Abstract
Background:
Vibrio vulnificus can cause serious infections in human beings associated with the consumption of raw oysters or cuts exposed to seawater. The traditional method for culturing V. vulnificus is time-consuming and has a high failure rate.Objectives:
This study aims to detect V. vulnificus using an AMCA-modified specific DNA aptamer.Methods:
Common pathogenic microorganisms present in the seawater of the Fujian Sea area were collected, cultured, and identified. The samples were found to contain mainly V. vulnificus, Staphylococcus aureus, Escherichia coli, Pseudomonas aeruginosa, V. alginolyticus, V. parahaemolyticus, and V. cholerae. AMCA was conjugated with 5’ ends of the aptamer using N-hydroxysuccinimide ester (NHS ester) to target V. vulnificus and produce a fluorescent signal upon binding. The aptamer was screened and optimized for rapid detection of V. vulnificus. We collected the 50 bacterial strains isolated from clinical secretion samples and used a fluorescence microscope to determine whether the sample contained V. vulnificus or not. We compared these results with those obtained from VITEK MS (considered the gold standard) to test the sensitivity and specificity of the AMCA-modified aptamer using IBM SPSS Statistics 22.Results:
In this experiment, the sensitivity and specificity of the modified aptamer for detecting V. vulnificus were determined to be 100% [95% CI (0.39, 1)] and 93.4% [95% CI (0.81, 0.98)], respectively. The positive predictive value was 57% [95% CI (0.20, 0.88)], and the negative predictive value was 100% [95% CI (0.89, 1)]. These findings indicate that V. vulnificus specimens can be rapidly detected via fluorescence reaction within 30 minutes.Conclusions:
Our results suggest that this modified DNA aptamer has the potential to be used for diagnosing V. vulnificus. Further research is needed to explore the application of aptamers in pathogen infections.Keywords
DNA Aptamers Vibrio vulnificus Fluorescence Rapid Detection Sensitivity Specificity
1. Background
Vibrio vulnificus is an important bacterial species that causes serious infections in humans (1). This bacterium has the highest mortality rate among various foodborne pathogens, and death often occurs rapidly. While other foodborne illnesses in the United States are decreasing, V. vulnificus cases continue to increase. Illness caused by V. vulnificus is typically associated with the consumption of raw oysters or cuts exposed to seawater (2), including individuals who have been injured and exposed to contaminated seawater. The prevalence of V. vulnificus heavily depends on the temperature and salinity of the seawater (3-6). The mortality rate of V. vulnificus infection has been reported to be 18 - 54% in the literature. If antibiotic therapies are delayed, the risk of death from the infection significantly increases (7, 8). While many effective detection methods targeting this pathogen have been designed, most of these methods are time-consuming, complicated, or expensive (9-12).
Identification via mass spectrometry is reliable, but its efficacy depends on the method used to culture the bacteria from patient samples. Metagenomic next-generation sequencing (NGS) is expensive and can take 4 - 5 hours. Vibrio vulnificus can also be tested using VITEK MS. VITEK MS has been widely used as an instrument for the clinical identification of pathogenic microorganisms (13-16). Quick identification of V. vulnificus is needed, particularly for patients with puncture wounds and skin ulcerations. Fan et al. (17) reported the successful detection of V. vulnificus with a DNAzyme-Based Biosensor; Yan et al. (18) designed a novel DNA aptamer using asymmetric polymerase chain reaction and exponential enrichment ligand phylogenetic evolution to target V. vulnificus. Although DNA aptamers have been reported to detect pathogenic bacteria, they have not yet been widely used in clinical applications.
2. Objectives
The sensitivity and specificity of aptamers to clinical strains have not been tested. Therefore, we sought to test a highly specific DNA aptamer in our clinic for the detection of V. vulnificus. We used the same V. vulnificus DNA aptamers as Yan et al. (18), which have not been tested yet. However, the sensitivity and specificity of the aptamer are still unknown. Hence, we aimed to modify the aptamer for more rapid detection of V. vulnificus.
