Abstract
Background:
Alterations in cytokine and chemokine levels during SARS-CoV-2 infection may serve as indicators of disease severity.Objectives:
This study aimed to evaluate the levels of plasma cytokines and chemokines in patients with COVID-19.Methods:
The study included 120 COVID-19 patients, divided into severe, mild, and recovered categories (n = 40 for each group). Plasma levels of cytokines such as interleukin 8 (IL-8) and tumor necrosis factor α (TNF-α) were measured using ELISA, while chemokines like monocyte chemoattractant protein 1 (MCP-1) and programmed death protein 1 (PD-1) were quantified through qRT-PCR.Results:
A higher incidence of positive biomarkers IL-8, TNF-α, MCP-1, and PD-1 was observed in the severe group compared to the mild and recovered groups. Notably, the expression levels of PD-1 and MCP-1 were significantly elevated in severely infected individuals relative to those in healthy subjects. A strong positive correlation was also noted between PD-1 and MCP-1 levels in cases of severe infection.Conclusions:
The findings suggest that MCP-1, PD-1, TNF-α, and IL-8 could act as biomarkers for assessing the severity of COVID-19 infections. These results aim to deepen our understanding of the immunopathological mechanisms at play in this disease.Keywords
1. Background
The COVID-19 pandemic, triggered by the SARS-CoV-2 virus, has become a significant global health challenge, causing widespread morbidity and mortality (1). The primary symptoms associated with COVID-19 encompass fever, cough, shortness of breath, muscle aches, and fatigue (2), while less common symptoms may include sputum production, headaches, hemoptysis, and diarrhea. In its severe form, the disease can escalate to infectious pneumonia, potentially leading to acute respiratory distress syndrome (ARDS), cardiac damage, and secondary infections (3). Despite concerted global efforts to curb the spread of this highly contagious virus, there remains an urgent demand for effective diagnostic tools and prognostic markers to better manage and treat those affected.
Numerous studies have indicated that individuals with COVID-19 show increased levels of proinflammatory cytokines in their blood (4). In certain instances, the disease may advance swiftly, resulting in a condition known as a cytokine storm. This condition overwhelms the immune system, leading to a significant decrease in lymphocytes, particularly T cells (5). The disruption of the immune system's overall functionality causes immune cells to release an excessive amount of pro-inflammatory cytokines and chemokines, exacerbating the cytokine storm (6). The overwhelming inflammation induced by the virus in COVID-19 patients is closely linked to the disease's severity and has been identified as a leading cause of death (7).
Chemokines, as inflammatory mediators, are essential in aiding the immune response to infections. Yet, their overproduction is a key factor in hyperinflammation (8). A meta-analysis has shown that severe COVID-19 cases present higher levels of chemokines compared to moderate cases (9). Biomarkers like IP-10 and MCP-1 are associated with the severity of COVID-19 and the mortality risk among those infected (10). Current diagnostic approaches for COVID-19 fall short in identifying mild or asymptomatic cases and in forecasting the progression of the disease.
2. Objectives
This study was conducted to assess the circulating levels of monocyte chemoattractant protein 1 (MCP-1), programmed death protein 1 (PD-1), interleukin 8 (IL-8), and tumor necrosis factor α (TNF-α) as chemokines and cytokines in individuals with severe COVID-19, in comparison to those with mild cases and individuals who have recovered from the disease. These biomarkers are key players in modulating the immune response, cytokine signaling, and inflammation, which are critical factors in the pathogenesis of COVID-19. The identification of reliable biomarkers is essential for the early detection of the disease, risk assessment, and informing treatment strategies.
3. Methods
In this case-control study, carried out between 2019 and 2021, participants were selected from Masih Daneshvari Hospital. The research involved groups of individuals diagnosed with severe COVID-19, those with mild COVID-19, and individuals who had recovered from the disease, with 40 participants in each category (Table 1). The SARS-CoV-2 infection in all participants was verified through viral PCR testing of nasal and pharyngeal swab specimens obtained during the acute phase of the infection, following WHO guidelines.
