Abstract
Background:
Candida parapsilosis complex, as an opportunistic pathogen, can cause various infections, especially in immunocompromised patients.Objectives:
This study investigated a novel thymoquinone-liposomal nanoparticle to increase the stability of thymoquinone with antifungal effects against C. parapsilosis isolates.Methods:
The thymoquinone was encapsulated in liposomal nanoparticles using a thin-film hydration technique and then analyzed by transmission electron microscopy (TEM), particle size, zeta potential, and UV-visible spectrophotometer. Also, the MTT (3-[4,5-dimethylthiazol-2-yl]-2,5 diphenyl tetrazolium bromide) assay was used on peripheral blood mononuclear cells (PBMCs) for cell metabolic activity. The antifungal activity of thymoquinone-liposomal nanoparticle against 15 clinical isolates of C. parapsilosis and the reference strain was examined based on the M27-A3 guideline.Results:
The synthesized thymoquinone-liposomal nanoparticle was approved by the TEM, particle size, zeta potential, and UV-Vis. The thymoquinone-liposomal nanoparticle showed no toxic effect on PBMCs following the MTT assay. The minimum inhibitory concentration (MIC) range of free thymoquinone and liposomal formulations with inhibitory effects on Candida isolates was 50 to 6.25 and 150 to 18.75 µg/mL, respectively. The MIC50 and MIC90 values of free thymoquinone and thymoquinone-liposomal nanoparticle were 25 and 50, and 75 and 150 µg/mL.Conclusions:
The synthesized thymoquinone-liposomal nanoparticle shows significant antifungal activity against C. parapsilosis isolates compared to free thymoquinone. However, because of its hydrophilicity and hydrophobicity, biocompatibility, particle size, non-toxic effect, and higher cell viability, the thymoquinone-liposomal nanoparticle is a more effective approach for treating fungal infections.Keywords
Antifungal Candida parapsilosis Nano Liposomal Thymoquinone Hydration Method Transmission Electron Microscopy
1. Background
Recently, Candida infections have been increased among immunocompromised patients. Candida parapsilosis is reported as the causative agent in a third of all Candida infections and is associated with high mortality (1, 2). This pathogenic fungal infection shows a high ability to transform from a symbiotic and commensal state to a pathogenic phase. C. parapsilosis can be resistant to treatment with antifungal drugs due to many factors, such as biofilm formation (3, 4). So far, drug resistance with different species of Candida has been reported, especially in hospitalized patients undergoing long-term antifungal drug treatment, in which C. parapsilosis is the common pathogenic yeast among the species (3, 5). Several studies have been conducted to develop antifungal agents based on their low cost, low toxicity, and effectiveness against pathogenic fungal isolates (6, 7).
Many studies have been conducted using nanotechnology to increase the effectiveness of antimicrobial agents, which can be helpful for drug-resistant isolates and various organisms (8, 9). Nano liposomes can adjust drug regulation by loading drugs and transmitting them into the cells, thus diminishing drug resistance. Today, many fungal infections are distinguished, and the importance of herbal medication due to its low side effects has increased (10). Nigella sativa is one of the herbal medication agents that have been used in the treatment of fungal infections and can also decrease clinical signs (11). Thymoquinone (TQ), the major compound of N. sativa, can be used to treat neurovascular and cardiovascular disorders and diminish hypercholesterolemia and excesses of blood sugar (12-14). Thymoquinone, along with related amino acids, will lead to the generation of reactive oxygen species (ROS) (15). Reactive oxygen species metabolic actions cause cell death, which leads to penetrating the sections of cytochrome c and cascade activation (15).
Thymoquinone is severely degraded after light exposure and is not stable in aquatic environments because of its hydrophobic groups. Thus, it is important to encapsulate it in a carrier. Thymoquinone liposomes, after characterization, are an excellent drug delivery system, as they are capable of incorporating both hydrophilic and lipophilic drugs (16). Liposomes can interact with cell membranes, leading to the delivery of the content inside the cell. Liposomes are generally characterized by biocompatibility and biodegradation and are also associated with non-immunogenic, non-toxic, and inflammatory inherent biological characteristics. Also, they can be absorbed by the medicinal target and have high activity, making them a better option in the direction of drug delivery compared to other drug delivery systems, such as nano- and micro-solutions. Due to the shape and surface structure of the liposomal carrier, it can bind to antibodies, peptides, proteins, carbohydrates, and other diverse and different small molecules (17).
2. Objectives
The current study aimed to synthesize and investigate novel TQ-liposomal nanoparticles (TQ-Lip-NP) to increase the stability of TQ with antifungal effects against C. parapsilosis isolates.
