assays were conducted to identify blaIMP, blaVIM, and blaNDM-1.Results:
Among 114 strains of carbapenem-resistant P. aeruginosa, overall resistance rates for C/T and C/A were 10.7% and 8.8%, respectively. Efflux pump inhibitor-based antibiotic susceptibility testing indicated that 35.08% of strains showed resistance modulated by PAβN. Among the strains, 27 (24.5%) were found to produce metallo-beta-lactamase (MBL), with blaVIM (17 strains, 14.91%) being the most common, followed by blaIMP (12 strains, 10.53%).Conclusions:
Emerging carbapenem resistance in P. aeruginosa strains is a serious therapeutic challenge for clinicians. Carbapenem resistance can be influenced by various factors, some of which were not assessed in our study. Nonetheless, our results revealed that the main mechanism associated with carbapenem-resistant P. aeruginosa strains is a PAβN-sensitive efflux pump. Among acquired MBLs, VIM-type enzymes were found to be the most prevalent.Keywords
Carbapenems Efflux Pumps PaβN Pseudomonas aeruginosa Ceftolozane/Tazobactam Ceftazidime/Avibactam
1. Background
Pseudomonas aeruginosa is a non-fermenting Gram-negative bacillus that can cause nosocomial infections resulting in sepsis, urinary tract infections, endocarditis, and pneumonia (1). Pseudomonas aeruginosa exhibits resistance to various antibiotics, including cefixime, penicillin G, kanamycin, aminopenicillins (alone or in combination with inhibitors), ceftriaxone, first- and second-generation cephalosporins, cefotaxime, ertapenem, and trimethoprim. This bacterium has the ability to rapidly acquire additional resistances, leading to treatment failures (2, 3). Antipseudomonal carbapenems are commonly used to manage infections caused by multidrug-resistant (MDR) P. aeruginosa. However, their increased utilization has resulted in the development of carbapenem resistance, which hampers the effective treatment of P. aeruginosa infections (4). Carbapenem resistance in P. aeruginosa can arise from various mechanisms, including the production of carbapenemases, over-expression of efflux pumps, loss of outer membrane porins, and the generation of extended-spectrum AmpC β-lactamase or β-lactamase (4).
The Ambler class B metallo-β-lactamases (MBLs) are the primary carbapenemases found in PA. MBL-encoding genes can be present on plasmids and transferred to other strains, leading to antibiotic resistance. Pseudomonas aeruginosa can harbor various types of MBLs, including IMP, NDM-1, VIM, GIM, SPM, FIM-1, SIM, and HMB-1 (5). Overexpression of MexAB-OprM, a member of the resistance nodulation-cell division (RND) family and a multidrug efflux pump, contributes to carbapenem resistance in P. aeruginosa (6). Ceftolozane-tazobactam (C/T) and ceftazidime-avibactam (C/A) are novel β-lactam/β-lactamase inhibitor combinations effective against Gram negative bacteria, including P. aeruginosa. They are potential therapeutic options for infections caused by carbapenem-resistant P. aeruginosa strains (2, 7). C/T exhibits stability against chromosomal AmpC β-lactamases, deleted OprD porins, and overexpressed MexAB-OprM efflux pumps. However, both C/T and C/A are ineffective against MBL-producing P. aeruginosa (8).
2. Objectives
We examined (1) the presence of efflux pumps using a phenotypic method, (2) the distribution of blaIMP, blaVIM, and blaNDM-1 genes among carbapenem-resistant P. aeruginosa strains, and (3) the in-vitro activity of C/A and C/T against carbapenem-resistant P. aeruginosa strains. These antibiotics are recognized for their high efficacy against carbapenem-resistant P. aeruginosa but have been insufficiently studied in Turkey.
3. Methods
3.1. Bacterial Strains
In the present study, a total of 114 non-duplicate clinical strains of meropenem-non-susceptible P. aeruginosa were examined. These strains were obtained from Hacettepe University Hospitals between 2019 and 2020. The specimens originated from various sources, including blood (n = 14), lower respiratory samples such as tracheal aspirate and sputum (n = 39), pus (n = 12), sterile body fluids such as peritoneal, pleural, and bile fluids (n = 13), tissue (n = 6), and urine (n = 30). Prior to the assessment, all strains were stored at 80°C and subcultured twice on 5% sheep blood agar.
