Abstract
Background:
The composition of lung tissue microorganisms in patients with different tissue types of lung cancer has not yet been determined. Previous studies have shown changes in the composition of pulmonary microbial flora in patients with lung cancer.Objectives:
This study aimed to investigate the differences and correlations in the microbial flora of pulmonary tissue among different histological types of lung cancer.Methods:
Samples of tumor and normal lung tissue from 29 patients with early-stage lung cancer were collected. The samples were sequenced using Illumina HiSeq high throughput sequencing technology for 16S rDNA in the V4 region of the bacteria. Also, their microbiological characteristics were detected, and bioinformatics analysis was performed.Results:
The results of microbial abundance analysis in the lung tissue of patients with lung cancer showed that the bacterial colony composition of the two tissues was similar, with Proteobacteria, Firmicutes, Anomalococcus, Bacteroides, and Actinobacteria predominating. Analysis of microbial diversity found no difference in α diversity and β diversity between normal lung tissue and tumor tissue. When analyzing patients with adenocarcinoma, the abundance of Micrococcales and Blastomonas was significantly higher in tumor tissue than in normal lung tissue.Conclusions:
The composition of microbial flora in different parts of lung tissue of early-stage lung cancer patients is consistent, but the dominant flora varies among different histological types of lung cancer.Keywords
Pulmonary Cancer Early Stage Microbial Flora Biodiversity High Throughput Sequencing
1. Background
Due to the limitations of microbial cultivation methods, lung tissue was considered a sterile environment before. The emergence and development of high-throughput sequencing technology changed this situation. Currently, 16SrRNA sequencing can find many microbial floras in normal lung tissue, which significantly changes with the change in human physiological and pathological conditions (1). Research suggests a high correlation between the microbial community in healthy lung tissue and the oropharynx (2). The relationship between lung diseases and microbial communities has become a hot topic in current research on respiratory diseases. Previous studies have shown a correlation between microbial communities and benign lung diseases such as asthma (3), chronic obstructive pulmonary disease (4), and pulmonary cystic fibrosis (5). However, there is limited research on the relationship between microbial communities and lung cancer.
Lung cancer as a malignant tumor has the highest mortality rate (6). The research on the pathogenesis of lung cancer is unclear, but it is believed that it may be related to air pollution, chronic infections, and smoking. In recent years, continuous research (7, 8) has confirmed a significant change in the microbial community in the lungs of lung cancer patients compared to healthy individuals. More and more evidence suggests that the occurrence and development of lung cancer may be closely related to this change. Polymorphic microbiomes have recently been added as one of the four new "hallmarks" of cancer (9). However, due to the diverse types of research samples, research results are often inconsistent, and even contradictory conclusions may be drawn.
2. Objectives
The aim was to clarify the changes in the composition of microbial communities in lung tissue of lung cancer patients, further explore the correlation between clinical pathological characteristics and gene sequencing results, screen clinically meaningful microbial biomarkers, and provide a research basis for lung cancer's occurrence and development mechanism.
3. Methods
3.1. Research Subjects
The lung cancer patients admitted to our thoracic surgery were selected from January 2021 to July 2022. The inclusion criteria were being 41 to 78 years old, having no surgical contraindications, undergoing single-hole thoracoscopic lobectomy, being at stage I of TNM, having no antibiotic treatment one week before surgery, having complete clinical data, and giving informed consent with this study. The exclusion criteria were being < 41 or > 78 years old, being complicated with bronchial asthma, pulmonary tuberculosis, obstructive pneumonia, chronic obstructive pulmonary disease (COPD), diabetes, and other diseases affecting the characteristics of pulmonary microbial flora, having an unstandardized sterile operation or contaminated specimen, and missing clinical data.