3. Methods
Seawater was collected three times from the Chang Le and Ping Tan areas of the Fujian province. We filtered 300 mL of seawater each time using a filter (VACUUM PRESSURE PUMP AP-01. Then, we inoculated the filter paper with alkaline peptone water, blood agar plates (Antu Biology, China), and thiosulfate citrate bile salts Sucrose plate (TCBS plate, Di Jing Biology, China). After 24 hours, the alkaline peptone water was transferred to a TCBS plate. If the bacteria grew on the plate, we identified every bacterial strain present using a mass spectrometer (VITEK MS, Merieux, France). We collected bacterial samples from seawater, including V. vulnificus, Staphylococcus aureus, Escherichia coli, Pseudomonas aeruginosa, V. alginolyticus, V. parahaemolyticus, V. cholerae. Vibrio vulnificus is often responsible for wound infections, so we collected 50 stains (Table 1), including four samples of V. vulnificus obtained from laboratory bacterial cultures of patients who were injured and exposed to contaminated seawater. These bacteria were isolated from wound secretions with a high isolation rate at the hospital over the preceding two years. After reinvigoration, the bacteria were inoculated onto Colombian blood agar plates and identified using VITEK MS.
The 50 Strains Tested by AMCA-Modified DNA Aptamer (four Vibrio vulnificus, one V. alginolyticus, one V. parahaemolyticus, and the 44 Most Prevalent Bacterial Strains Present in Clinical Samples of Seawater Infection Secretions)
No | Strain | No | Strain | No | Strain |
---|---|---|---|---|---|
1 | Staphylococcus aureus | 18 | Klebsiella aerogenes | 35 | Citrobacter koseri |
2 | Escherichia coli | 19 | Staphylococcus lugdunensis | 36 | Haemophilus parainfluenzae |
3 | Pseudomonas aeruginosa | 20 | Corynebacterium striatum | 37 | Pseudomonas putida |
4 | Klebsiella pneumoniae | 21 | Streptococcus constellatus | 38 | Streptococcus mitis |
5 | Staphylococcus epidermidis | 22 | Stenotrophomonas maltophilia | 39 | Enterococcus avium |
6 | Enterococcus faecalis | 23 | Streptococcus pyogenes | 40 | Moraxella osloensis |
7 | Staphylococcus haemolyticus | 24 | Haemophilus influenzae | 41 | Aeromonas hydrophila |
8 | Acinetobacter baumannii | 25 | Corynebacterium tuberculostearicum | 42 | Citrobacter braakii |
9 | Proteus mirabilis | 26 | Klebsiella oxytoca | 43 | nonO1/O139 Vibrio cholerae |
10 | Bacillus cereus | 27 | Staphylococcus capitis | 44 | Pseudomonas stutzeri |
11 | Morganella morganii | 28 | Streptococcus dysgalactiae | 45 | Vibrio vulnificus |
12 | Enterobacter cloacae | 29 | Staphylococcus hominis | 46 | Vibrio vulnificus |
13 | Proteus vulgaris | 30 | Raoultella ornithinolytica | 47 | Vibrio vulnificus |
14 | Serratia marcescens | 31 | Corynebacterium amycolatum | 48 | Vibrio vulnificus |
15 | Streptococcus agalactiae | 32 | Citrobacter freundii | 49 | Vibrio alginolyticus |
16 | Streptococcus anginosus | 33 | Achromobacter xylosoxidans | 50 | Vibrio parahaemolyticus |
17 | Enterococcus faecium | 34 | Staphylococcus warneri |
The V. vulnificus DNA aptamers were previously reported by Yan et al. (18) and purchased from Sangon Biotech (Shanghai, China). AMCA was conjugated with 5’ ends of the aptamer using N-hydroxysuccinimide ester (NHS ester) by Sangon Biotech (Shanghai, China). Over ten such aptamers have been reported in the literature, and therefore, we chose to modify the one with the highest affinity (sequence from 5’ to 3’: AGGGTTGGTCGTCCAGCATTCCGTTCGATTGTAGTTCTGACTCTT GTGAAGTTAATGAGCTCCTTTTGCTGACTGTTGTT). The AMCA-modified DNA aptamer allows it to express a fluorescent signal if it b binds to its V. vulnificus target. Based on this property, we were able to quickly detect V. vulnificus through fluorescence microscopy. The adaptor peak spectrum was detected through external testing using the Thermo Scientific LTQ Orbitrap XL ETD.