3.2. Antimicrobial Susceptibility Testing
In vitro, antibiotic susceptibility testing was performed using the Phoenix™ Automated Microbiology System (BD Becton-Dickinson, Sparks, USA) to assess the susceptibility of ceftazidime (CAZ), amikacin (AMK), piperacillin/tazobactam (TZP), cefepime (FEP), and ciprofloxacin (CIP) (9). The minimum inhibitory concentration (MIC) of meropenem was determined using the micro broth dilution technique. Gradient test strips (biomerieux, France) were utilized to measure the MICs of C/T and C/A. The interpretation of the antibiotic susceptibility test results was based on the European Committee on Antimicrobial Susceptibility Testing (EUCAST) breakpoint tables (version 9.0) (10). Pseudomonas aeruginosa ATCC 27853 was used as a quality control strain.
3.3. Efflux Pump Inhibition
To investigate the interaction between meropenem (Sigma-Aldrich, USA) and phenylalanine arginine β-naphthylamide (PAβN, Sigma-Aldrich, Germany), the MICs of the antibiotics were measured in the presence and absence of PAβN at a concentration of 25 mg/L (11, 12). The strains overexpressing efflux pumps exhibited at least a four-fold decrease in MICs in the presence of PAβN compared to the MICs in the absence of PAβN (12). The strains were assessed in duplicate, and the results were screened 24 hours after incubation at 35°C.
3.4. Detection of Carbapenemase-Producing Genes
All carbapenem-resistant P. aeruginosa strains were examined for the presence of carbapenemase-encoding genes, including blaIMP, blaVIM, and blaNDM-1, using the polymerase chain reaction (PCR) method with minor modifications as previously described (13-15). For DNA isolation, 4 - 5 single colonies were picked from fresh cultures and incubated at 95°C for 15 min in PCR-grade water. Following centrifugation at 10,000 rpm for 10 min, 2 μL of the supernatant was used as the DNA template in a final volume of 20 μL, which included 1X PCR Buffer, 2.5 mM MgCl2, 0.5 µM of each primer, 200 µM of each dNTP, and 1U Taq DNA polymerase. The specific primer sequences are presented in Table 1. The cycling conditions comprised an initial denaturation at 94°C for 5 min, followed by 35 cycles of 94°C for 30 s, 52°C for 40 s, and 72°C for 50 s, with a final elongation step at 72°C for 5 min (see Appendix 1).
Primers Used for PCR Amplification of Metallo-β-Lactamase Genes
4. Results
4.1. Antimicrobial Susceptibility Patterns in Carbapenem-Resistant Pseudomonas aeruginosa Strains
Among the 114 carbapenem-resistant P. aeruginosa strains, the overall resistance rates were 10.7% for C/T and 8.8% for C/A. The MIC50 values for C/A and C/T were 1 mg/mL and 0.75 mg/mL, respectively. The MIC90 values for C/T and C/A were 48 mg/mL and 16 mg/mL, respectively. Based on the MIC90 values, C/A demonstrated greater potency than C/T. The majority of strains (94.7%, n = 108) showed categorical agreement with both C/T and C/A. However, six strains were susceptible to C/A but resistant to C/T. Among the five antibiotics tested on carbapenem-resistant P. aeruginosa strains, amikacin exhibited the highest potency (19.5% resistance), followed by FEP (44.4% resistance), CAZ (46.4% resistance), and TZP (51.8% resistance). The highest resistance was observed against CIP (62.2%) (Figure 1A). Table 2 presents the susceptibility test results for other antimicrobial agents. Four strains were classified as MDR and harbored both MBLs, exhibiting a four-fold reduction in meropenem MIC in the presence of the efflux pump inhibitor PAßN.
Phenotypic Resistance in Clinical Strains of Carbapenem-Resistant Pseudomonas aeruginosa
Antimicrobial Class | Antimicrobial Agents | MIC50 | MIC90 | Resistance (%) |
---|---|---|---|---|
β-lactam (carbapenem) | Meropenem | > 8 | > 8 | 88.50 |
β-lactam (carbapenem) | Imipenem | > 8 | > 8 | 97.3 |
β-lactam (cephalosporin) | Ceftazidime | 4 | > 16 | 46.4 |
β-lactam (cephalosporin) | Cefepime | 4 | > 8 | 44.4 |
β-lactam (penicillin)/β-lactamase inhibitor | Piperacillin/tazobactam | 4 | 32 | 51.8 |
β-lactam (cephalosporin)/ β-lactamase inhibitor | Ceftazidim/avibactam | 1 | 16 | 8.8 |
β-lactam (cephalosporin)/β-lactamase inhibitor | Ceftolozane/tazobactam | 0.75 | 48 | 10.7 |
Aminoglycoside | Amikacin | < 8 | > 32 | 19.5 |
Quinolone | Ciprofloxacin | 0.5 | > 1 | 62.2 |
4.2. Efflux Pump Inhibition
In carbapenem-resistant P. aeruginosa strains, 35.08% of the strains have become susceptible to 25 mg/L of PAβN, resulting in a decrease in MICs of meropenem in 40 P. aeruginosa strains. The bacteria cultured in Mueller-Hinton broth containing PAΒN (25 mg/L), with or without meropenem, exhibited robust growth, indicating that PAΒN (25 mg/L) itself did not have an antibacterial effect. Among the 40 strains with PAβN-sensitive efflux pumps, five strains were found to have MBLs (Figure 1B).