3.2. Main Reagents and Instruments
We employed Qubit dsDNA HS Assay Kit (Life Technologies), Library Quant Kit Illumina GA Revised Primers SYBR Fast Universal (KAPA), Axigen Gel Extraction Kit (250) (Axigen), Agency AMPure XP 60 mL Kit (Beckman Coulter), Usage High Identity PCR Master Mix (NEB), HiSeq 3000/4000 SBS Kit (300 cycles) (Illumina), NanoDrop 2000C (Thermo Company), fluorescent quantitative PCR (Eppendorf Company), Qubit 2.0 (Life Technologies Company), gel imaging system (Bio-Rad Company), nucleic acid analyzer (Agilent Company), and high-throughput sequencing (Illumina Company). High-throughput sequencing was completed by Hangzhou Guhe Information Technology Co., Ltd.
3.3. Sample Collection
A piece of soybean-sized lung tissue from the surgical specimen was cut within 20 minutes of isolation (sterile operation), froze it in liquid nitrogen, and stored it in a -80°C refrigerator. The selected tissues are tumor tissue (C) and peripheral normal lung tissue (L) located more than 5 cm from the tumor site. Normal lung tissue and tumor tissue were obtained from 29 patients. All procedures were meant not to affect the postoperative pathological diagnosis of the patient.
3.4. DNA Extraction and 16S rDNA Gene Amplicon Sequencing
The approximately 30 mg of fresh frozen lung tissue samples were prepared and nucleic acid extraction or purification reagents (Hangzhou Gu He: GHFDE100) were used for DNA extraction. Total microbial genomic DNA was extracted from the fresh frozen lung tissue samples. The primers for PCR amplification of the V4 hypervariable region of 16S rDNA were forward primer 515F (5’-GTGCCAGCMGCCGCGGTAA-3’) and reverse primer 806R (5’-GGACTACHVGGGTWTCTAAT-3’). A total of 50 uL (25 μL high fidelity DNA polymerase, 3 μL(10 uM) of each forward and reverse primers, 10 μL DNA template, 3 μL DMSO、and 6 μL ddH2O was used as the PCR (polymerase chain reaction) reaction system. The amplification reaction conditions were as follows: Pre-denaturation at 98°C for 30 s, followed by 25 cycles of denaturation at 98°C for 15 s, annealing at 58°C for 15 s, and extension at 72°C for 15 s. The final extension was done at 72°C for 1 min. The PCR products were purified using AMPure XP Beads (Beckman Coulter, Indianapolis, IN), and the library was quantified using Qubit. Subsequently, sequencing was performed using the Illumina NovaSeq platform. The original data NMDC40029556 are stored at the National Microbial Science Data Center (NMDC).
3.5. Further Processing of Sequencing Data and Bioinformatics Analysis
Each sample data was split from the original data based on primer and barcode sequences. After reading splicing and filtering, we performed an operational classification unit (OTU) clustering analysis with 97% similarity, annotated species, and analyzed abundance by calculating the value of chao1 α analysis of alpha diversity. Using Bray Curtis distance and plotting principal coordinates analysis (PCoA) graphs, the microbial community structure of different samples was analyzed β diversity analysis. Microbial abundance analysis of α diversity and β diversity was done in the microbial community data analysis platform (https://www.microbiomeanalyst.ca/MicrobiomeAnalyst).
3.6. Statistical Analysis
SPSS 26.0 software was used to perform differential analysis on the data. Continuous variables are presented as mean ± standard deviation and analyzed by t-test. Categorical variables are presented as percentages and analyzed by the chi-square test or Fisher's exact test. P < 0.05 was considered statistically significant.
4. Results
4.1. General Information
According to the inclusion and exclusion criteria, a total of 29 lung cancer patients were included in this study, with an average age of 65.31 ± 8.64 years, including 19 males and 10 females. Among all patients, 7 had a smoking history, 22 had adenocarcinoma, and 7 had squamous cell carcinoma as pathological types. All patients were treated for the first time. Table 1 shows specific information on the patients.