Briefly Presents the Symptoms of Patients Based on the Severity of the Disease
Disease Severity | Specifications |
---|---|
Mild | Mild clinical signs (fever above 38°C, with or without cough, no dyspnea), no visible sign of pneumonia |
Medium | Fever; Respiratory symptoms, the visible sign of pneumonia |
Severe | Respiratory distress, respiratory rate (RR) more than 30 times per minute-pulse oximetry oxygen saturation level (SPO2) less than 93% at rest- The arterial partial pressure of O2 and the fraction of inspired oxygen (PaO2/FiO2) ratio less than 300 mmHg |
3.1. Enzyme-linked Immunosorbent Assay
Peripheral blood samples were collected from each participant post-diagnosis by a healthcare specialist through standard venipuncture. Serum samples were separated via centrifugation at 1000×g for 15 minutes at 4°C and were then promptly stored at -80°C pending further analysis. The concentrations of soluble IL-8 and TNF-α were quantified by Enzyme-linked Immunosorbent assay (ELISA), following the instructions provided by the kit's manufacturer. The optical density (OD) for IL-8 and TNF-α (both supplied by Carmania Parsgen Co, Iran) was determined spectrophotometrically using a microplate reader at wavelengths of 450 nm and 620 nm, respectively. The quantities of IL-8 and TNF-α were established based on a standard curve.
3.2. qRT-PCR
RNA was extracted from peripheral blood samples utilizing the RNeasy Midi Kit (Qiagen, Cat No.75144) following the manufacturer's guidelines. Subsequently, cDNA was synthesized from the extracted RNA using the Viva 2-step qRT-PCR Kit (Cat No. RTPL12). The expression levels of MCP-1 and PD-1 were quantified via qRT-PCR using CinnaGreen qRT-PCR Mix, 2X (Cat No.MM2041), with 18srRNA serving as the internal control for normalization, as per the manufacturer's instructions. The gene expression data were normalized against 18srRNA and analyzed using the ΔΔCt (2−ΔΔCt) method. The specific primer sequences employed in this expression study are detailed in Table 2.
Parameters | MCP-1 | PD-1 | 18S rRNA |
---|---|---|---|
Forward primer | AGAATCACCAGCAGCAAGTGTCC | CGTGGCCTATCCACTCCTCA | GTAACCCGTTGAACCCCATT |
Reverse primer | TCCTGAACCCACTTCTGCTTGG | ATCCCTTGTCCCAGCCACTC | CCATCCAATCGGTAGTAGCG |
Length of amplified fragment | 98 | 106 | 152 |
3.3. Statistical Analysis
Statistical analyses were conducted using SPSS 24 software. Group differences were assessed using a one-way ANOVA test followed by Tukey's HSD post-hoc analysis. The correlation between variables was examined using Spearman's rank correlation coefficient. The area under the receiver operating characteristics (ROC) curve (AUC) was calculated for the patients, and the optimal cut-off value was identified using Youden’s index. All results are reported as mean ± standard deviation (SD), with P-values of less than 0.05 deemed statistically significant.
4. Results
4.1. Patient Characteristics
The study included 120 individuals diagnosed with COVID-19, categorized into three distinct groups based on the severity of their condition: Severe, mild, and recovered. There were no significant variations in age across these groups. The average ages SD for patients with severe, mild, and recovered COVID-19 were 55.62 (8.22), 54.48 (7.94), and 55.32 (8.88) years, respectively, with statistical analysis indicating no significant differences in age among the groups (P = 0.41). The distribution of males in the severe, mild, and recovered groups was 60%, 62%, and 58%, respectively, showing no significant differences in gender distribution across the groups (P = 0.07).
The biomarkers IL-8, TNF-α, PD-1, and MCP-1 exhibited varying positivity rates across different infection severity groups. Specifically, in the severe infection group, the positivity rates for IL-8, TNF-α, PD-1, and MCP-1 were 92.00%, 90%, 85%, and 80%, respectively. The corresponding positivity rates for the mild infection group were 75%, 78%, 70%, and 60%. In the recovered group, the rates of positive results for IL-8, TNF-α, PD-1, and MCP-1 stood at 28%, 22%, 30%, and 25%, respectively, as depicted in Figure 1. A statistical analysis comparing the positivity rates of these biomarkers among the severe infection, mild infection, and recovered groups, conducted using the two-sample binomial test, indicated a statistically significant difference across the groups (P < 0.001).
