Abstract
Background:
Crimean-Congo hemorrhagic fever virus (CCHFV) is a highly lethal virus that causes hemorrhagic fever in humans and is endemic in many countries, including Iran. Therefore, fast, accurate, and reliable diagnosis is crucial for patient management and outbreak control.Objectives:
This study aims to optimize a TaqMan multiplex real-time RT-PCR for the rapid and specific diagnosis of CCHFV.Methods:
In this study, the L (NC_005301.3) and S (NC_005302.1) fragments were used as reference sequences for blast analysis. The L and S sequence segments of CCHFV with more than 90% identity from different areas were downloaded from the Genbank database. Primers and probes were designed based on the best-conserved regions of CCHFV L and S sequence segments. To construct the plasmid, a 1751 bp fragment from the MS2 phage that was previously amplified using cloning primers was inserted into the pET-32a plasmid. The S and L segments of the CCHFV, which were 110 bp and 135 bp, respectively, were inserted downstream of the MS2 phage sequence from HindIII to NotI. The Viral-like particles (VLPs) were produced in Escherichia coli, strain BL-21(DE3), in the presence of 1 mM Isopropyl β-D-1-thiogalactopyranoside (IPTG). The stability of VLP particles was confirmed in the presence of the ribonuclease enzyme. The fabrication of VLPs was approved by transmission electron microscopy (TEM) with negative staining (1% phosphotungstic acid). To validate the specificity of the primers and probes sequences, we compared them to the NCBI database and tested them experimentally using extracted DNA and RNA samples from healthy subjects and an infectious panel.Results:
The VLPs showed complete resistance in the presence of the ribonuclease enzyme, and the TEM results confirmed that the VLPs were correctly produced. The TaqMan multiplex real-time RT-PCR confirmed that the primers and probes were designed correctly and were completely specific to the CCHFV. The limit of detection (LOD) of the multiplex assay for the L and S genes was one copy of the VLPs per µL.Conclusions:
This TaqMan assay is reliable for amplifying CCHFV due to its design on conserved regions of the CCHFV sequences, which have minimal variability and high specificity.Keywords
Viral-Like Particle Crimean-Congo Hemorrhagic Fever Virus MS2 TEM
1. Background
Crimean-Congo hemorrhagic fever virus (CCHFV) is a zoonotic virus that belongs to the Orthonairovirus genus in the Nairoviridae family and can cause severe hemorrhagic fever with a high fatality rate (1, 2). The virus undergoes maturation and evolution in Hyalomma ticks and is transmitted to animals through tick bites in domestic animal settings (3-6). While the virus typically does not cause disease in animals except ostriches, humans can develop symptoms one to three days after being bitten by an infected tick or five to six days after contact with an infected animal (7). The disease progresses to a bleeding episode after a few days (8). Given the high mortality rate associated with CCHFV, rapid identification of patients is crucial for starting supportive therapy and controlling outbreaks (9, 10).
The CCHFV genome is a single-stranded negative-sense RNA consisting of three segments: Small (S), medium (M), and large (L). The L segment encodes RNA-dependent RNA polymerase, while the S segment encodes nucleocapsid protein (NP), which forms the main structure of the virus, and the M segment encodes envelope glycoproteins Gn (G1) and Gc (G2) (11). The virus has spread to 30 countries in Asia, Africa, parts of Europe, and the Middle East and has been identified by the World Health Organization (WHO) as a significant disease requiring investigation, diagnosis, prediction, and management (12, 13).
Several laboratory methods can be used to diagnose CCHFV infection, including enzyme-linked immunosorbent assay (ELISA), antigen detection, serum neutralization, reverse transcriptase polymerase chain reaction (RT-PCR) assay, and virus isolation by cell culture (9, 14). In the acute phase of the illness, isolation of the virus or antigen detection ELISA from the patient is also performed (15). Fatal cases rarely show significant antibody responses, while in those destined to survive, IgM and IgG antibodies are frequently detectable after about a week of illness (16, 17). Therefore, identifying the virus with molecular techniques plays a vital role in identifying people who are infected or suspected of infection (18, 19). The incubation period for CCHFV infections is commonly 3 to 7 days, but it varies depending on the type of exposure. Hemorrhagic fever typically appears 3 to 6 days post-onset of illness, with a petechial rash observable on the trunk and limbs.
Rapid laboratory diagnosis of CCHFV can be achieved through RT-PCR (20, 21). Since RT-PCR detects the virus's genetic material and can be designed to be highly specific, a probable diagnosis of CCHFV can be made without the need to culture the virus, which requires a specialized laboratory and a significant amount of time. The high sensitivity of RT-PCR means that positive results can be obtained even from samples that are negative for virus culture (15, 22). However, molecular methods require positive control, as in other diagnostic tests (23).