Clinical and Pathologic Characteristics of Enrolled Patients
Feature | No. (%) |
---|---|
Gender | |
Male | 19 (65.5) |
Female | 10 (34.5) |
Age (y) | |
≥ 60 | 25 (86.2) |
< 60 | 4 (13.8) |
Smoking history | |
Yes | 7 (14.1) |
No | 22 (75.9) |
Classification | |
AD | 22 (75.9) |
SCC | 7 (17.2) |
4.2. Analysis of the Species Composition and Abundance of Microbial Communities in Normal Lung Tissue and Tumor Tissue
Sequencing was performed on 29 samples to analyze the bacterial community composition of normal lung tissue (L) and tumor tissue (C). Figure 1 shows that the composition of bacterial colonies in the two tissues was similar, mainly composed of Proteobacteria, Firmicutes, Deinococota, Bacteroidota, and Actinobacteriota at the phylum level. This indicates no significant change in the overall composition of bacterial colonies at the phylum level during the occurrence and development of lung cancer. However, compared to normal lung tissue, the abundance of Proteus decreased in tumor tissue (47.2% vs. 54.1%), the abundance of Actinobacillus (8.3% vs. 5.0%) and abnormal cocci (15.8% vs. 11.5%) increased, while the abundance of Firmicutes (18.9% vs. 19.2%) and Bacteroides (8.5% vs. 8.9%) changed slightly.
Community abundance percentage at the phylum level (A); order level (B); and genus level (C)
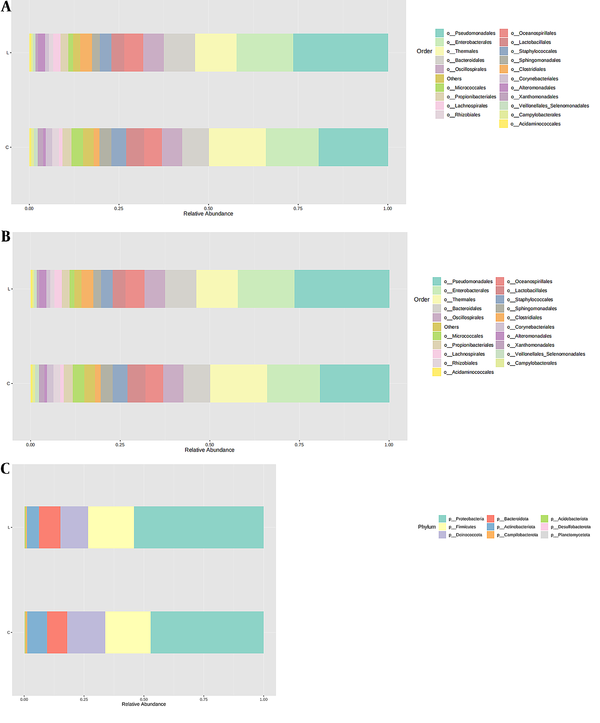
The top 20 microbial populations at the order level included Pseudomonas, Enterobacteriaceae, Thermales, Bacteroidales, Oscillospirales, Oceanospirillales, Lactobacillales, Clostridiales, Staphylococcales, Sphingomonadales, Propionibacterials, Lachnospirillales, Alternationales, Micrococcaceae, Rhizobiales, Corynebacteria, Veillonellales, Xanthomonadales, Acidaminococcales, and Campylobacterales. The top 20 microbial populations at the genus level included Pseudomonas, Meiothermus, Enterobacteriaceae, Halomonas, Staphylococcus, Enterococcus, Clostridium, Sphingomonas, Propionibacterium, Faecalibacterium, Shewanella, Prevotella, Acinetobacter, Escherichia, Shigella, Brevibacterium, Enhydrobacterium, Stenotrophomonas, and Blastomonas.