Percentage of positive cases for IL-8, TNF-Α, PD-1, and MCP-1 severe infection, mild infection, and recovered groups
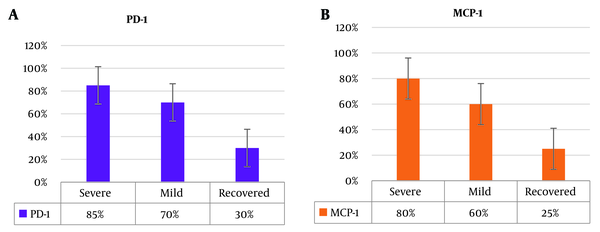
The expression level of PD-1 in individuals with severe infection was significantly elevated, showing a 2.63-fold increase when compared to healthy individuals. In the case of individuals with mild infection, PD-1 levels were increased by 2.29-fold relative to healthy individuals, as depicted in Figure 2. Similarly, the expression level of MCP-1 in those with severe infection was 2.82 times higher than in healthy individuals. MCP-1 levels were 2.14 times higher for individuals with mild infection than those in healthy individuals, as shown in Figure 2. These findings highlight significant differences in the expression levels of PD-1 and MCP-1 across different infection severity groups, underscoring their potential utility as valuable biomarkers for determining the severity of infection.
Expression levels of PD-1 and MCP-1 in severe, mild, and recovered COVID-19 infected patients were measured by qRT-PCR
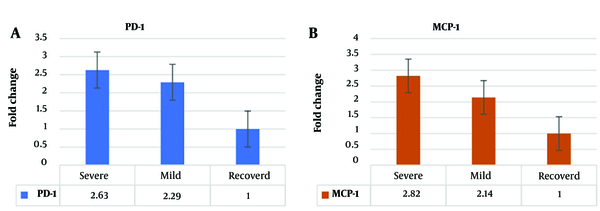
We evaluated the correlation to determine any association between cytokines/chemokines and disease severity. A strong positive linear relationship was observed between PD-1 and MCP-1 in patients with severe infections (r = 0.64, P < 0.05), indicating a significant association. However, no correlation was found between PD-1 and MCP-1 in patients with mild and recovered COVID-19 infections. Similarly, no correlation was observed between IL-8 and TNF-α across severe, mild, and recovered COVID-19 infection groups. The diagnostic utility of cytokines and chemokines in determining illness severity was further analyzed using the receiver operating characteristic (ROC) curve and the area under the ROC curve (AUC) as shown in Figure 3. The AUC for ROC values for MCP-1, PD-1, TNF-α, and IL-8 were 0.90, 0.91, 0.92, and 0.93, respectively, suggesting that these cytokines could effectively predict the severity of COVID-19 infections (Figure 3). Our data suggest that these cytokines and chemokines may be biomarkers for disease severity in COVID-19-infected patients.
ROC curves of MCP-1, PD-1, TNF-α, and IL-8 in serum of COVID-19 patients
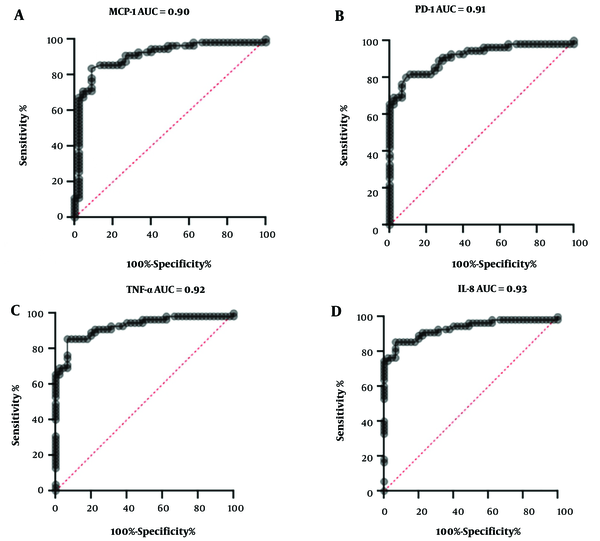
5. Discussion
COVID-19 manifests in a spectrum ranging from asymptomatic cases and spontaneous recovery to severe ARDS, characterized by respiratory failure and lung damage. Identifying individuals at high risk of adverse outcomes and pinpointing potential risk factors and biomarkers for severe illness is crucial. To this end, we utilized ELISA and qRT-PCR analyses on the serum of COVID-19 patients. Our findings indicated that patients with severe infections exhibited a higher percentage of positive results for IL-8, TNF-α, PD-1, and MCP-1 compared to those with mild infections or those who had recovered. Subsequent statistical analysis using ROC curves suggested that these cytokines and chemokines could serve as important indicators for assessing the severity of COVID-19 in affected individuals.