3. Methods
3.1. Candida parapsilosis Clinical and Reference Isolates
A total of 15 clinical isolates of C. parapsilosis complex were isolated (2015 - 2019) from hospitalized cases infected by candidemia (bloodstream) in Mashhad, along with a reference strain of C. parapsilosis (ATCC 22019). The matrix-assisted laser desorption ionization-time of flight mass spectrometry (MALDI-TOF) approach was used to identify the clinical Candida cultures (1).
3.2. Preparation and Characterization Techniques of TQ Liposomes
The conventional thin-film hydration technique was used to prepare TQ liposomes (18). Thymoquinone (Cat. No. SC-215986; Sigma Chemical Co., USA) was dissolved in a 2 mL mixture (1:1) of methanol and chloroform. The ingredients (drug/lipid: 1:10) were taken in a flask with a round bottom. Evaporation of the solvents was done in a rotary evaporator to generate a thin lipid film. Using 2 mL of sterile normal saline, the dried lipid film was hydrated, and then brief sonication was done (19). The methods used are summarized using a flowchart diagram in Figure 1.
The flowchart diagram illustrating the processes of synthesizing thymoquinone-liposomal nanoparticles (TQ-Lip-NP) against the clinical isolates of Candida parapsilosis.
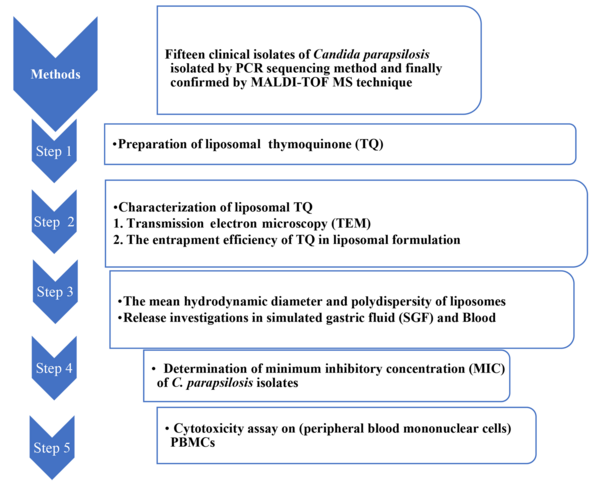
3.3. Transmission Electron Microscopy
The transmission electron microscopy (TEM) evaluated the structure of nanoliposomes (18). Characterization of TQ-loaded liposomes indicated the existence of unilamellar and multilamellar vesicles (Figure 2). Firstly, one drop of nano liposomal preparation was maintained on a platform-coated grid for 20 min. Approximately 10-20 drops of the negative stain (2% uranium acetate; pH 7.0) were flushed on the platform; the grid was dried and observed by TEM, and images were taken by a digital camera. The size of liposomes was 50 - 120 nm, while the TQ-loaded liposomes exhibited bigger sizes (50 - 200 nm). The TQ entrapment efficiency in the liposomal formulation was estimated to be 80% (19).
The thymoquinone-liposomal nanoparticles (TQ-Lip-NP) visualized using transmission electron microscopy (TEM).
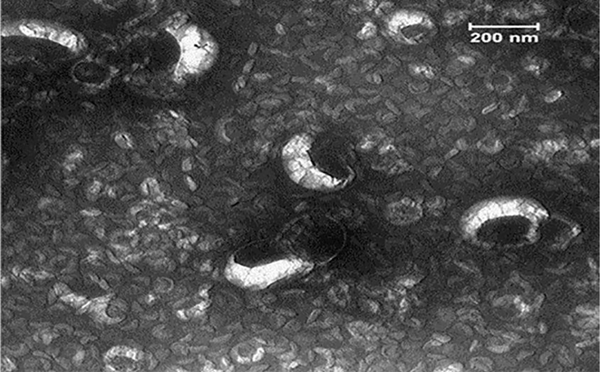
3.4. The Mean Polydispersity and Hydrodynamic Diameter of Liposomes
These parameters were measured using the dynamic light scattering (DLS) method using a Zetasizer Nano ZS (Malvern Instruments, CO., UK) attached to a 633-nm laser source. The dynamic light scattering (Zeta sizer) measured the particle size and Zeta potential of nanoliposomes (Figure 3) (20).