4.3. Detection of Carbapenemase-Producing Genes
Among the 114 strains of carbapenem-resistant P. aeruginosa, 27 (24.5%) strains were found to produce MBL. The most common carbapenemase gene was blaVIM, detected in 17 strains (14.91%), followed by blaIMP in 12 strains (10.53%). No positive strains for blaNDM-1 were identified. Two strains were positive for both blaIMP and blaVIM simultaneously (Figure 1B). The overall distribution of carbapenemase genes, efflux pump, resistance rates to tested antibiotics, and coexistence of blaIMP, blaVIM, and phenotypic efflux pump test-positive strains are depicted in Figure 1A and B. A heat map in Figure 1C represents the percentage distribution of resistant strains for all tested antibiotics, the prevalence of MBLs, and the presence of 4 phenotypic efflux pump inhibitors based on sample origin.
A, Percentage distribution of carbapenemase genes, efflux pump-positive strains based on phenotypic tests, and resistance rates to tested antibiotics. B, Coexistence of blaIMP, blaVIM, and phenotypic efflux pump test-positive strains. C, Heat map displaying the percentage distribution of resistant isolates for all tested antibiotics, the prevalence of MBLs, and phenotypic efflux pump inhibitors according to sample origin. The color gradient ranges from green to red, representing a linear scale of percent distribution from low to high. The red box indicates a higher prevalence compared to the green box.
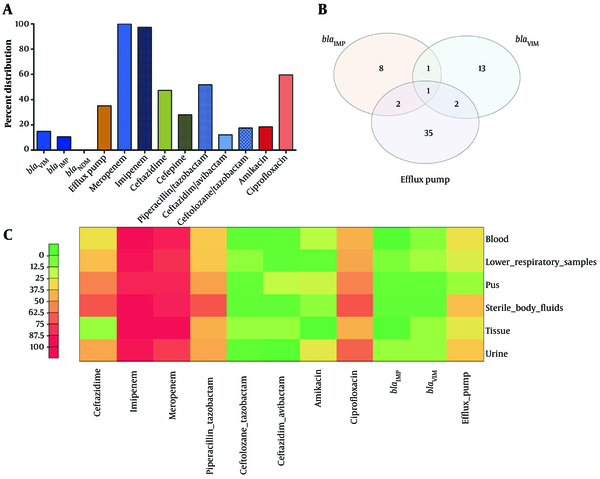
5. Discussion
Carbapenem resistance in P. aeruginosa strains poses a significant clinical challenge, as carbapenems have been widely considered the most potent and effective agents against MDR P. aeruginosa strains. However, there has been a recent rise in the prevalence of carbapenem resistance among P. aeruginosa strains globally, including in Turkey (13, 16, 17). According to the Antimicrobial Testing Leadership and Surveillance program (18), the lowest rate of carbapenem-resistant P. aeruginosa was observed in Oceania (5.1% - 7.1%), while the highest rates were found in the Middle East (27.9% - 19.5%). Resistance rates were 30.7% in South America, 28.0% in Europe, 24.4% in North America, 22.8% in Africa, and 18.1% in Asia (19). A meta-analysis conducted in Turkey reported a pooled prevalence of 30.1% for resistance against meropenem and 28.0% for imipenem (17). Among carbapenem-resistant P. aeruginosa clinical strains, MBLs are the most common type of carbapenemases. VIMs are the most widely distributed (Middle East, South America, Africa), followed by IMPs and NDMs (20).
Kazmierczak et al. studied carbapenemases in P. aeruginosa strains collected from 96 medical centers across 18 European countries. They found that 13.4% of the strains carried MBLs, with VIM being the most prevalent (21). In Turkey, P. aeruginosa strains exhibit various types of MBLs, including VIM-1, VIM-2, VIM-5, and IMP (22-25). The first isolation of NDM-1-producing P. aeruginosa was reported in 2011 in Serbia, and subsequent cases of NDM-positive strains have been identified in Italy, France, Egypt, and Slovakia (26-30). In Turkey, a few P. aeruginosa strains producing NDM-1 have been identified (24, 31). However, in our study, no NDM-1-positive strains were detected, suggesting that the presence of NDM-1 may be limited to a localized area. In the current study, the detection rate of MBLs was 24.5%, with VIM being the most common type. These findings highlight the high prevalence of carbapenemase genes among the strains, emphasizing the importance of their detection as they can easily disseminate among bacterial strains. Ongoing surveillance is necessary to identify potential outbreaks.