CCHFV is an RNA virus, and RNA is unstable in harsh conditions such as high pH and temperatures. RNase is also abundant in the environment; even minor contamination can completely degrade RNA molecules (24). Armed RNA technology allows almost any exogenous RNA of an appropriate size to be integrated downstream of the MS2 phage packaging site and non-specifically assembled into expressed MS2 phage capsid. Furthermore, in this technique, Armed RNA is stable and resistant in the presence of the ribonuclease enzyme and has natural virus-like properties (25, 26). The geographic distribution of the Crimean-Congo hemorrhagic fever virus is widespread, with genetic variations among the viruses in different areas exceeding 20 percent (18, 27). The Middle East, Iran, Pakistan, Iraq, and Turkey are areas where the virus is prevalent, and rapid detection of infection plays a critical role in preventing and controlling the disease (28).
2. Objectives
This study aims to establish a TaqMan multiplex real-time RT-PCR assay based on the highly conserved L and S segments regions of the CCHFV and to develop a positive control for CCHFV using Armed RNA technology.
3. Methods
3.1. Design Specific Primers and TaqMan Probes
The NCBI nucleotide database (www.ncbi.nlm.nih.gov/nucleotide) was used to obtain CCHFV sequences with more than 90% identity. We then aligned 239 L and 351 S segments using CCHFV and MEGA6 software. Using AlleleID7 software, we designed and validated a set of primers and TaqMan probes based on the most conserved regions of CCHFV L and S genome segments. These primers and probes have the potential to detect the virus at the lowest limit of detection (LOD) and can identify both segments simultaneously (29). We also synthesized the 135 bp and 110 bp conserved regions of the CCHFV (Figure 1), which contain the sequence for constructing a standard for TaqMan real-time PCR through Metabion company (Germany). The primers and probes used in the study are listed in Table 1.
Single-strand overlap-extension of L and S segments using the SOEing PCR method. Red: Restriction enzyme linker, Green: Restriction enzyme site, Yellow: Primers site, Blue: Probe site, Grey: Overlap DNA segment.
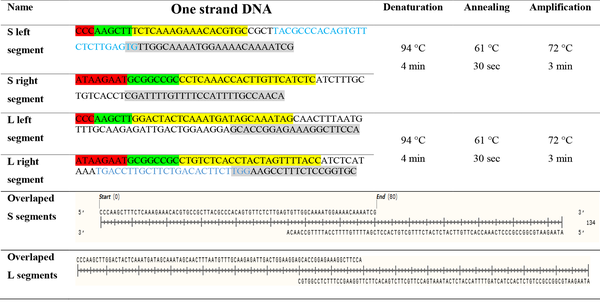
Sequence of Primers and Probes Used in the Study
Primer Name | Sequence |
---|---|
MS2 forward cloning primer | ATGGATCCCCTTTCGGGGTCCT |
MS2 reverse cloning primer | GCAAGCTTAGTTGAACTTCTTTGTTGTC |
S segment, forward primer | 3´-TCTCAAAGAAACACGTGC-5´ |
S segment, reverse primer | 3´-CYTCAAACCAYTTGTTCATCTC-5' |
S segment, probe | FAM-TACGCCCACAGTGTTCTCTTGAGTG-BHQ1 |
L segment, forward primer | 3´-GGACTACTCAAATGATAGCAAATAG-5´ |
L segment, reverse primer | 3´-CTGTCTCACCTACTAGTTTTACC-5' |
L segment, probe | FAM-TGCTCCTCCGACCAGACA-BHQ1 |
RNase P, forward primer | 3´-CTTGCTGTTTGGGCTCTC-5´ |
RNase P, reverse primer | 3´-AATCTTCATCTCCTTCTGATGG-5' |
RNase P, probe | HEX-TGCGGTGTCCACCAACTGCC-BHQ1 |
3.2. Vectors and Bacterial Strain
In this study, we used pET-32a (Novagen company, Germany) as a vector, which contains an ampicillin selection marker, a T7 promoter for potent transcription in Escherichia coli BL21 (DE3), and can be induced by isopropyl-β-d-thiogalactoside (IPTG). The IPTG-inducible promoter is inserted in the E. coli BL21 (DE3) genome. It expresses a high T7 transcription factor protein level after induction, allowing for the expression of the exogenous fragment cloned in pET-32a.
3.3. Positive Control Preparation
In this study, we utilized a plasmid that contained a 1751bp fragment of MS2 phage (maturase) previously fabricated in pET-32a (30). To join the S and L overlap DNA segments, we used SOEing PCR (Splicing Overlap Extension), a fast and effective PCR method for simultaneously joining multiple DNA fragments via designed overlap segments (31). Initially, we designed one-strand DNAs with NotI and HindIII restriction sites. Subsequently, we mixed, annealed, and extended the second strand of DNAs (as shown in Figure 1). These two-stranded DNAs were purified using Michael R. Green and Joseph Sambrook's protocol (32) and then cloned in a designed pET-32a with MS2 maturase, a coat protein gene (positions 1765 to 1787). The primer set and one-strand DNA sequences for amplifying each genome for cloning purposes are shown in Figure 1 and Table 1. Notably, MS2 was cloned in the BamHI to the HindIII site of pET-32a, and the CCHFV pET-32a, located downstream, was cloned between HindIII and NotI of the MS2 fragment.