We measured the binding affinity of aptamers for these strains under similar conditions but with varying bacterial solution concentrations while the aptamer was kept at a concentration of 100 umol/. The aptamer used in the experiment was AMCA modified aptamer. We also observed the binding affinity of the aptamer under the 45G-530 fluorescence microscope (Olympus BX53, Japan) at a fixed final concentration of 100umol/L AMCA-modified aptamer over different incubation times. Then mixture of V. vulnificus and AMCA-modified aptamer could emit blue fluorescence between 440 - 460 nm under the fluorescence microscope in the 30 minutes. Therefore, we selected the aptamer with the highest binding affinity for V. vulnificus and analyzed its specificity and detection limits.
We used normal saline to adjust the bacterial solution to 0.1 McTurbidimetric and prepared the AMCA-modified aptamer concentration at 100 umol/L using distilled water. Then, we mixed 1uL of 0.1 McTurbidimetric bacterial solution, 1.5 uL of 100umol/L AMCA-modified aptamer, and 2.5 uL of 0.85% normal saline solution together in a new test tube. The absorption of the 1 μL sample of mixture onto the slide, we covered it with a cover glass. Then, the fluorescence microscope was used to detect the presence of V. vulnificus. In this experiment, we used AMCA-modified aptamer and V. vulnificus as controls, respectively, to eliminate the fluorescent interference of reagents and strains. We then recorded each bacterial fluorescence microscopy result. We used the result of VITEK MS as the diagnostic gold standard to calculate the accuracy of strains. The main observation indicators were the detection of mass spectrometry as the diagnostic gold standard, and the sensitivity, specificity, accuracy, positive predictive value, and negative predictive value of the aptamer for pathogen detection were calculated by IBM SPSS statistics 22.
4. Results
The bacterial species we cultured from seawater were V. vulnificus, S. aureus, E. coli, P. aeruginosa, V. alginolyticus, V. parahaemolyticus, and V. cholerae. It has been reported that the strains of V. vulnificus, S. aureus, E. coli, V. alginolyticus, V. parahaemolyticus, and V. cholerae are abundant in the ocean (6, 19, 20). Our results showed similar characteristics to those of comparable previous reports in the literature (21). Bacteria detected in seawater were incorporated into the 50 strains tested by the aptamer (Table 1). These bacteria were isolated from secretions with a high isolation rate at the hospital over the preceding two years. After reinvigoration, the bacteria were inoculated onto Colombian blood agar plates and identified using a flight mass spectrometer. All the 50 strains tested by VITEK MS had a single high-confidence identification.
The aptamer peak spectrum was detected through external testing by Sangon Biotech (Shanghai, China) using Thermo Scientific LTQ Orbitrap XL ETD, as shown in Figure 1. A single high crest can be seen in the figure, indicating the high purity of the AMCA-modified DNA aptamer. In this experiment, the study setup took approximately 40 minutes in duration; we used DNA aptamer (Figure 2D) and V. vulnificus (Figure 2B) as controls, respectively, to eliminate the fluorescent interference of the reagents and strains. We cannot see blue fluorescence under the fluorescence microscope.