Efflux pump overexpression, particularly MexXY, and MexAB-OprM, contributes to increased resistance to carbapenems in P. aeruginosa strains (6). The reported prevalence rates of efflux pump overexpression in carbapenem-resistant P. aeruginosa strains range from 37.5% to 80.9% (13, 32, 33). In a Turkish study, efflux pump overexpression was found in 47.6% of carbapenem-resistant P. aeruginosa strains (34). It is worth noting that meropenem is a substrate for efflux pumps (35). In the presence of PaβN, approximately 35.9% of the strains under investigation exhibited a significant decrease in meropenem MICs, irrespective of the presence of any MBL genes. The efflux pump plays a crucial role in conferring carbapenem resistance in the strains examined. However, it is important to acknowledge that our study had limitations, including the lack of information regarding the expression of AmpC, other class C beta-lactamases, efflux pumps, and oprD gene mutations.
Treating infections caused by carbapenem-resistant P. aeruginosa strains poses a challenge due to their increased antibiotic resistance. Consistent with previous studies, these strains exhibit elevated resistance to various antibiotic groups, including quinolones, cephalosporins, and penicillins (16, 17). In our study, we assessed the in vitro activity of C/A against carbapenem-resistant P. aeruginosa strains and observed that the MICs of C/A were 2 - 4 times lower than the MICs for CAZ alone. This aligns with other in vitro studies that have demonstrated a smaller MIC for CZA compared to CAZ alone, indicating increased susceptibility of P. aeruginosa to C/A (36).
The susceptibility rates of carbapenem-resistant P. aeruginosa strains to C/T and C/A were 89.3% and 91.2%, respectively. In previous reports, carbapenem-resistant P. aeruginosa strains demonstrated susceptibility rates ranging from 51.8% to 92% for C/A and 65.4% to 94% for C/T (7, 37-40). Many studies have consistently shown higher susceptibility rates for C/T compared to C/A among carbapenem-resistant P. aeruginosa strains (7, 37, 38, 41). However, in contrast to these previous studies, our findings indicate that C/A exhibited greater activity than C/T against carbapenem-resistant P. aeruginosa strains.
Our results align with those of Sader et al., who observed higher susceptibility rates for C/A (86.5%) compared to C/T (66.2%) among meropenem non-susceptible P. aeruginosa strains (40). This difference in susceptibility between C/A and C/T may be attributed to the ability of avibactam to suppress class A β-lactamases, class C β-lactamases, and class D β-lactamases, which is not seen with tazobactam (42). Moreover, variations in the activity of C/A compared to C/T among P. aeruginosa strains reflect the diverse resistance mechanisms expressed by these organisms, highlighting how these mechanisms can have different impacts on the efficacy of these two combinations.
Carbapenem-resistant P. aeruginosa strains were isolated from 2019 to 2021 across 17 centers in 12 countries as part of the ERACE-PA Global Surveillance Program. Among these strains, the most commonly identified carbapenemase genotype was VIM. The observed susceptibility rates of VIM-positive strains to C/T and C/A were 1% and 4%, respectively (20). In the ATLAS global surveillance program, which collected 214 MBL-positive P. aeruginosa strains from 2017 to 2019, 4.2% of the strains were found to be susceptible to C/A (18). Lomovskaya et al. determined that the susceptibility rates of MBLs-producing P. aeruginosa strains to C/T and C/A were 1.6% and 4.9%, respectively (43). Within our collection, VIM is the predominant carbapenemase produced by carbapenem-resistant P. aeruginosa strains.
The susceptibility rates to C/T and C/A among MBL-positive strains were 55.1% and 62.4%, respectively. Adam and Elhag previously reported the presence of MBL genes in carbapenem-sensitive Gram-negative strains, suggesting the potential presence of hidden MBL genes (44). In our study, we identified MBL genes in strains that were reported as susceptible to C/T and C/A, indicating that these strains may serve as reservoirs for these resistance genes, posing a potential risk for silent dissemination in both community and hospital settings. The emergence of meropenem resistance in our clinical strains of P. aeruginosa, where efflux pump activity was not detected, may be attributed to constitutive overproduction of the cephalosporinase AmpC or non-enzymatic mechanisms such as outer membrane impermeability. However, it is essential to note that our study did not analyze these resistance mechanisms in meropenem-resistant P. aeruginosa strains, which is a limitation of our findings.