The mean zeta potential average of thymoquinone-liposomal nanoparticles (TQ-Lip-NP)
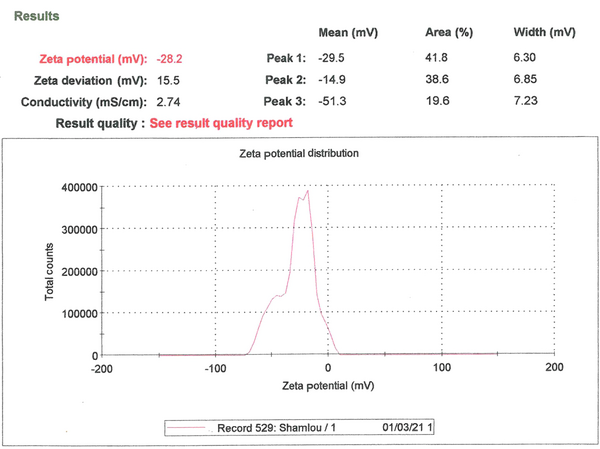
3.5. Release Investigations in Simulated Gastric Fluid and Blood
The release profile of nanoliposomes was assessed in simulated gastric fluid (SGF) and whole blood cells (WBC) to determine the stability of nanoliposomes in SGF and WBC. Hydrochloric acid solution (0.2 N, 39 mL) was mixed with sodium chloride solution (0.2 N, 250 mL), and then 600 mL deionized water (DW) was added to prepare the SGF (pH 2.2), and the ultimate volume was adjusted to 1000 mL using DW. Phosphate buffer saline was adjusted and provided by dissolving KH2PO4 (6.8 g) in DW (250 mL). The mixture was added to NaOH solution (0.2 N, 77 mL), followed by adding 600 mL DW, and the pH was adjusted to 7.4. Finally, the volume of the solution was adjusted to 1000 mL with DW (20).
3.6. Antifungal Susceptibility Testing of TQ-Lip-Ps
This test was done on TQ-Lip-NPs based on the Clinical and Laboratory Standards Institute (CLSI) M27-A3 protocol guidelines (1). For this purpose, 2.5 × 103 CFU/mL of the clinical isolates and reference strain (ATCC 22019) of C. parapsilosis were made using a spectrophotometer and prepared in RPMI 1640 medium (Gibco, Invitrogen, and Carlsbad, CA, USA). Also, two-fold serially diluted concentrations of TQ-Lip-NPs (0.12 - 118 µg/mL) and free TQ (0.78 - 400 µg/mL) were prepared in RPMI 1640 medium. Then, yeast suspensions (100 μL) and 100 μL of each of the TQ compounds were added into a 96-well microplate (1). The microplate underwent incubation at 37°C for 48 hours, and the lowest concentration inhibiting 80% of the Candida growth was considered the minimum inhibitory concentration (MIC). Candida suspension with no treatment was considered a positive control, and the culture medium was the negative control (1).
3.7. Cytotoxicity Assay on Peripheral Blood Mononuclear Cells
The mitochondrial function of peripheral blood mononuclear cells (PBMCs) was investigated using tetrazolium salt reduction by the MTT (3-[4,5-dimethylthiazol-2-yl]-2,5-diphenyl tetrazolium bromide) method (14). For MTT dye preparation, MTT powder (50 mg) was added to PBS (10 mL), followed by sterilizing by a 0.22 µm filter. Then, 5 × 103 cells were calculated in the DMEM medium with 10% fetal bovine serum (FBS) and 1% streptomycin/penicillin (BiIdia Corporation, Iran). Afterward, the suspension (100 μL) was seeded in a microplate with 96 wells and underwent incubation with 5% CO2 at 37°C for 24 hours. Then, 100 μL of free TQ and TQ-Lip-NPs were added to each well, and the plate was subjected to incubation for 24 hours. Then, MTT dye (10 μL) was added to the wells, and we placed the microplate in the dark for 4 hours. DMSO (Merck, Germany) was then added to the wells, and 15 min later, an ELISA reader (STAT FAX 2100) recorded the absorbance at 590 - 600 nm. The cell suspension with no exposure to these agents was regarded as a positive control, and the culture medium was applied as a negative control. The blank well included DMSO and tetrazolium salt. The experiments were conducted three times. The following equation was used to obtain the results:
Cell viability (%) = the test absorbance/the control absorbance × 1.
3.8. Statistical Analysis
The experiments were analyzed using the Npar Test, and the average of the measurements was considered the results. Statistical analyses were done by the Mann-Whitney U test using SPSS 23, with a P-value of < 0. 5 was assumed to be significant.
4. Results
4.1. Characterization Techniques of NPs
The synthesized TQ-Lip-NP was approved by the TEM, particle size, zeta potential, and UV-Vis. Size measurements were done three times, and the mean of the recordings was reported (Table 1).