3.4. Construction of Recombinant BL21 (DE3)/pET32a-MS2-CCHF Strain
The plasmid that was fabricated was validated using the Sanger sequencing method. Once the plasmid was confirmed, it was transformed into E. coli BL21(DE3) (Institute Pasteur, Iran) under selection conditions of ampicillin on a solid LB plate.
3.5. Expression of pET32a-MS2-CCHF
A single recombinant E. coli BL21 (DE3) colony was picked and cultured in a 5 mL LB medium, then incubated for 18 hours. Next, the culture was transferred into 1 L of LB medium containing 50 mg/L ampicillin and incubated at 37°C with 250 rpm agitation for 12 hours until the optimal cell density was reached. Then, 1 mM final concentration IPTG was added, and the culture was kept for 16 hours to allow for protein expression and viral-like particle assembly (30).
3.6. Release of Expressed Viral-like Particles from Recombinant BL21 (DE3)
The bacterial culture was precipitated by centrifugation at 12000 rpm for 10 minutes. The bacterial pellet was then suspended in a sonication buffer and disrupted by ultrasonication to release viral-like particles. Finally, 200 μL of MS2 RNA genome of phages was extracted using an RNA extraction kit based on the instruction manual (GeneAll Biotechnology, Korea).
3.7. Internal Control Preparation
This study used a fragment of RNase P in the human genome (as an internal control) to evaluate the RNA extraction from samples and the cDNA synthesis procedure. The set of primers was designed based on the exon-exon junction.
3.8. Product Stability Confirmation in the Presence of the Ribonuclease Enzyme
After sonication, the bacteria were centrifuged at 14000 rpm for 20 min, and the supernatant was collected in a 50 mL conical tube. The supernatant was treated with 100 U/mL RNaseA and 1 U/mL DNase I for 4 h at 37°C to digest plasmid and bacterial RNA, respectively. Finally, the VLPs were precipitated using Polyethylene glycol (PEG) in Tris/Saline/EDTA (TSE) buffer (10 mM Tris, pH 7.5, 1mM EDTA, and 100mM NaCl). The VLP genomes were extracted and evaluated by agarose gel electrophoresis (1%) and stained with gel-red dye. The integrity of the VLPs was confirmed by transmission electron microscopy (TEM) after staining with 1% phosphotungstic acid.
3.9. Analysis of the Specificity of the Primers and Probes
The specificity of the primers and probes was experimentally determined and confirmed by blasting them in the NCBI database. Additionally, the primers and probes were assessed experimentally for specificity using 20 blood samples as the negative control group and an infectious panel consisting of 8 samples for each virus (COVID-19, influenza A and B, B19, HBV, HCV, HIV, HTLV-1, CMV, HSV1, VZV) with three repetitions.
3.10. Taqman Real-time RT-PCR
The one-step CCHFV multiplex real-time RT-PCR was developed and validated using the Biorabit one-step real-time PCR mastermix. The S, L segments and RNase P internal control (IC) gene were checked in a multiplex reaction, while the concentration of the extracted genome was measured using a Nanodrop (Thermo Fisher, USA). The number of MS2 phage genomes was obtained by ng of RNA to calculate the copy number. To determine the limit of detection (LOD) of positive controls (VLPs), serial dilutions (10-1, 10-2, 10-3, 10-4, and 10-5 copy/mL) were prepared (30). Three multiplex reactions were repeated for segments and gene control with designed primers and probes (Table 1). The repeatability was assessed three times: Once a day and two more times on different days with different users.
3.11. Amplification of the CCHFV by TaqMan Real-time PCR Assay
The Viral-like particles containing CCHFV were analyzed using a multiplex TaqMan real-time RT-PCR assay. The monoplex and multiplex reactions were performed using the Biorabit TaqMan mastermix (4X CAPITAL™ 1-Step qRT-PCR Probe mastermix) with a final reaction volume of 20µL. The reaction mixture contained 5µL of buffer, 200 moles of each forward and reversed primer, 100 nmol of each probe (as shown in Table 1), and 5 µL of each Armored RNA (a total of 10 µL). The reaction proceeded with an RT step for 20 minutes at 50°C, followed by a denaturation step for 2 min at 95°C, and 40 cycles of 10 s at 94°C, 40 s at 60°C for annealing, amplification, and fluorescent collection, respectively.
4. Results
4.1. Viral-like Particles Confirmation
The result of VLPs confirmation using TEM with ∼30nm diameter shown in Figure 2, confirmed the successful production of MS2.