4.3. Analysis of Microbial Diversity in Normal Lung Tissue and Tumor Tissue
The diversity of microorganisms in the human body can be described by their richness and evenness, namely α diversity and β diversity. To analyze the differences in OTU composition between normal lung tissue and tumor tissue, we utilized the Chao1 index and principal coordinate analysis. Through a t-test on the Chao1 index of the microbial community in two tissues, it was found that there was no significant difference in microbial diversity between the two tissues, as shown in Figure 2 (P-value: 0.46745; (t-test) statistics: -0.73264). Figure 3 is a PCoA constructed based on the Bray-Curtis distance matrix. According to the similarity of sample composition reflected by the distance of flora in two-dimensional coordinates, the difference between samples was calculated by multivariate analysis of variance (PERMANOVA). There was no obvious difference between the two groups of tissue samples (F-value: 1.0419; R-squared: 0.021245; P-value: 0.377). This indicates no significant difference in the OTU composition of the bacterial community between normal lung tissue and tumor tissue.
The difference in the Chao1 index
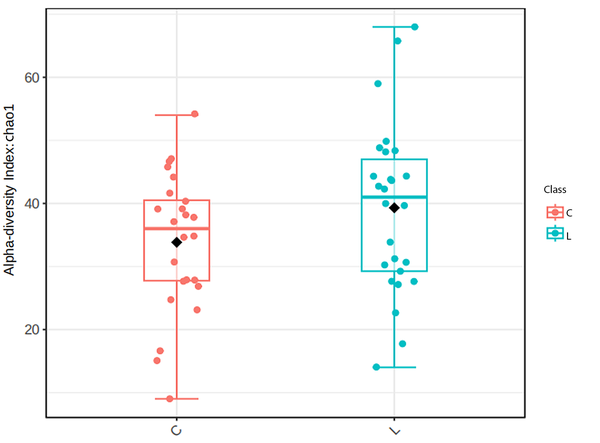
Principal coordinates analysis (PCoA) of tissue microbiota
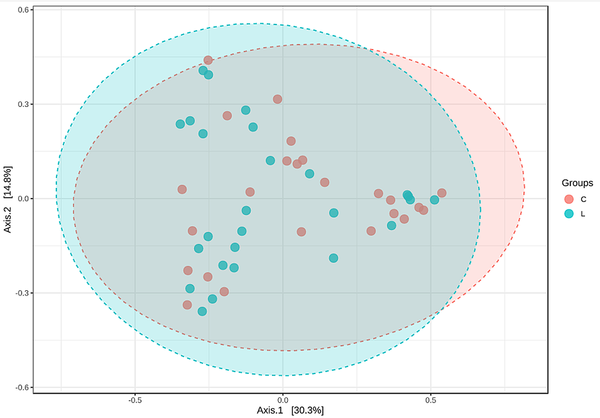
4.4. Differential Species of Bacterial Communities Between Lung Adenocarcinoma Tissue and Normal Lung Tissue
Heat tree analysis utilizes a hierarchical classification structure to quantitatively describe the classification differences between microbial communities. This is done using the median abundance and statistically analyzing the differences with a non-parametric Wilcoxon rank sum test. The goal is to identify the distinct species present in each community. Using heat tree analysis, we found no difference between normal lung tissue and tumor tissue flora. However, when analyzing patients with adenocarcinoma, we found that the abundance of Micrococcaceae and Blastomonas in tumor tissue was significantly higher than that in normal lung tissue (P values = 0.02 and 0.003, respectively), as shown in Figure 4.