Zeta Potential, Particle Size, and Polydispersity Index Analysis of the Thymoquinone-Liposomal Nanoparticle
Zeta Average (nm) | Zeta Potential (mv) | PDI | Encapsulation Efficiency | |
---|---|---|---|---|
TQ-Lip-NP | 74 ± 0.2 | -28 ± 2 | 0.2 | 76 ± 4 |
4.1.1. The Average Particle Size Results of TQ-Lip-NP
The average particle size (ZAve) results of TQ-Lip-NP with 74.4 and a polydispersity index (PDI) of 0.2 showed a good mean average size and polydispersity in liposomal formulation (Figure 4). The mean average of the zeta potential of -28 mv showed a good negative voltage charge with no agglutination or aggregation of nanoliposomes (Figure 4).
The average particle size (ZAve) and polydispersity index (PDI) of thymoquinone-liposomal nanoparticles (TQ-Lip-NP)
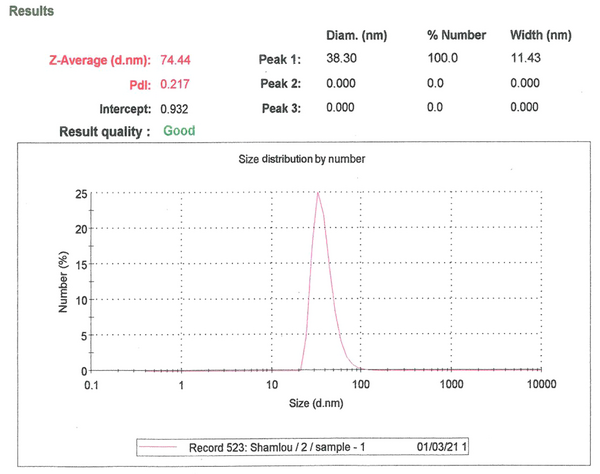
4.1.2. The Encapsulation Efficiency of TQ-Lip-NP
The encapsulation efficiency (EE%) of TQ-Lip-NP was 76.9%. The viability percentages of PBMCs receiving TQ-Lip-NPs and free TQ during incubation for 24 hours are provided in Figure 5.
The assessment of the cytotoxic effects or cell viability percentage of thymoquinone-liposomal nanoparticles (TQ-Lip-NP) and free TQ
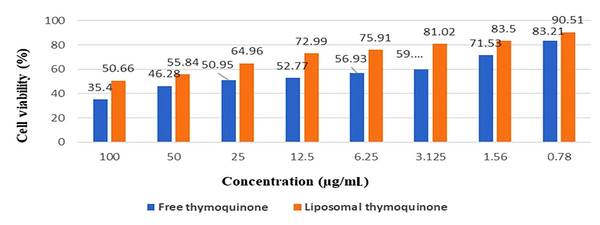
4.2. The Viability Percentages of Cells Treated with TQ-Lip-NPs and Free TQ
The viability percentages of cells treated with TQ-Lip-NPs and free TQ were 90 and 83%, respectively. TQ-Lip-NPs showed lower toxicity and a dose-dependent effect on the viability of PBMCs. Moreover, the TQ-Lip-NP showed no toxic effect on PBMCs following the MTT assay (P-value < 0.5) (Figure 5).
4.3. Release Profile
About 72% and 75% of TQ-Lip-NP were released mainly around 6 h at pH values of 7.4 and 2.2, respectively (P-value < 0.5) (Figure 6).
Release results of liposomal formulation of thymoquinone (TQ), in different pH values: Simulated gastric fluid (SGF) with a pH of 2.2 and Phosphate buffer saline (similar to the acidity of whole blood) with a pH of 7.4
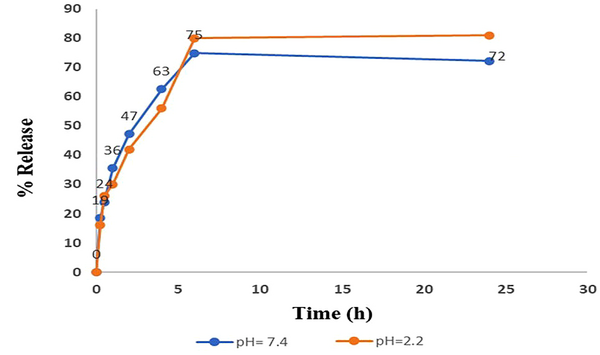
4.4. The MIC Range of Free TQ and Liposomal Formulation
The MIC range of free TQ and liposomal formulations with inhibitory effects against Candida isolates was 50 - 6.25 and 150 - 18.75 µg/mL, respectively. Moreover, the MIC50 and MIC90 values of free TQ and TQ-Lip-NP were 25 and 50, and 75 and 150 µg/mL, respectively (Table 2). Three isolates (C3, C7, and C10) showed highly significant resistance to free TQ, and seven isolates (C2, C5, C6, C8, C11, C13, and C15) showed more significant resistance to the liposomal formulation of TQ than the others (P-value < 0.5) (Table 3). The geometric mean of free TQ and TQ-Lip-NP was 21 and 55, and the MIC50 and MIC90 values were 25 and 50, and 75 and 150 µg/mL, respectively.