The MS2 + phage particle, containing L and S fragments, from CCHFV stained with 1% PTA and imaged by TEM
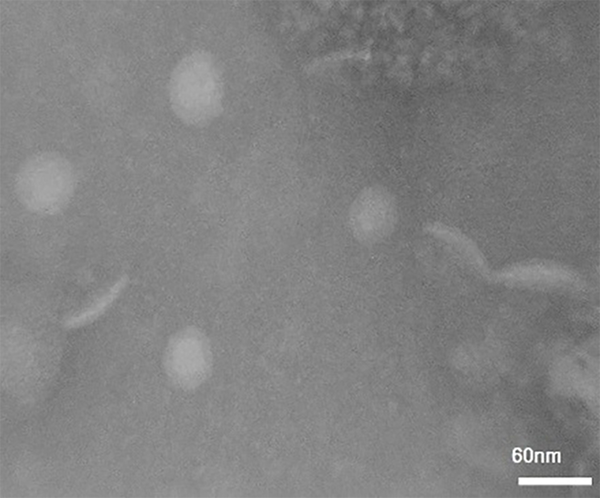
4.2. Stability of Expressed Viral-like Particles Evaluation
The Viral-like particles were analyzed by 1% gel agarose electrophoresis after treatment with the ribonuclease enzyme, and the results are shown in Figure 3. The gel was stained with GelRed and demonstrated that the Viral-like particles were completely resistant in the presence of the ribonuclease enzyme.
Resistance of MS2 + CCHF viral-like particles in the presence of the ribonuclease enzyme. Lane A: Ladder 100bp (BIORON Gmbh), Lanes B and C: Mobility of VLPs in 1% gel agarose between 800 and 900bp
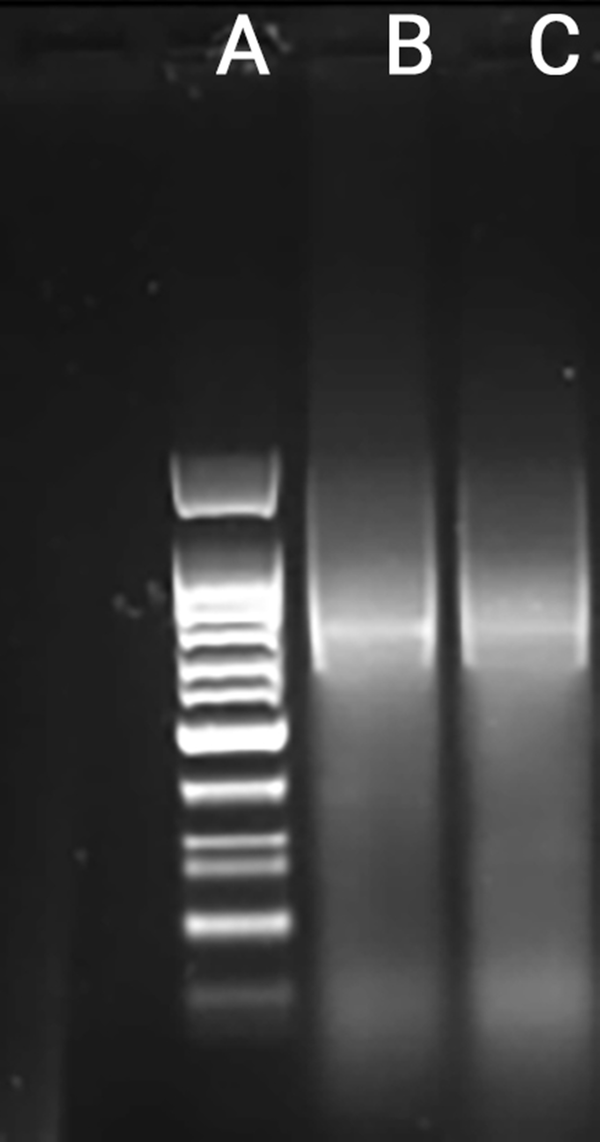
4.3. PCR Amplification of MS2 + CCHFV Viral-like Particles
The PCR products were analyzed by 1% agarose gel electrophoresis and stained with gel red (Figure 4). The results showed that 135bp and 110bp fragments of CCHFV were inserted downstream of the MS2 packaging sequence and successfully packaged in the viral-like particles. Additionally, the particles were amplified correctly using specific primers.