The peak spectrum of the aptamer by Thermo Scientific LTQ Orbitrap XL ETD. From the single peak, it can be seen that the aptamer was of a high purity
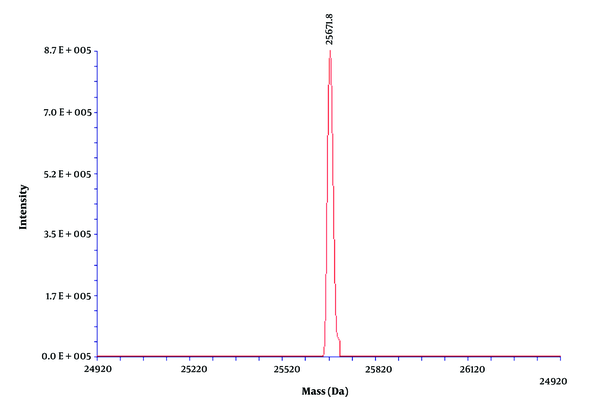
In Figure 2, we can see strains such as Aeromonas hydrophila (Figure 2F), Streptococcus dysgalactiae (Figure 2G), and Enterococcus faecalis (Figure 2E) after binding to the AMCA-modified specific DNA aptamer. They fluoresce blue under the fluorescence microscope, indicating a positive result. However, we cannot see any blue fluorescence from the E. coli (Figure 2C) mixture under the fluorescence microscope, suggesting a negative result. The specific calculation methods are shown in Table 2. Our results indicate that the sensitivity and specificity of the modified aptamer for detecting V. vulnificus were 100% [95% CI (0.39, 1)] and 93.4% [95% CI (0.81, 0.98)], respectively. The positive predictive value is 57% [95% CI (0.20, 0.88)], and the negative predictive value is 100% [95% CI (0.89, 1)], as shown in Table 2.
A, Vibrio vulnificus and DNA aptamer; B, V. vulnificus; C, Escherichia coli and DNA aptamer; D, DNA aptamer; E, Enterococcus faecalis and DNA aptamer; F, Aeromona hydrophila and DNA aptamer; G, Streptococcus dysgalactiae and DNA aptamer; H, V. parahaemolyticus and DNA aptamer. All the strains are detected under a fluorescence microscope
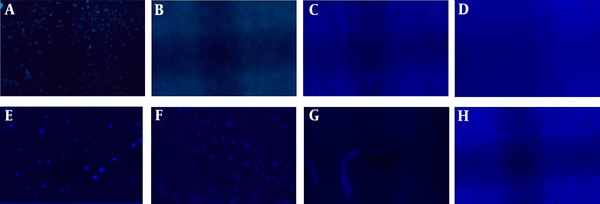
Using VITEK MS as the Diagnostic Gold Standard to Calculate the Accuracy of the AMCA-Modified DNA Aptamer a
VITEK MS | Total | ||
---|---|---|---|
DNA Aptamer | + | - | |
+ | 4 (A) | 3 (B) | 7 |
- | 0 (C) | 43 (D) | 43 |
Total | 4 | 46 |
5. Discussion
The AMCA-modified DNA aptamer has high purity, as evidenced by the single high peak in the adaptor peak spectrum (Figure 1). Additionally, the AMCA-modified DNA aptamer has been successfully synthesized and preserved. To comprehensively assess the ability of the aptamer to detect V. vulnificus in patients with wounds exposed to pathogenic bacteria-contaminated seawater, we collected seawater from two different areas and subjected it to filtration to enhance the detection of pathogenic bacteria. Following the testing of 50 strains, our research showed that our designed fluorescent aptamer exhibited significant sensitivity, specificity, and negative predictive value for the rapid detection of V. vulnificus. However, the positive predictive value was only 57%. Hence, this test proves to be more valuable for ruling out the presence of V. vulnificus rather than confirming its presence.
In clinical samples, several factors can impact the results of these assay types. These factors include the presence of squamous epithelial cells, neutrophil nuclei in secretions, and other substances that can bind to DNA aptamers and induce AMCA fluorescence. Additionally, the number of bacteria in our simulated samples was limited, so real-world samples may contain a wider variety of bacterial species that could potentially affect the accuracy of this assay. Moreover, it is worth noting that microscopic examination as a method has certain limitations, as it necessitates a certain level of professional skill and training to perform accurately.
5.1. Conclusions
In this experiment, we were able to rapidly detect V. vulnificus specimens through a fluorescent reaction within 30 minutes. The number of experimental strains collected was small, particularly with regard to V. vulnificus, so more testing is warranted to confirm the performance of this novel aptamer-based approach.
Acknowledgements
References
-
1.