5.1. Conclusions
In this study, the main mechanism associated with carbapenem-resistant P. aeruginosa strains is the PAβN-sensitive efflux pump. Among the acquired carbapenemases in P. aeruginosa strains, VIM-type enzymes are the most commonly observed with a worldwide distribution. C/T and C/A have demonstrated their retained in vitro potency against clinical strains of carbapenem-resistant P. aeruginosa collected from hospitalized patients in Turkey. These findings suggest that C/T and C/A may play a potentially important role in the management of P. aeruginosa infections, including those caused by carbapenem-resistant strains. However, it is crucial to consider local susceptibility patterns and antibiotic susceptibility test results when deciding on the usage of C/T and C/A, as is the case with all antimicrobials.
References
-
1.
Livermore DM. Multiple mechanisms of antimicrobial resistance in Pseudomonas aeruginosa: our worst nightmare? Clin Infect Dis. 2002;34(5):634-40. [PubMed ID: 11823954]. https://doi.org/10.1086/338782.
-
2.
Sader HS, Castanheira M, Mendes RE, Flamm RK, Farrell DJ, Jones RN. Ceftazidime-avibactam activity against multidrug-resistant Pseudomonas aeruginosa isolated in U.S. medical centers in 2012 and 2013. Antimicrob Agents Chemother. 2015;59(6):3656-9. [PubMed ID: 25845861]. [PubMed Central ID: PMC4432162]. https://doi.org/10.1128/AAC.05024-14.
-
3.
Torrens G, Cabot G, Ocampo-Sosa AA, Conejo MC, Zamorano L, Navarro F, et al. Activity of ceftazidime-avibactam against clinical and isogenic laboratory pseudomonas aeruginosa isolates expressing combinations of most relevant beta-lactam resistance mechanisms. Antimicrob Agents Chemother. 2016;60(10):6407-10. [PubMed ID: 27480848]. [PubMed Central ID: PMC5038310]. https://doi.org/10.1128/AAC.01282-16.
-
4.
Lin KY, Lauderdale TL, Wang JT, Chang SC. Carbapenem-resistant Pseudomonas aeruginosa in Taiwan: Prevalence, risk factors, and impact on outcome of infections. J Microbiol Immunol Infect. 2016;49(1):52-9. [PubMed ID: 24662016]. https://doi.org/10.1016/j.jmii.2014.01.005.
-
5.
Viedma E, Juan C, Villa J, Barrado L, Orellana MA, Sanz F, et al. VIM-2-producing multidrug-resistant Pseudomonas aeruginosa ST175 clone, Spain. Emerg Infect Dis. 2012;18(8):1235-41. [PubMed ID: 22840969]. [PubMed Central ID: PMC3414013]. https://doi.org/10.3201/eid1808.111234.
-
6.
Poole K. Pseudomonas aeruginosa: resistance to the max. Front Microbiol. 2011;2:65. [PubMed ID: 21747788]. [PubMed Central ID: PMC3128976]. https://doi.org/10.3389/fmicb.2011.00065.
-
7.
Humphries RM, Hindler JA, Wong-Beringer A, Miller SA. Activity of ceftolozane-tazobactam and ceftazidime-avibactam against beta-lactam-resistant pseudomonas aeruginosa isolates. Antimicrob Agents Chemother. 2017;61(12). [PubMed ID: 28993338]. [PubMed Central ID: PMC5700315]. https://doi.org/10.1128/AAC.01858-17.
-
8.
Kresken M, Korber-Irrgang B, Korte-Berwanger M, Pfennigwerth N, Gatermann SG, Seifert H, et al. Dissemination of carbapenem-resistant Pseudomonas aeruginosa isolates and their susceptibilities to ceftolozane-tazobactam in Germany. Int J Antimicrob Agents. 2020;55(6):105959. [PubMed ID: 32325200]. https://doi.org/10.1016/j.ijantimicag.2020.105959.
-
9.
EUCAST. Antimicrobial susceptibility testing EUCAST disk diffusion method, Version 6.0. 2017. Available from: http://www.eucast.org/ast_of_bacteria/previous_versions_of_documents.
-
10.
EUCAST. Breakpoint tables for interpretation of MICs and zone diameters, version 10.0. 2023. Available from: http://www.eucast.org/clinical_breakpoints.
-
11.