Agarose gel electrophoresis of the RT-PCR amplification products of MS2 + CCHFV, a VLP, using 1% agarose gel
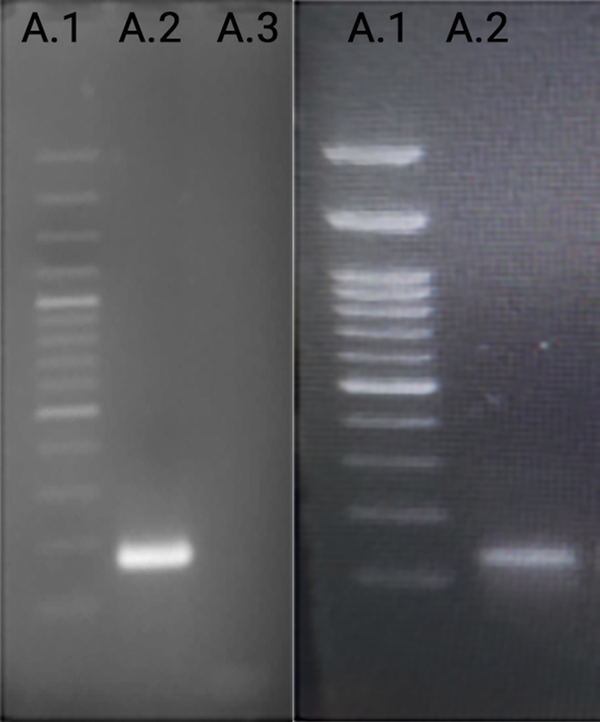
4.4. Analytical Specificity of Primers and Probes
The analytical specificity of the assay was evaluated with a concentration of 10μL. The results were negative for 20 healthy subjects and a panel of infectious agents (8 samples for each virus), including COVID-19, Influenza A and B, B19, HBV, HCV, HIV, HTLV-1, CMV, HSV1, and VZV. The tests were repeated three times and were performed at the AJA University of Medical Sciences diagnostic laboratory. The results showed a clinical and analytical specificity of 100% for CCHFV.
4.5. Reproducibility and LOD
The reproducibility and limit of detection (LOD) of the multiplex RT-TaqMan real-time PCR were evaluated in triplicate at each concentration for intra-assay and inter-assay variability. The results were as follows: The reproducibility of the assay was assessed by conducting three replicates for each reaction. The LOD for CCHFV was determined to be 102 copies/mL for the L segment, 102 copies/mL for the S segment, and 101 copies/mL for the multiplex detection of both L and S segments. Serial dilutions of VLP RNA samples were prepared and analyzed by real-time RT-PCR, confirming the specificity of the L and S segment primers for CCHFV.
5. Discussion
Real-time PCR is the most common method for diagnosing many viruses, including CCHFV. real-time RT-PCRs are mainly designed to identify the highly conserved S region in the CCHFV genome. In one study, SybrGreen was used to obtain 100% specificity and five copies/μL sensitivity for CCHFV diagnosis (22). Another research study based on this method was evaluated on Balkan CCHFV strains, which was found to be more accurate than nested PCR (33). Zahraei et al. also obtained 100% specificity and less than 20 copies/reaction sensitivity using SybrGreen (34). However, in a study by Kamboj et al., although they obtained a LOD of 5 copies/reaction due to 3' end primer mismatches, the real-time RT-PCR could not identify all 18 clinical samples (35). On the other hand, another investigation on 6 CCHFV genotypes synthetic RNAs showed that LOD for Afghanistan, Kosovo, DR Congo, and Greece are two copies/μL; however, for Senegal and Mauritania, it was 200 copies/μL (36).
In several studies with probes, specificity was 100%, and sensitivity was high (37, 38). In a study conducted in Iran on 37 suspected samples, 15 were positive, and the results were confirmed by sequencing. Also, a sensitivity of 10 copies/μL (or lower) was obtained (39). However, in this investigation, multiplex real-time RT-PCR results revealed that the PCR reaction was correctly optimized, and the primers and probes were appropriately designed. These primers and probes were designed based on highly conserved regions of the L and S sequence of the CCHFV, which is different from other studies that used primers designed only on the S sequence of the CCHFV (40, 41).
According to bioinformatical (www.ncbi.nlm.nih.gov/nucleotide) and experimental analysis on healthy controls, the primers and probes have 100% specificity for CCHFV (the specificity has been evaluated based on healthy subjects and a panel of infectious agents). By designing multiplex real-time RT-PCR with FAM reporters for both probes, viral genomes could be detected at the lowest LOD (the same as human immunodeficiency virus (HIV) diagnostic multiplex real-time RT-PCR). The SOEing-PCR (Splicing Overlap Extension) method was used to prepare the control. The findings indicate that it is possible to prepare a positive control for many infectious agents at a low cost. Based on this investigation, it is the first study in which CCHFV was optimized with Armored RNA technology, and two target sites (S and L segments) were employed for genome amplification. Moreover, this method can detect the virus with the lowest LOD (1 copy/μL). It is suggested to validate the findings of this study on clinical samples to ensure the efficiency of this test.
5.1. Conclusions
Rapid diagnosis of infected patients is crucial for managing and controlling viral diseases. This TaqMan assay reliably amplifies and diagnoses CCHFV due to the highly conserved regions of the L and S segments with minimal variability, thereby significantly reducing the probability of mismatches affecting this multiplex real-time RT-PCR. Using FAM for both probes in this TaqMan multiplex real-time RT-PCR, which has the lowest LOD and highest specificity, makes it an excellent choice for precise, specific, and repeatable CCHFV detection. Therefore, we recommend this assay for the accurate and rapid detection of CCHFV.