Heat tree of tissue microbial flora
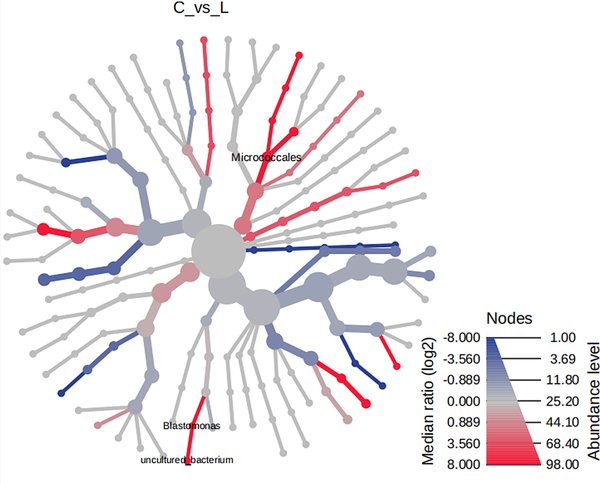
5. Discussion
Because of the communication with the outside world through the respiratory tract, the pulmonary microbial flora is in constant renewal and replacement. However, most bacterial microorganisms belong to Bacteroides, Firmicutes, Proteobacteria, and Actinomycetes (10, 11), and the main bacterial flora in the lungs of healthy people is Praetoria, Streptococcus, Neisseria, Haemophilus, and Fusobacterium (12, 13). Yu et al. (14) collected lung tissue of lung cancer patients, including tumor and non-tumor sites, and completed gene sequencing of 165 cases. The results showed significant differences in microbial flora between the lung and other parts of the human body, such as skin, mouth, nose, vagina, and intestinal tract. Proteus was the most abundant category (60%). This study also found that most of the microbial flora in lung tissue belonged to the above four phyla. Proteus was the main phyla in both normal lung tissue and tumor tissue, but it is different from previous reports at the genus level, which may be related to the individual differences in the pulmonary microbial flora.
Previous studies have found changes in the biodiversity of microbial communities in the lung tissue of lung cancer patients, but the conclusions of different studies differ. Greathouse et al. (15) studied 143 lung cancer cases and sequenced lung tissue samples from the tumor site and adjacent areas. The results showed that compared to tumor tissue, the α diversity of the microbial community in normal lung tissue was reduced. Liu et al. (8) collected samples from cancerous and contralateral non-cancerous sites and studied 24 lung cancer patients and 18 non-lung cancer patients for sequencing. It was found that compared to normal tissue, the α diversity of the microbial community in tumor tissue was decreased. Laroumagne et al. also studied paired samples of cancerous lung tissue and contralateral non-cancerous sites from lung cancer patients, showing a significant decrease in microbial diversity in lung cancer tissue (16). Our study found that there is no significant difference in α diversity or β diversity of the microbial community in tumor tissue and normal lung tissue.
More and more evidence (17, 18) suggests that human tissues may experience persistent chronic inflammation due to microbial imbalance, which may be closely related to the occurrence and development of cancer. The persistence of inflammation can continuously stimulate normal cells, causing irreversible damage to them, ultimately leading to cancer in the body's tissues. It is generally believed that microbial communities produce pro-inflammatory factors in lung tissue, which cause chronic inflammation by stimulating the respiratory epithelium. The continuous inflammatory response leads to abnormal proliferation of epithelial cells, ultimately inducing cell transformation and forming malignant tumors (19).
Previous studies have shown that due to the combined effects of genetic and environmental risk factors, lung cancer cells can grow and proliferate uncontrollably in the human body. Recently, there has been continuous evidence that microbial communities may play a certain role (13, 20), and microorganisms and their metabolites may disrupt the normal cell cycle through multiple pathways, leading to upregulation or downregulation of various signaling pathways within the cell. This, in turn, causes cells to lose control of their own proliferation and lead to cancer transformation (21). Sun et al. (22) found alterations in the microbial community of patients with lung cancer, whose diversity might depend on the site and pathology. This study found that Micrococcaceae and Blastomonas flora abundance was significantly higher in lung adenocarcinoma tumor tissues than in normal tissues. This suggests that bacterial metabolites may play a role in the occurrence and development of adenocarcinoma. However, further research with a larger sample size is needed to confirm these differences in various tissues and to investigate the underlying mechanisms of lung cancer development (23).
5.1. Conclusions
Through high-throughput sequencing of 29 surgical specimens from patients with early-stage lung cancer, we found the major microbial components in tumor and normal lung tissue. This study found differences in lung microbiota among different histological types of lung cancer, providing a basis for studying the mechanism of lung cancer occurrence and development.
References
-
1.
Baranova E, Druzhinin V, Matskova L, Demenkov P, Volobaev V, Minina V, et al. Sputum microbiome composition in patients with squamous cell lung carcinoma. Life (Basel). 2022;12(9):1365. [PubMed ID: 36143401]. [PubMed Central ID: PMC9501211]. https://doi.org/10.3390/life12091365.