Cheng D, Ji S, Wang G, Zhu F, Xiao S, Zhu S. Two cases of Vibrio vulnificus primary sepsis. Zhonghua Shao Shang za zhi= Zhonghua Shaoshang Zazhi= Chinese Journal of Burns. 2022;38(3):276-80.
-
2.
Raszl SM, Froelich BA, Vieira CR, Blackwood AD, Noble RT. Vibrio parahaemolyticus and Vibrio vulnificus in South America: water, seafood and human infections. J Appl Microbiol. 2016;121(5):1201-22. [PubMed ID: 27459915]. https://doi.org/10.1111/jam.13246.
-
3.
King NJ, Pirikahu S, Fletcher GC, Pattis I, Roughan B, Perchec Merien A. Correlations between environmental conditions and Vibrio parahaemolyticus or Vibrio vulnificus in Pacific oysters from New Zealand coastal waters. New Zealand Journal of Marine and Freshwater Research. 2020;55(3):393-410. https://doi.org/10.1080/00288330.2020.1796718.
-
4.
Campbell VM, Chouljenko A, Hall SG. Depuration of live oysters to reduce Vibrio parahaemolyticus and Vibrio vulnificus: A review of ecology and processing parameters. Compr Rev Food Sci Food Saf. 2022;21(4):3480-506. [PubMed ID: 35638353]. https://doi.org/10.1111/1541-4337.12969.
-
5.
Metelmann C, Metelmann B, Grundling M, Hahnenkamp K, Hauk G, Scheer C. [Vibrio vulnificus, an increasing threat of sepsis in Germany?]. Anaesthesist. 2020;69(9):672-8. [PubMed ID: 32620988]. https://doi.org/10.1007/s00101-020-00811-9.
-
6.
Nigro OD, James-Davis LI, De Carlo EH, Li YH, Steward GF. Variable freshwater influences on the abundance of Vibrio vulnificus in a tropical urban Estuary. Appl Environ Microbiol. 2022;88(6). e0188421. [PubMed ID: 35196141]. [PubMed Central ID: PMC8939318]. https://doi.org/10.1128/AEM.01884-21.
-
7.
Lorenzoni G, Tedde G, Mara L, Bazzoni AM, Esposito G, Salza S, et al. Presence, Seasonal Distribution, and Biomolecular Characterization of Vibrio parahaemolyticus and Vibrio vulnificus in Shellfish Harvested and Marketed in Sardinia (Italy) between 2017 and 2018. J Food Prot. 2021;84(9):1549-54. [PubMed ID: 33956961]. https://doi.org/10.4315/JFP-21-059.
-
8.
Zhao H, Xu L, Dong H, Hu J, Gao H, Yang M, et al. Correlations between clinical features and mortality in patients with Vibrio vulnificus infection. PLoS One. 2015;10(8). e0136019. [PubMed ID: 26274504]. [PubMed Central ID: PMC4537211]. https://doi.org/10.1371/journal.pone.0136019.
-
9.
Yang X, Zhang X, Wang Y, Shen H, Jiang G, Dong J, et al. A real-time recombinase polymerase amplification method for rapid detection of Vibrio vulnificus in seafood. Front Microbiol. 2020;11:586981. [PubMed ID: 33240242]. [PubMed Central ID: PMC7677453]. https://doi.org/10.3389/fmicb.2020.586981.
-
10.
Boonstra M, Fouz B, van Gelderen B, Dalsgaard I, Madsen L, Jansson E, et al. Fast and accurate identification by MALDI-TOF of the zoonotic serovar E of Vibrio vulnificus linked to eel culture. J Fish Dis. 2023;46(4):445-52. [PubMed ID: 36656662]. https://doi.org/10.1111/jfd.13756.
-
11.
Wangman P, Surasilp T, Pengsuk C, Sithigorngul P, Longyant S. Development of a species‐specific monoclonal antibody for rapid detection and identification of foodborne pathogen Vibrio vulnificus. Journal of Food Safety. 2021;41(6). https://doi.org/10.1111/jfs.12939.
-
12.