Pannek S, Higgins PG, Steinke P, Jonas D, Akova M, Bohnert JA, et al. Multidrug efflux inhibition in Acinetobacter baumannii: comparison between 1-(1-naphthylmethyl)-piperazine and phenyl-arginine-beta-naphthylamide. J Antimicrob Chemother. 2006;57(5):970-4. [PubMed ID: 16531429]. https://doi.org/10.1093/jac/dkl081.
-
12.
Vera-Leiva A, Carrasco-Anabalon S, Lima CA, Villagra N, Dominguez M, Bello-Toledo H, et al. The efflux pump inhibitor phenylalanine-arginine beta-naphthylamide (PAbetaN) increases resistance to carbapenems in Chilean clinical isolates of KPC-producing Klebsiella pneumoniae. J Glob Antimicrob Resist. 2018;12:73-6. [PubMed ID: 29275225]. https://doi.org/10.1016/j.jgar.2017.12.003.
-
13.
Rostami S, Farajzadeh Sheikh A, Shoja S, Farahani A, Tabatabaiefar MA, Jolodar A, et al. Investigating of four main carbapenem-resistance mechanisms in high-level carbapenem resistant Pseudomonas aeruginosa isolated from burn patients. J Chin Med Assoc. 2018;81(2):127-32. [PubMed ID: 29033110]. https://doi.org/10.1016/j.jcma.2017.08.016.
-
14.
Poirel L, Walsh TR, Cuvillier V, Nordmann P. Multiplex PCR for detection of acquired carbapenemase genes. Diagn Microbiol Infect Dis. 2011;70(1):119-23. [PubMed ID: 21398074]. https://doi.org/10.1016/j.diagmicrobio.2010.12.002.
-
15.
Nordmann P, Poirel L, Carrer A, Toleman MA, Walsh TR. How to detect NDM-1 producers. J Clin Microbiol. 2011;49(2):718-21. [PubMed ID: 21123531]. [PubMed Central ID: PMC3043507]. https://doi.org/10.1128/JCM.01773-10.
-
16.
Doi Y. Treatment options for carbapenem-resistant Gram-negative bacterial infections. Clin Infect Dis. 2019;69(Suppl 7):S565-75. [PubMed ID: 31724043]. [PubMed Central ID: PMC6853760]. https://doi.org/10.1093/cid/ciz830.
-
17.
Acar A, Karaahmetoglu G, Akalin H, Altay AF. Pooled prevalence and trends of antimicrobial resistance in Pseudomonas aeruginosa clinical isolates over the past 10 years in Turkey: A meta-analysis. J Glob Antimicrob Resist. 2019;18:64-70. [PubMed ID: 30753904]. https://doi.org/10.1016/j.jgar.2019.01.032.
-
18.
Karlowsky JA, Kazmierczak KM, Valente M, Luengas EL, Baudrit M, Quintana A, et al. In vitro activity of ceftazidime-avibactam against Enterobacterales and Pseudomonas aeruginosa isolates collected in Latin America as part of the ATLAS global surveillance program, 2017-2019. Braz J Infect Dis. 2021;25(6):101647. [PubMed ID: 34774471]. [PubMed Central ID: PMC9392196]. https://doi.org/10.1016/j.bjid.2021.101647.
-
19.
Antimicrobial testing leadership and surveillance. 2022. Available from: https://atlas-surveillance.com.
-
20.
Gill CM, Aktathorn E, Alfouzan W, Bourassa L, Brink A, Burnham CD, et al. The ERACE-PA global surveillance program: Ceftolozane/tazobactam and ceftazidime/avibactam in vitro activity against a global collection of carbapenem-resistant pseudomonas aeruginosa. Eur J Clin Microbiol Infect Dis. 2021;40(12):2533-41. [PubMed ID: 34291323]. [PubMed Central ID: PMC8590662]. https://doi.org/10.1007/s10096-021-04308-0.
-
21.
Kazmierczak KM, de Jonge BLM, Stone GG, Sahm DF. In vitro activity of ceftazidime/avibactam against isolates of Pseudomonas aeruginosa collected in European countries: INFORM global surveillance 2012-15. J Antimicrob Chemother. 2018;73(10):2777-81. [PubMed ID: 30010951]. https://doi.org/10.1093/jac/dky267.
-
22.
Er H, Altindis M, Asik G, Demir C. [Molecular epidemiology of beta-lactamases in ceftazidime-resistant Pseudomonas aeruginosa isolates]. Mikrobiyol Bul. 2015;49(2):156-65. [PubMed ID: 26167816]. https://doi.org/10.5578/mb.8901.
-
23.