Acknowledgements
References
-
1.
Cevik MA, Erbay A, Bodur H, Gulderen E, Bastug A, Kubar A, et al. Clinical and laboratory features of Crimean-Congo hemorrhagic fever: predictors of fatality. Int J Infect Dis. 2008;12(4):374-9. [PubMed ID: 18063402]. https://doi.org/10.1016/j.ijid.2007.09.010.
-
2.
Garrison AR, Alkhovsky SV, Avšič-Županc T, Bente DA, Bergeron É, Burt F, et al. ICTV virus taxonomy profile: Nairoviridae. J Gen Virol. 2020;101(8):798-9. [PubMed ID: 32840475]. [PubMed Central ID: PMC7641396]. https://doi.org/10.1099/jgv.0.001485.
-
3.
Burt FJ, Spencer DC, Leman PA, Patterson B, Swanepoel R. Investigation of tick-borne viruses as pathogens of humans in South Africa and evidence of Dugbe virus infection in a patient with prolonged thrombocytopenia. Epidemiol Infect. 1996;116(3):353-61. [PubMed ID: 8666081]. [PubMed Central ID: PMC2271429]. https://doi.org/10.1017/s0950268800052687.
-
4.
Chinikar S, Persson SM, Johansson M, Bladh L, Goya M, Houshmand B, et al. Genetic analysis of Crimean-congo hemorrhagic fever virus in Iran. J Med Virol. 2004;73(3):404-11. [PubMed ID: 15170636]. https://doi.org/10.1002/jmv.20106.
-
5.
Logan TM, Linthicum KJ, Bailey CL, Watts DM, Moulton JR. Experimental transmission of Crimean-Congo hemorrhagic fever virus by Hyalomma truncatum Koch. Am J Trop Med Hyg. 1989;40(2):207-12. [PubMed ID: 2493203]. https://doi.org/10.4269/ajtmh.1989.40.207.
-
6.
Hoogstraal H. The epidemiology of tick-borne Crimean-Congo hemorrhagic fever in Asia, Europe, and Africa. J Med Entomol. 1979;15(4):307-417. [PubMed ID: 113533]. https://doi.org/10.1093/jmedent/15.4.307.
-
7.
Meriç Koç M, Willke A. [A case of Crimean-Congo hemorrhagic fever with long incubation period in Kocaeli, Turkey]. Mikrobiyol Bul. 2012;46(1):129-33. Turkey. [PubMed ID: 22399182].
-
8.
Butenko AM, Karganova GG. Crimean-Congo hemorrhagic fever in Russia and other countries of the former Soviet Union. Crimean-Congo hemorrhagic fever. Springer; 2007. p. 99-114. https://doi.org/10.1007/978-1-4020-6106-6_9.
-
9.
Ergonul O. Crimean-Congo hemorrhagic fever virus: new outbreaks, new discoveries. Curr Opin Virol. 2012;2(2):215-20. [PubMed ID: 22482717]. https://doi.org/10.1016/j.coviro.2012.03.001.
-
10.
Raabe VN. Diagnostic testing for Crimean-Congo hemorrhagic fever. J Clin Microbiol. 2020;58(4). [PubMed ID: 32024724]. [PubMed Central ID: PMC7098759]. https://doi.org/10.1128/JCM.01580-19.
-
11.
Zivcec M, Scholte FE, Spiropoulou CF, Spengler JR, Bergeron E. Molecular insights into Crimean-Congo hemorrhagic fever virus. Viruses. 2016;8(4):106. [PubMed ID: 27110812]. [PubMed Central ID: PMC4848600]. https://doi.org/10.3390/v8040106.
-
12.
Shahhosseini N, Wong G, Babuadze G, Camp JV, Ergonul O, Kobinger GP, et al. Crimean-Congo hemorrhagic fever virus in Asia, Africa and Europe. Microorganisms. 2021;9(9). [PubMed ID: 34576803]. [PubMed Central ID: PMC8471816]. https://doi.org/10.3390/microorganisms9091907.
-
13.
Jonkmans N, D'Acremont V, Flahault A. Scoping future outbreaks: a scoping review on the outbreak prediction of the WHO Blueprint list of priority diseases. BMJ Glob Health. 2021;6(9). [PubMed ID: 34531189]. [PubMed Central ID: PMC8449939]. https://doi.org/10.1136/bmjgh-2021-006623.
-
14.
Borio L, Inglesby T, Peters CJ, Schmaljohn AL, Hughes JM, Jahrling PB, et al. Hemorrhagic fever viruses as biological weapons: medical and public health management. JAMA. 2002;287(18):2391-405. [PubMed ID: 11988060]. https://doi.org/10.1001/jama.287.18.2391.