The Minimum Inhibitory Concentration Results of the Thymoquinone on the Growth of Clinical Isolates and Reference Strain of Candida parapsilosis Isolates
Candida parapsilosis Isolates | 400 µg/mL | 200 µg/mL | 100 µg/mL | 50 µg/mL | 25 µg/mL | 12.5 µg/mL | 6.25 µg/mL | 3.125 µg/mL | 1.56 µg/mL | 0.78 µg/mL | PC | NC |
---|---|---|---|---|---|---|---|---|---|---|---|---|
C1 | S | S | S | S | S | S | S a | R | R | R | + | - |
C2 | S | S | S | S | S a | R | R | R | R | R | + | - |
C3 | S | S | S | S a | R | R | R | R | R | R | + | - |
C4 | S | S | S | S | S a | R | R | R | R | R | + | - |
C5 | S | S | S | S | S | S a | R | R | R | R | + | - |
C6 | S | S | S | S | S a | R | R | R | R | R | + | - |
C7 | S | S | S | S a | R | R | R | R | R | R | + | - |
C8 | S | S | S | S | S a | R | R | R | R | R | + | - |
C9 | S | S | S | S | S | S a | R | R | R | R | + | - |
C10 | S | S | S | S a | R | R | R | R | R | R | + | - |
C11 | S | S | S | S | S a | R | R | R | R | R | + | - |
C12 | S | S | S | S | S | S | S a | R | R | R | + | - |
C13 | S | S | S | S | S | S a | R | R | R | R | + | - |
C14 | S | S | S | S | S a | R | R | R | R | R | + | - |
C15 | S | S | S | S | S | S a | R | R | R | R | + | - |
Reference strain 22019 | S | S | S | S a | R | R | R | R | R | R | + | - |
The Minimum Inhibitory Concentration Results of the Thymoquinone-Liposomal Nanoparticles on the Growth of Clinical Isolates and Reference Strain of Candida parapsilosis Isolates
Candida parapsilosis Isolates | 600 µg/mL | 300 µg/mL | 150 µg/mL | 75 µg/mL | 37.5 µg/mL | 18.75 µg/mL | 9.37 µg/mL | 4.68 µg/mL | 2.34 µg/mL | 1.17 µg/mL | PC | NC |
---|---|---|---|---|---|---|---|---|---|---|---|---|
C1 | S | S | S | S | S a | R | R | R | R | R | + | - |
C2 | S | S | S a | R | R | R | R | R | R | R | + | - |
C3 | S | S | S | S a | R | R | R | R | R | R | + | - |
C4 | S | S | S | S | S a | R | R | R | R | R | + | - |
C5 | S | S | S a | R | R | R | R | R | R | R | + | - |
C6 | S | S | S | S a | R | R | R | R | R | R | + | - |
C7 | S | S | S | S | S | S a | R | R | R | R | + | - |
C8 | S | S | S | S a | R | R | R | R | R | R | + | - |
C9 | S | S | S | S | S | S a | R | R | R | R | + | - |
C10 | S | S | S | S | S a | R | R | R | R | R | + | - |
C11 | S | S | S | S a | R | R | R | R | R | R | + | - |
C12 | S | S | S | S | S a | R | R | R | R | R | + | - |
C13 | S | S | S | S a | R | R | R | R | R | R | + | - |
C14 | S | S | S | S | S | S a | R | R | R | R | + | - |
C15 | S | S | S a | R | R | R | R | R | R | R | + | - |
Reference strain 22019 | S | S | S | S a | R | R | R | R | R | R | + | - |
5. Discussion
The emergence of drug-resistant species has caused the development of novel drugs to solve this problematic issue. Using essential oils, like TQ, as the major components of N. sativa improves the effectiveness of antifungal agents and reduces antifungal resistance (14, 21). The prevalence of Candida infections has increased in recent years due to the increase in the number of patients with immune system disorders, especially Candida species resistant to antifungal drugs (1, 5). Candidemia is the most common form of Candida species-related invasive disease; C. parapsilosis is the second or third most common and is the fourth or sixth most common nosocomial infection (1, 2). The increase in the frequency of C. parapsilosis infections has been attributed to various factors, including biofilm formation. Immunocompromised patients are at high risk of being affected by C. parapsilosis, particularly patients requiring prolonged use of a central venous catheter (1).