Yilmaz NO, Agus N, Bozcal E, Uzel A. Prevalence and molecular characterisation of metallo-beta-lactamase producing strains of imipenem-resistant Pseudomonas aeruginosa in Turkey. Indian J Med Microbiol. 2014;32(3):349-50. [PubMed ID: 25008840]. https://doi.org/10.4103/0255-0857.136622.
-
24.
Malkocoglu G, Aktas E, Bayraktar B, Otlu B, Bulut ME. VIM-1, VIM-2, and GES-5 Carbapenemases among Pseudomonas aeruginosa isolates at a Tertiary Hospital in Istanbul, Turkey. Microb Drug Resist. 2017;23(3):328-34. [PubMed ID: 27326514]. https://doi.org/10.1089/mdr.2016.0012.
-
25.
Bahar G, Mazzariol A, Koncan R, Mert A, Fontana R, Rossolini GM, et al. Detection of VIM-5 metallo-beta-lactamase in a Pseudomonas aeruginosa clinical isolate from Turkey. J Antimicrob Chemother. 2004;54(1):282-3. [PubMed ID: 15190017]. https://doi.org/10.1093/jac/dkh321.
-
26.
Jovcic B, Lepsanovic Z, Suljagic V, Rackov G, Begovic J, Topisirovic L, et al. Emergence of NDM-1 metallo-beta-lactamase in Pseudomonas aeruginosa clinical isolates from Serbia. Antimicrob Agents Chemother. 2011;55(8):3929-31. [PubMed ID: 21646490]. [PubMed Central ID: PMC3147624]. https://doi.org/10.1128/AAC.00226-11.
-
27.
Khajuria A, Praharaj AK, Kumar M, Grover N. Emergence of NDM - 1 in the clinical isolates of Pseudomonas aeruginosa in India. J Clin Diagn Res. 2013;7(7):1328-31. [PubMed ID: 23998058]. [PubMed Central ID: PMC3749628]. https://doi.org/10.7860/JCDR/2013/5509.3137.
-
28.
Carattoli A, Fortini D, Galetti R, Garcia-Fernandez A, Nardi G, Orazi D, et al. Isolation of NDM-1-producing Pseudomonas aeruginosa sequence type ST235 from a stem cell transplant patient in Italy, May 2013. Euro Surveill. 2013;18(46). [PubMed ID: 24256888]. https://doi.org/10.2807/1560-7917.es2013.18.46.20633.
-
29.
Zafer MM, Amin M, El Mahallawy H, Ashour MS, Al Agamy M. First report of NDM-1-producing Pseudomonas aeruginosa in Egypt. Int J Infect Dis. 2014;29:80-1. [PubMed ID: 25449240]. https://doi.org/10.1016/j.ijid.2014.07.008.
-
30.
Kulkova N, Babalova M, Sokolova J, Krcmery V. First report of new Delhi metallo-beta-lactamase-1-producing strains in Slovakia. Microb Drug Resist. 2015;21(1):117-20. [PubMed ID: 25343711]. https://doi.org/10.1089/mdr.2013.0162.
-
31.
Copur Cicek A, Erturk A, Ejder N, Rakici E, Kostakoglu U, Esen Yildiz I, et al. Screening of antimicrobial resistance genes and epidemiological features in hospital and community-associated carbapenem-resistant Pseudomonas aeruginosa infections. Infect Drug Resist. 2021;14:1517-26. [PubMed ID: 33907430]. [PubMed Central ID: PMC8068481]. https://doi.org/10.2147/IDR.S299742.
-
32.
Wi YM, Greenwood-Quaintance KE, Schuetz AN, Ko KS, Peck KR, Song JH, et al. Activity of ceftolozane-tazobactam against carbapenem-resistant, non-carbapenemase-producing Pseudomonas aeruginosa and associated resistance mechanisms. Antimicrob Agents Chemother. 2018;62(1). [PubMed ID: 29133568]. [PubMed Central ID: PMC5740377]. https://doi.org/10.1128/AAC.01970-17.
-
33.
Xu Y, Niu H, Hu T, Zhang L, Su S, He H, et al. High expression of metallo-beta-lactamase contributed to the resistance to carbapenem in clinical isolates of Pseudomonas aeruginosa from Baotou, China. Infect Drug Resist. 2020;13:35-43. [PubMed ID: 32021318]. [PubMed Central ID: PMC6954094]. https://doi.org/10.2147/IDR.S233987.
-
34.
Muderris T, Durmaz R, Ozdem B, Dal T, Unaldi O, Aydogan S, et al. Role of efflux pump and OprD porin expression in carbapenem resistance of Pseudomonas aeruginosa clinical isolates. J Infect Dev Ctries. 2018;12(1):1-8. [PubMed ID: 31628827]. https://doi.org/10.3855/jidc.9486.