-
15.
Schwarz TF, Nsanze H, Longson M, Nitschko H, Gilch S, Shurie H, et al. Polymerase chain reaction for diagnosis and identification of distinct variants of Crimean-Congo hemorrhagic fever virus in the United Arab Emirates. Am J Trop Med Hyg. 1996;55(2):190-6. [PubMed ID: 8780459]. https://doi.org/10.4269/ajtmh.1996.55.190.
-
16.
Charrel RN, Attoui H, Butenko AM, Clegg JC, Deubel V, Frolova TV, et al. Tick-borne virus diseases of human interest in Europe. Clin Microbiol Infect. 2004;10(12):1040-55. [PubMed ID: 15606630]. https://doi.org/10.1111/j.1469-0691.2004.01022.x.
-
17.
Saijo M, Tang Q, Shimayi B, Han L, Zhang Y, Asiguma M, et al. Recombinant nucleoprotein-based serological diagnosis of Crimean-Congo hemorrhagic fever virus infections. J Med Virol. 2005;75(2):295-9. [PubMed ID: 15602720]. https://doi.org/10.1002/jmv.20270.
-
18.
Whitehouse CA. Crimean-Congo hemorrhagic fever. Antiviral Res. 2004;64(3):145-60. [PubMed ID: 15550268]. https://doi.org/10.1016/j.antiviral.2004.08.001.
-
19.
Zeller H. Laboratory diagnosis of Crimean-Congo hemorrhagic fever. Crimean-Congo hemorrhagic fever. 2007. p. 233-43. https://doi.org/10.1007/978-1-4020-6106-6_18.
-
20.
Drosten C, Kummerer BM, Schmitz H, Gunther S. Molecular diagnostics of viral hemorrhagic fevers. Antiviral Res. 2003;57(1-2):61-87. [PubMed ID: 12615304]. https://doi.org/10.1016/s0166-3542(02)00201-2.
-
21.
Wolfel R, Paweska JT, Petersen N, Grobbelaar AA, Leman PA, Hewson R, et al. Virus detection and monitoring of viral load in Crimean-Congo hemorrhagic fever virus patients. Emerg Infect Dis. 2007;13(7):1097-100. [PubMed ID: 18214191]. [PubMed Central ID: PMC2878241]. https://doi.org/10.3201/eid1307.070068.
-
22.
Drosten C, Gottig S, Schilling S, Asper M, Panning M, Schmitz H, et al. Rapid detection and quantification of RNA of Ebola and Marburg viruses, Lassa virus, Crimean-Congo hemorrhagic fever virus, Rift Valley fever virus, dengue virus, and yellow fever virus by real-time reverse transcription-PCR. J Clin Microbiol. 2002;40(7):2323-30. [PubMed ID: 12089242]. [PubMed Central ID: PMC120575]. https://doi.org/10.1128/JCM.40.7.2323-2330.2002.
-
23.
Bustin SA, Benes V, Garson JA, Hellemans J, Huggett J, Kubista M, et al. The MIQE guidelines: minimum information for publication of quantitative real-time PCR experiments. Clin Chem. 2009;55(4):611-22. [PubMed ID: 19246619]. https://doi.org/10.1373/clinchem.2008.112797.
-
24.
Bernhardt HS. The RNA world hypothesis: the worst theory of the early evolution of life (except for all the others)(a). Biol Direct. 2012;7:23. [PubMed ID: 22793875]. [PubMed Central ID: PMC3495036]. https://doi.org/10.1186/1745-6150-7-23.
-
25.
Zhan S, Li J, Xu R, Wang L, Zhang K, Zhang R. Armored long RNA controls or standards for branched DNA assay for detection of human immunodeficiency virus type 1. J Clin Microbiol. 2009;47(8):2571-6. [PubMed ID: 19494069]. [PubMed Central ID: PMC2725685]. https://doi.org/10.1128/JCM.00232-09.
-
26.
Mikel P, Vasickova P, Tesarik R, Malenovska H, Kulich P, Vesely T, et al. Preparation of MS2 phage-Like particles and their use as potential process control viruses for detection and quantification of enteric RNA viruses in different matrices. Front Microbiol. 2016;7:1911. [PubMed ID: 28133456]. [PubMed Central ID: PMC5234545]. https://doi.org/10.3389/fmicb.2016.01911.
-
27.
Ergonul O. Crimean-Congo haemorrhagic fever. Lancet Infect Dis. 2006;6(4):203-14. [PubMed ID: 16554245]. [PubMed Central ID: PMC7185836]. https://doi.org/10.1016/S1473-3099(06)70435-2.
-
28.
Fereidouni M, Apanaskevich DA, Pecor DB, Pshenichnaya NY, Abuova GN, Tishkova FH, et al. Crimean-Congo hemorrhagic fever virus in Central, Eastern, and South-eastern Asia. Virol Sin. 2023. [PubMed ID: 36669701]. https://doi.org/10.1016/j.virs.2023.01.001.