The current study synthesized TQ-Lip-NP as a new antifungal formulation against clinical isolates of C. parapsilosis complex isolated from those with candidemia. Recently, alternative therapies, like herbal medicines, have been considered for microbial infections (21). For instance, the antifungal effect of N. sativa on the Candida genus has been reported (22). However, the antifungal activity of TQ (a major and essential component of N. sativa) is higher than that of N. sativa. Almshawit and Macreadie (23) and Rahsepar et al. (24) reported that the MIC value of TQ against C. albicans was 50 µg/mL, which is similar to our results (23, 24). In our study, the MIC50 and MIC90 values of free TQ were 25 and 50 µg/mL, respectively. However, two major problems with using free TQ are its sensitivity to light and its insoluble nature in aquatic environments, which should be considered when using this compound.
Thymoquinone, as a fungicidal compound, can induce oxidative stress, which damages biological molecules. It is also a lipophilic compound with poor solubility in an aqueous medium (23). Hence, to enhance the TQ activity, a liposomal formulation was investigated. Because of its low solubility in water, encapsulation of TQ not only solves this problem but also improves its efficiency due to its gradual release from the nanoliposomal structure (25). On the other hand, the development of biosynthesized NPs can significantly reduce drug-resistant and challenging-to-treat Candida infections, as has been shown in various studies. Furthermore, NPs have demonstrated high biological activity and low toxicity. Randhawa et al. (26) and Rahsepar et al. (24) reported that the MIC value of nano-sized TQ was 160 µg/mL (24, 26). However, our MIC50 and MIC90 values for TQ-Lip-NP were 75 and 150 µg/mL, which is less than the mentioned studies. This could be due to the better synthesis of nanoliposomes made in this study or the difference in the structure of the studied organisms.
In this study, although the geometric mean of free TQ was less than that of TQ-Lip-NP (21 compared to 55), with a stronger effect of antifungal properties, according to its cytotoxicity effect and its compatibility with normal cells, TQ-Lip-NP showed significantly fewer toxic effects on PBMCs than free TQ. Furthermore, the MIC50 and MIC90 of free TQ were less than those of TQ-Lip-NP, and these data could be evaluated in the course of a clinical trial study in the future. Bhattacharjee et al. (27) and Rahsepar et al. (24) reported that the viability percentage of PBMCs treated with TQ was merely 42%, and the free TQ concentration range was 9 µg/mL, which is not consistent with our findings (24, 27). The study results showed that TQ-Lip-NP has more acceptable effects with a viability of 90% (compared to 83% of free TQ) at a concentration of 0.78 µg/mL. The cytotoxicity results showed that TQ-Lip-NPs were more biocompatible compared to free TQ and that toxicity showed a significant reduction when liposome forms were applied.
TQ-Lip-NPs were safer and more biocompatible and effective compared to free TQ; thus, they can be an appropriate alternative to azole drugs with many side effects. Furthermore, considering the tendency to form fungal biofilms among C. parapsilosis isolates following the use of venous catheters, it seems that the structures of nanoliposomes can also be used to penetrate these resistant structures. The current study evaluated clinical isolates of C. parapsilosis isolated from high-risk candidemia hospitalized patients following central venous catheters. Nonetheless, more in vivo and in vitro studies are required to evaluate the efficacy of nanoliposomal structures.
One of the limitations of our study is the relatively small number of isolates of C. parapsilosis. Moreover, other Candida species were not investigated. Therefore, if more isolates and Candida species could be investigated, the possibility of applying and using these nanoliposomal compounds in future clinical studies could be given special attention.
5.1. Conclusions
The novel synthesized TQ-Lip-NP was approved by the TEM method, particle size, zeta potential, and UV-Vis. TQ-Lip-NP showed no significant antifungal activity against C. parapsilosis complex isolates compared to free TQ. However, because of its hydrophilicity and hydrophobicity, particle size, biocompatibility, non-toxic effect, and higher cell viability, TQ-Lip-NP could be considered a more effective approach to treating Candida infections.
References
-
1.
Arastehfar A, Daneshnia F, Najafzadeh MJ, Hagen F, Mahmoudi S, Salehi M, et al. Evaluation of molecular epidemiology, clinical characteristics, antifungal susceptibility profiles, and molecular mechanisms of antifungal resistance of Iranian Candida parapsilosis species complex blood isolates. Front Cell Infect Microbiol. 2020;10:206. [PubMed ID: 32509592]. [PubMed Central ID: PMC7253641]. https://doi.org/10.3389/fcimb.2020.00206.
-
2.
Trofa D, Gacser A, Nosanchuk JD. Candida parapsilosis, an emerging fungal pathogen. Clin Microbiol Rev. 2008;21(4):606-25. [PubMed ID: 18854483]. [PubMed Central ID: PMC2570155]. https://doi.org/10.1128/CMR.00013-08.
-
3.