-
2.
Dickson RP, Erb-Downward JR, Freeman CM, McCloskey L, Falkowski NR, Huffnagle GB, et al. Bacterial topography of the healthy human lower respiratory tract. mBio. 2017;8(1):e02287-16. [PubMed ID: 28196961]. [PubMed Central ID: PMC5312084]. https://doi.org/10.1128/mBio.02287-16.
-
3.
Hilty M, Burke C, Pedro H, Cardenas P, Bush A, Bossley C, et al. Disordered microbial communities in asthmatic airways. PLoS One. 2010;5(1):e8578. [PubMed ID: 20052417]. [PubMed Central ID: PMC2798952]. https://doi.org/10.1371/journal.pone.0008578.
-
4.
Pragman AA, Kim HB, Reilly CS, Wendt C, Isaacson RE. The lung microbiome in moderate and severe chronic obstructive pulmonary disease. PLoS One. 2012;7(10):e47305. [PubMed ID: 23071781]. [PubMed Central ID: PMC3469539]. https://doi.org/10.1371/journal.pone.0047305.
-
5.
Feigelman R, Kahlert CR, Baty F, Rassouli F, Kleiner RL, Kohler P, et al. Sputum DNA sequencing in cystic fibrosis: Non-invasive access to the lung microbiome and to pathogen details. Microbiome. 2017;5(1):20. [PubMed ID: 28187782]. [PubMed Central ID: PMC5303297]. https://doi.org/10.1186/s40168-017-0234-1.
-
6.
Xia C, Dong X, Li H, Cao M, Sun D, He S, et al. Cancer statistics in China and United States, 2022: Profiles, trends, and determinants. Chin Med J (Engl). 2022;135(5):584-90. [PubMed ID: 35143424]. [PubMed Central ID: PMC8920425]. https://doi.org/10.1097/CM9.0000000000002108.
-
7.
Hosgood III HD, Mongodin EF, Wan Y, Hua X, Rothman N, Hu W, et al. The respiratory tract microbiome and its relationship to lung cancer and environmental exposures found in rural China. Environ Mol Mutagen. 2019;60(7):617-23. [PubMed ID: 30942501]. [PubMed Central ID: PMC8259386]. https://doi.org/10.1002/em.22291.
-
8.
Liu HX, Tao LL, Zhang J, Zhu YG, Zheng Y, Liu D, et al. Difference of lower airway microbiome in bilateral protected specimen brush between lung cancer patients with unilateral lobar masses and control subjects. Int J Cancer. 2018;142(4):769-78. [PubMed ID: 29023689]. https://doi.org/10.1002/ijc.31098.
-
9.
Hanahan D. Hallmarks of cancer: New dimensions. Cancer Discov. 2022;12(1):31-46. [PubMed ID: 35022204]. https://doi.org/10.1158/2159-8290.CD-21-1059.
-
10.
Ran Z, Liu J, Wang F, Xin C, Xiong B, Song Z. Pulmonary micro-ecological changes and potential microbial markers in lung cancer patients. Front Oncol. 2020;10:576855. [PubMed ID: 33537234]. [PubMed Central ID: PMC7848173]. https://doi.org/10.3389/fonc.2020.576855.
-
11.
Lee SH, Sung JY, Yong D, Chun J, Kim SY, Song JH, et al. Characterization of microbiome in bronchoalveolar lavage fluid of patients with lung cancer comparing with benign mass like lesions. Lung Cancer. 2016;102:89-95. [PubMed ID: 27987594]. https://doi.org/10.1016/j.lungcan.2016.10.016.
-
12.
Sommariva M, Le Noci V, Bianchi F, Camelliti S, Balsari A, Tagliabue E, et al. The lung microbiota: role in maintaining pulmonary immune homeostasis and its implications in cancer development and therapy. Cell Mol Life Sci. 2020;77(14):2739-49. [PubMed ID: 31974656]. [PubMed Central ID: PMC7326824]. https://doi.org/10.1007/s00018-020-03452-8.