-
29.
Jerome KR. Lennette's laboratory diagnosis of viral infections. CRC Press; 2016. https://doi.org/10.3109/9781420084962.
-
30.
Gholami M, Ravanshad M, Baesi K, Samiee SM, Hosseini Rozbahani N, Mohraz M. Preparation and evaluation of ribonuclease-resistant viral HIV RNA standards based on armored RNA technology. Iran Biomed J. 2018;22(6):394-400. [PubMed ID: 29776310]. [PubMed Central ID: PMC6305816]. https://doi.org/10.29252/.22.6.394.
-
31.
Thornton JA. Splicing by overlap extension PCR to obtain hybrid DNA products. Methods Mol Biol. 2016;1373:43-9. [PubMed ID: 25646606]. https://doi.org/10.1007/7651_2014_182.
-
32.
Green MR, Sambrook J. Precipitation of DNA with ethanol. Cold Spring Harb Protoc. 2016;2016(12). [PubMed ID: 27934690]. https://doi.org/10.1101/pdb.prot093377.
-
33.
Duh D, Saksida A, Petrovec M, Dedushaj I, Avsic-Zupanc T. Novel one-step real-time RT-PCR assay for rapid and specific diagnosis of Crimean-Congo hemorrhagic fever encountered in the Balkans. J Virol Methods. 2006;133(2):175-9. [PubMed ID: 16343650]. https://doi.org/10.1016/j.jviromet.2005.11.006.
-
34.
Zahraei B, Hashemzadeh MS, Najarasl M, Zahiriyeganeh S, Tat M, Metanat M, et al. Novel, in-house, SYBR green based one-step rRT-PCR: Rapid and accurate diagnosis of Crimean-Congo hemorrhagic fever virus in suspected patients from Iran. Jundishapur J Microbiol. 2016;9(1). https://doi.org/10.5812/jjm.29246.
-
35.
Kamboj A, Pateriya AK, Mishra A, Ranaware P, Kulkarni DD, Raut AA. Novel molecular beacon probe-based real-time RT-PCR assay for diagnosis of Crimean-Congo hemorrhagic fever encountered in India. Biomed Res Int. 2014;2014:496219. [PubMed ID: 24877102]. [PubMed Central ID: PMC4022296]. https://doi.org/10.1155/2014/496219.
-
36.
Sas MA, Vina-Rodriguez A, Mertens M, Eiden M, Emmerich P, Chaintoutis SC, et al. A one-step multiplex real-time RT-PCR for the universal detection of all currently known CCHFV genotypes. J Virol Methods. 2018;255:38-43. [PubMed ID: 29408661]. https://doi.org/10.1016/j.jviromet.2018.01.013.
-
37.
Ibrahim SM, Aitichou M, Hardick J, Blow J, O'Guinn ML, Schmaljohn C. Detection of Crimean-Congo hemorrhagic fever, Hanta, and sandfly fever viruses by real-time RT-PCR. Methods Mol Biol. 2011;665:357-68. [PubMed ID: 21116810]. https://doi.org/10.1007/978-1-60761-817-1_19.
-
38.
Jaaskelainen AJ, Kallio-Kokko H, Ozkul A, Bodur H, Korukruoglu G, Mousavi M, et al. Development and evaluation of a real-time RT-qPCR for detection of Crimean-Congo hemorrhagic fever virus representing different genotypes. Vector Borne Zoonotic Dis. 2014;14(12):870-2. [PubMed ID: 25514124]. [PubMed Central ID: PMC4270107]. https://doi.org/10.1089/vbz.2014.1577.
-
39.
Sharti M, Hashemzadeh M, Dorostkar R. Development of quantitative real-time RT-PCR assay for detection and viral load determination of Crimean-Congo Hemorrhagic Fever (CCHF) virus. Rom J Mil Med. 2019;122(3):69-75. https://doi.org/10.55453/rjmm.2019.122.3.10.
-
40.
Koehler JW, Delp KL, Hall AT, Olschner SP, Kearney BJ, Garrison AR, et al. Sequence optimized Real-Time reverse transcription polymerase chain reaction assay for detection of Crimean-Congo hemorrhagic fever virus. Am J Trop Med Hyg. 2018;98(1):211-5. [PubMed ID: 29165231]. [PubMed Central ID: PMC5928694]. https://doi.org/10.4269/ajtmh.17-0165.
-
41.
Yapar M, Aydogan H, Pahsa A, Besirbellioglu BA, Bodur H, Basustaoglu AC, et al. Rapid and quantitative detection of Crimean-Congo hemorrhagic fever virus by one-step real-time reverse transcriptase-PCR. Jpn J Infect Dis. 2005;58(6):358-62. [PubMed ID: 16377867].