Silva S, Rodrigues CF, Araujo D, Rodrigues ME, Henriques M. Candida species biofilms' antifungal resistance. J Fungi (Basel). 2017;3(1):8. [PubMed ID: 29371527]. [PubMed Central ID: PMC5715972]. https://doi.org/10.3390/jof3010008.
-
4.
Melo AS, Bizerra FC, Freymuller E, Arthington-Skaggs BA, Colombo AL. Biofilm production and evaluation of antifungal susceptibility amongst clinical Candida spp. isolates, including strains of the Candida parapsilosis complex. Med Mycol. 2011;49(3):253-62. [PubMed ID: 21039308]. https://doi.org/10.3109/13693786.2010.530032.
-
5.
Zarrinfar H, Kord Z, Fata A. High incidence of azole resistance among Candida albicans and C. glabrata isolates in Northeastern Iran. Curr Med Mycol. 2021;7(3):18-21. [PubMed ID: 35528623]. [PubMed Central ID: PMC9006729]. https://doi.org/10.18502/cmm.7.3.7801.
-
6.
Roemer T, Krysan DJ. Antifungal drug development: Challenges, unmet clinical needs, and new approaches. Cold Spring Harb Perspect Med. 2014;4(5):a019703. [PubMed ID: 24789878]. [PubMed Central ID: PMC3996373]. https://doi.org/10.1101/cshperspect.a019703.
-
7.
Moghadam S, Azari B, Rashidi R, Bafghi MH, Rakhshandeh H, Selman SM, et al. Antifungal activity of three different varieties of Capsicum annuum against clinical isolates of Candida species. Trop Dis Travel Med Vaccines. 2023;9(1):9. [PubMed ID: 37468970]. [PubMed Central ID: PMC10357713]. https://doi.org/10.1186/s40794-023-00194-w.
-
8.
Hassanpour P, Hamishehkar H, Bahari Baroughi B, Baradaran B, Sandoghchian Shotorbani S, Mohammadi M, et al. Antifungal effects of voriconazole-loaded nano-liposome on fluconazole-resistant clinical isolates of Candida albicans, biological activity and ERG11, CDR1, and CDR2 gene expression. Assay Drug Dev Technol. 2021;19(7):453-62. [PubMed ID: 34435891]. https://doi.org/10.1089/adt.2020.1057.
-
9.
Moghadam S, Azari B, Darroudi M, Zarrinfar H, Sabouri Z, Mohammed Selman S, et al. Comparison of antifungal activities of zinc, copper, cerium oxide, silver, gold, and selenium nanoparticles against clinical isolates of Aspergillus. Nanomed J. 2023;10(3):227-33. https://doi.org/10.22038/nmj.2023.71162.1762.
-
10.
Azari B, Zahmatkesh Moghadam S, Zarrinfar H, Tasbandi A, Jamialahmadi T, Sahebkar A. Antifungal activity of curcuminoids and difluorinated curcumin against clinical isolates of Candida species. In: Sahebkar A, Sathyapalan T, editors. Natural Products and Human Diseases. Advances in Experimental Medicine and Biology. Vol. 1328. Cham: Springer; 2021. p. 123-9. https://doi.org/10.1007/978-3-030-73234-9_8.
-
11.
Ahmad A, Husain A, Mujeeb M, Khan SA, Najmi AK, Siddique NA, et al. A review on therapeutic potential of Nigella sativa: A miracle herb. Asian Pac J Trop Biomed. 2013;3(5):337-52. [PubMed ID: 23646296]. [PubMed Central ID: PMC3642442]. https://doi.org/10.1016/S2221-1691(13)60075-1.
-
12.
Nemmar A, Al-Salam S, Zia S, Marzouqi F, Al-Dhaheri A, Subramaniyan D, et al. Contrasting actions of diesel exhaust particles on the pulmonary and cardiovascular systems and the effects of thymoquinone. Br J Pharmacol. 2011;164(7):1871-82. [PubMed ID: 21501145]. [PubMed Central ID: PMC3246712]. https://doi.org/10.1111/j.1476-5381.2011.01442.x.
-
13.
Raghunandhakumar S, Paramasivam A, Senthilraja S, Naveenkumar C, Asokkumar S, Binuclara J, et al. Thymoquinone inhibits cell proliferation through regulation of G1/S phase cell cycle transition in N-nitrosodiethylamine-induced experimental rat hepatocellular carcinoma. Toxicol Lett. 2013;223(1):60-72. [PubMed ID: 24012840]. https://doi.org/10.1016/j.toxlet.2013.08.018.
-
14.