-
35.
Bonfiglio G, Russo G, Nicoletti G. Recent developments in carbapenems. Expert Opin Investig Drugs. 2002;11(4):529-44. [PubMed ID: 11922861]. https://doi.org/10.1517/13543784.11.4.529.
-
36.
Stone GG, Bradford PA, Yates K, Newell P. In vitro activity of ceftazidime/avibactam against urinary isolates from patients in a Phase 3 clinical trial programme for the treatment of complicated urinary tract infections. J Antimicrob Chemother. 2017;72(5):1396-9. [PubMed ID: 28088768]. https://doi.org/10.1093/jac/dkw561.
-
37.
Grupper M, Sutherland C, Nicolau DP. Multicenter evaluation of ceftazidime-avibactam and ceftolozane-tazobactam inhibitory activity against meropenem-nonsusceptible Pseudomonas aeruginosa from blood, respiratory tract, and wounds. Antimicrob Agents Chemother. 2017;61(10). [PubMed ID: 28739780]. [PubMed Central ID: PMC5610483]. https://doi.org/10.1128/AAC.00875-17.
-
38.
Buehrle DJ, Shields RK, Chen L, Hao B, Press EG, Alkrouk A, et al. Evaluation of the in vitro activity of ceftazidime-avibactam and ceftolozane-tazobactam against meropenem-resistant Pseudomonas aeruginosa isolates. Antimicrob Agents Chemother. 2016;60(5):3227-31. [PubMed ID: 26976862]. [PubMed Central ID: PMC4862525]. https://doi.org/10.1128/AAC.02969-15.
-
39.
Alatoom A, Elsayed H, Lawlor K, AbdelWareth L, El-Lababidi R, Cardona L, et al. Comparison of antimicrobial activity between ceftolozane-tazobactam and ceftazidime-avibactam against multidrug-resistant isolates of Escherichia coli, Klebsiella pneumoniae, and Pseudomonas aeruginosa. Int J Infect Dis. 2017;62:39-43. [PubMed ID: 28610832]. https://doi.org/10.1016/j.ijid.2017.06.007.
-
40.
Sader HS, Castanheira M, Flamm RK. Antimicrobial activity of ceftazidime-avibactam against Gram-negative bacteria isolated from patients hospitalized with Pneumonia in U.S. medical centers, 2011 to 2015. Antimicrob Agents Chemother. 2017;61(4). [PubMed ID: 28069649]. [PubMed Central ID: PMC5365649]. https://doi.org/10.1128/AAC.02083-16.
-
41.
Pfaller MA, Shortridge D, Sader HS, Gales A, Castanheira M, Flamm RK. Ceftolozane-tazobactam activity against drug-resistant Enterobacteriaceae and Pseudomonas aeruginosa causing healthcare-associated infections in Latin America: report from an antimicrobial surveillance program (2013-2015). Braz J Infect Dis. 2017;21(6):627-37. [PubMed ID: 28941394]. [PubMed Central ID: PMC9425460]. https://doi.org/10.1016/j.bjid.2017.06.008.
-
42.
Sid Ahmed MA, Khan FA, Hadi HA, Skariah S, Sultan AA, Salam A, et al. Association of bla(VIM-2), bla(PDC-35), bla(OXA-10), bla(OXA-488) and bla(VEB-9) beta-Lactamase Genes with Resistance to Ceftazidime-Avibactam and Ceftolozane-Tazobactam in Multidrug-Resistant Pseudomonas aeruginosa. Antibiotics (Basel). 2022;11(2). [PubMed ID: 35203733]. [PubMed Central ID: PMC8868128]. https://doi.org/10.3390/antibiotics11020130.
-
43.
Lomovskaya O, Rubio-Aparicio D, Nelson K, Sun D, Tsivkovski R, Castanheira M, et al. In vitro activity of the ultrabroad-spectrum beta-lactamase inhibitor QPX7728 in combination with multiple beta-lactam antibiotics against Pseudomonas aeruginosa. Antimicrob Agents Chemother. 2021;65(6). [PubMed ID: 33782010]. [PubMed Central ID: PMC8315991]. https://doi.org/10.1128/AAC.00210-21.
-
44.
Adam MA, Elhag WI. Prevalence of metallo-beta-lactamase acquired genes among carbapenems susceptible and resistant Gram-negative clinical isolates using multiplex PCR, Khartoum hospitals, Khartoum Sudan. BMC Infect Dis. 2018;18(1):668. [PubMed ID: 30558551]. [PubMed Central ID: PMC6296134]. https://doi.org/10.1186/s12879-018-3581-z.