Roig AP, Carmona-Salido H, Sanjuan E, Fouz B, Amaro C. Corrigendum to "A multiplex PCR for the detection of Vibrio vulnificus hazardous to human and/or animal health from seafood" [International Journal of Food Microbiology, 377 (2022) 109778]. Int J Food Microbiol. 2023;387:110062. [PubMed ID: 36623955]. https://doi.org/10.1016/j.ijfoodmicro.2022.110062.
-
13.
Rychert J, Slechta ES, Barker AP, Miranda E, Babady NE, Tang YW, et al. Multicenter evaluation of the vitek MS v3.0 system for the identification of filamentous fungi. J Clin Microbiol. 2018;56(2). [PubMed ID: 29142047]. [PubMed Central ID: PMC5786734]. https://doi.org/10.1128/JCM.01353-17.
-
14.
Yoon J, Kim CH, Yoon SY, Lim CS, Lee CK. Application of a multiplex immunochromatographic assay for rapid identification of carbapenemases in a clinical microbiology laboratory: performance and turn-around-time evaluation of NG-test Carba 5. BMC Microbiol. 2021;21(1):260. [PubMed ID: 34587902]. [PubMed Central ID: PMC8482613]. https://doi.org/10.1186/s12866-021-02309-9.
-
15.
Garza-González E, Camacho-Ortíz A, Rodríguez-Noriega E, Esparza-Ahumada S, Flores-Treviño S, Bocanegra-Ibarias P, et al. Comparison of matrix-assisted laser desorption ionization time-of-flight mass spectrometry (MALDI-TOF MS) and the Vitek 2 system for routine identification of clinically relevant bacteria and yeast. Ann Clin Lab Sci. 2020;50(1):119-27.
-
16.
Wilen CB, McMullen AR, Burnham CA. Comparison of sample preparation methods, instrumentation platforms, and contemporary commercial databases for identification of clinically relevant mycobacteria by matrix-assisted laser desorption ionization-time of flight mass spectrometry. J Clin Microbiol. 2015;53(7):2308-15. [PubMed ID: 25972426]. [PubMed Central ID: PMC4473241]. https://doi.org/10.1128/JCM.00567-15.
-
17.
Fan S, Ma C, Tian X, Ma X, Qin M, Wu H, et al. Detection of Vibrio vulnificus in seafood with a dnazyme-based biosensor. Front Microbiol. 2021;12:655845. [PubMed ID: 34149642]. [PubMed Central ID: PMC8213197]. https://doi.org/10.3389/fmicb.2021.655845.
-
18.
Yan W, Gu L, Liu S, Ren W, Lyu M, Wang S. Identification of a highly specific DNA aptamer for Vibrio vulnificus using systematic evolution of ligands by exponential enrichment coupled with asymmetric PCR. J Fish Dis. 2018;41(12):1821-9. [PubMed ID: 30270541]. https://doi.org/10.1111/jfd.12891.
-
19.
Wang Q, Fu S, Yang Q, Hao J, Zhou C, Liu Y. The impact of water intrusion on pathogenic Vibrio Species to inland brackish waters of China. Int J Environ Res Public Health. 2020;17(18). [PubMed ID: 32957572]. [PubMed Central ID: PMC7558382]. https://doi.org/10.3390/ijerph17186781.
-
20.
Fleischmann S, Herrig I, Wesp J, Stiedl J, Reifferscheid G, Strauch E, et al. Prevalence and distribution of potentially human pathogenic vibrio spp. on German north and Baltic sea coasts. Front Cell Infect Microbiol. 2022;12:846819. [PubMed ID: 35937704]. [PubMed Central ID: PMC9355094]. https://doi.org/10.3389/fcimb.2022.846819.
-
21.
Lamon S, Consolati SG, Fois F, Cambula MG, Pes M, Porcheddu G, et al. Occurrence, seasonal distribution, and molecular characterization of Vibrio vulnificus, Vibrio cholerae, and Vibrio parahaemolyticus in shellfish (Mytilus galloprovincialis and Ruditapes decussatus) collected in Sardinia (Italy). J Food Prot. 2019;82(11):1851-6. [PubMed ID: 31603702]. https://doi.org/10.4315/0362-028X.JFP-19-021.