-
13.
Kovaleva OV, Romashin D, Zborovskaya IB, Davydov MM, Shogenov MS, Gratchev A. Human lung microbiome on the way to cancer. J Immunol Res. 2019;2019:1394191. [PubMed ID: 31485458]. [PubMed Central ID: PMC6710786]. https://doi.org/10.1155/2019/1394191.
-
14.
Yu G, Gail MH, Consonni D, Carugno M, Humphrys M, Pesatori AC, et al. Characterizing human lung tissue microbiota and its relationship to epidemiological and clinical features. Genome Biol. 2016;17(1):163. [PubMed ID: 27468850]. [PubMed Central ID: PMC4964003]. https://doi.org/10.1186/s13059-016-1021-1.
-
15.
Greathouse KL, White JR, Vargas AJ, Bliskovsky VV, Beck JA, von Muhlinen N, et al. Interaction between the microbiome and TP53 in human lung cancer. Genome Biol. 2018;19(1):123. [PubMed ID: 30143034]. [PubMed Central ID: PMC6109311]. https://doi.org/10.1186/s13059-018-1501-6.
-
16.
Laroumagne S, Lepage B, Hermant C, Plat G, Phelippeau M, Bigay-Game L, et al. Bronchial colonisation in patients with lung cancer: a prospective study. Eur Respir J. 2013;42(1):220-9. [PubMed ID: 23100491]. https://doi.org/10.1183/09031936.00062212.
-
17.
Han S, Huang K, Gu Z, Wu J. Tumor immune microenvironment modulation-based drug delivery strategies for cancer immunotherapy. Nanoscale. 2020;12(2):413-36. [PubMed ID: 31829394]. https://doi.org/10.1039/c9nr08086d.
-
18.
Fahrmann JF, Vykoukal JV, Ostrin EJ. Amino acid oncometabolism and immunomodulation of the tumor microenvironment in lung cancer. Front Oncol. 2020;10:276. [PubMed ID: 32266129]. [PubMed Central ID: PMC7105613]. https://doi.org/10.3389/fonc.2020.00276.
-
19.
Zhao Y, Liu Y, Li S, Peng Z, Liu X, Chen J, et al. Role of lung and gut microbiota on lung cancer pathogenesis. J Cancer Res Clin Oncol. 2021;147(8):2177-86. [PubMed ID: 34018055]. [PubMed Central ID: PMC8236441]. https://doi.org/10.1007/s00432-021-03644-0.
-
20.
Petrelli F, Ghidini M, Ghidini A, Perego G, Cabiddu M, Khakoo S, et al. Use of antibiotics and risk of cancer: A systematic review and meta-analysis of observational studies. Cancers (Basel). 2019;11(8):1174. [PubMed ID: 31416208]. [PubMed Central ID: PMC6721461]. https://doi.org/10.3390/cancers11081174.
-
21.
Garcia-Castillo V, Sanhueza E, McNerney E, Onate SA, Garcia A. Microbiota dysbiosis: A new piece in the understanding of the carcinogenesis puzzle. J Med Microbiol. 2016;65(12):1347-62. [PubMed ID: 27902422]. https://doi.org/10.1099/jmm.0.000371.
-
22.
Sun Y, Liu Y, Li J, Tan Y, An T, Zhuo M, et al. Characterization of lung and oral microbiomes in lung cancer patients using culturomics and 16S rRNA gene sequencing. Microbiol Spectr. 2023;11(3):e0031423. [PubMed ID: 37092999]. [PubMed Central ID: PMC10269771]. https://doi.org/10.1128/spectrum.00314-23.
-
23.
Mao Q, Jiang F, Yin R, Wang J, Xia W, Dong G, et al. Interplay between the lung microbiome and lung cancer. Cancer Lett. 2018;415:40-8. [PubMed ID: 29197615]. https://doi.org/10.1016/j.canlet.2017.11.036.