Erboga M, Kanter M, Aktas C, Sener U, Fidanol Erboga Z, Bozdemir Donmez Y, et al. Thymoquinone ameliorates cadmium-induced nephrotoxicity, apoptosis, and oxidative stress in rats is based on its anti-apoptotic and anti-oxidant properties. Biol Trace Elem Res. 2016;170(1):165-72. [PubMed ID: 26226832]. https://doi.org/10.1007/s12011-015-0453-x.
-
15.
Yu SM, Kim SJ. Thymoquinone-induced reactive oxygen species causes apoptosis of chondrocytes via PI3K/Akt and p38kinase pathway. Exp Biol Med (Maywood). 2013;238(7):811-20. [PubMed ID: 23788172]. https://doi.org/10.1177/1535370213492685.
-
16.
Odeh F, Ismail SI, Abu-Dahab R, Mahmoud IS, Al Bawab A. Thymoquinone in liposomes: A study of loading efficiency and biological activity towards breast cancer. Drug Deliv. 2012;19(8):371-7. [PubMed ID: 23043626]. https://doi.org/10.3109/10717544.2012.727500.
-
17.
Naik SR, Desai SK, Shah PD, Wala SM. Liposomes as potential carrier system for targeted delivery of polyene antibiotics. Recent Pat Inflamm Allergy Drug Discov. 2013;7(3):202-14. [PubMed ID: 23745986]. https://doi.org/10.2174/1872213x113079990016.
-
18.
Shukla R, Cheryan M. Zein: The industrial protein from corn. Ind Crops Prod. 2001;13(3):171-92. https://doi.org/10.1016/s0926-6690(00)00064-9.
-
19.
Khan MA, Aljarbou AN, Khan A, Younus H. Liposomal thymoquinone effectively combats fluconazole-resistant Candida albicans in a murine model. Int J Biol Macromol. 2015;76:203-8. [PubMed ID: 25709021]. https://doi.org/10.1016/j.ijbiomac.2015.02.015.
-
20.
Hatamipour M, Sahebkar A, Alavizadeh SH, Dorri M, Jaafari MR. Novel nanomicelle formulation to enhance bioavailability and stability of curcuminoids. Iran J Basic Med Sci. 2019;22(3):282-9. [PubMed ID: 31156789]. [PubMed Central ID: PMC6528723]. https://doi.org/10.22038/ijbms.2019.32873.7852.
-
21.
Katiraee F, Eidi S, Bahonar AR, Zarrinfar H, Khosravi AR. [Comparision of MICs of some Iranian herbal essences against azole resistance and azole susceptible of Candida albican]. J Med Plants. 2008;7(27):37-44. Persian.
-
22.
Moghim H, Taghipoor S, Shahinfard N, Kheiri S, Panahi R. Antifungal effects of Zataria multiflora and Nigella sativa extracts against Candida albicans. J Herbmed Pharmacol. 2015;4(4):138-41.
-
23.
Almshawit H, Macreadie I. Fungicidal effect of thymoquinone involves generation of oxidative stress in Candida glabrata. Microbiol Res. 2017;195:81-8. [PubMed ID: 28024529]. https://doi.org/10.1016/j.micres.2016.11.008.
-
24.
Rahsepar S, Roudbar Mohammadi S, Delavari H, Roudbari M, Mohammad Hassan Z. Design and synthesis of novel thymoquinone-zein nanoparticles; evaluation of the inhibitory effect on Candida albicans and biofilm formation in vitro. Infect Epidemiol Microbiol. 2022;8(2):169-76. https://doi.org/10.52547/iem.8.2.169.
-
25.
Shariare MH, Khan MA, Al-Masum A, Khan JH, Uddin J, Kazi M. Development of stable liposomal drug delivery system of thymoquinone and its in vitro anticancer studies using breast cancer and cervical cancer cell lines. Molecules. 2022;27(19):6744. [PubMed ID: 36235288]. [PubMed Central ID: PMC9571792]. https://doi.org/10.3390/molecules27196744.
-
26.
Randhawa MA, Gondal MA, Al-Zahrani AH, Rashid SG, Ali A. Synthesis, morphology and antifungal activity of nano-particulated amphotericin-B, ketoconazole and thymoquinone against Candida albicans yeasts and Candida biofilm. J Environ Sci Health A Tox Hazard Subst Environ Eng. 2015;50(2):119-24. [PubMed ID: 25560257]. https://doi.org/10.1080/10934529.2015.975042.
-
27.
Bhattacharjee M, Upadhyay P, Sarker S, Basu A, Das S, Ghosh A, et al. Combinatorial therapy of Thymoquinone and Emodin synergistically enhances apoptosis, attenuates cell migration and reduces stemness efficiently in breast cancer. Biochim Biophys Acta Gen Subj. 2020;1864(11):129695. [PubMed ID: 32735937]. https://doi.org/10.1016/j.bbagen.2020.129695.