Numerous research studies have indicated that patients with severe cases of COVID-19 often exhibit significant increases in the levels of proinflammatory cytokines in their blood (6, 11). While the exact mechanisms of how cytokines communicate in COVID-19 patients remain largely unknown, there have been growing achievements in utilizing cytokines or cytokine inhibitors as therapeutic interventions (12, 13). Interleukin 8 is a chemokine released by various cell types, including endothelial cells, epithelial cells, macrophages, and monocytes. It acts on leukocytes such as neutrophils and natural killer cells, inducing chemotaxis, degranulation, and the production of reactive oxygen species (14). The accumulation of activated neutrophils, which can cause damage to lung tissue, has been proposed as a mechanism contributing to ARDS (14).
Elevated levels of IL-8 in the bloodstream have been linked to a longer duration of illness in patients with severe or critical COVID-19 (15). IL-8 has also been associated with the recruitment and activation of polymorphonuclear-myeloid-derived suppressor cells (PMN-MDSC), which inhibit the response of T-cells to the SARS-CoV-2 virus (16). Furthermore, the frequency of PMN-MDSCs is higher in non-survivors compared to survivors of critical COVID-19, and their frequency is positively correlated with plasma levels of IL-8 in hospitalized COVID-19 patients (16). These findings suggest that IL-8 signaling plays a crucial role in the disease progression, revealing potential therapeutic strategies for treating COVID-19 (17).
Our findings demonstrated that severe COVID-19 patients had higher levels of MCP-1 in their peripheral blood cells than those with mild symptoms or who had recovered. Previous studies on severe cases of SARS-CoV have also shown that elevated MCP-1 secretion is associated with lung injury and the need for intensive care unit (ICU) admission (6, 18-20). Additionally, MCP-1 is a parameter linked to thrombosis, which suggests it may be associated with an increased risk of mortality in COVID-19 patients (20). Consistent with our results, Huang et al. reported a significant increase in serum levels of proinflammatory cytokines, particularly MCP-1, in patients infected with 2019-nCoV, indicating its potential role in activating the T-helper-1 (Th1) cell response.
We found that serum tumor necrosis factor α (TNF-α) levels were elevated in COVID-19 patients with severe disease and adverse prognosis. This finding aligns with previous research indicating that cytokine release syndrome (CRS) is strongly associated with disease severity, characterized by increased serum levels of TNF-α, granulocyte-colony stimulating factor (G-CSF), interferon gamma-induced protein 10 (IP-10), monocyte chemoattractant protein 1 (MCP-1), and macrophage inflammatory protein 1α (MIP1A) (6, 21). An increase in TNF-α can potentially facilitate viral infection and cause damage to organs (22). While TNF-α plays a role in coordinating the inflammatory response during the acute phase of inflammation, excessive TNF-α can suppress the immune system, leading to adverse outcomes (23, 24).
Moreover, upregulation of PD-1 in T-cells was detected during the progression of symptomatic stages of COVID-19 (25). This upregulation of PD-1 has been found to contribute to developing the severe form of the disease (26). Consistent with this, our study revealed an increased level of PD-1 in patients experiencing severe symptoms, suggesting a potential association between PD-1 and the severity of COVID-19. SARS-CoV-2 triggers lymphocyte depletion and exhaustion in infected individuals. This is primarily characterized by the expression of programmed death inhibitory receptor 1 (PD-1) and programmed death ligand 1 (PDL-1) (27, 28), which play crucial roles in central and peripheral immune tolerance and exhaustion.
Regarding the correlation analysis, a strong positive relationship was observed between PD-1 and MCP-1 in severe COVID-19 patients. Studies have indicated that COVID-19 patients exhibit increased expressions of PD-1 in both CD4+ and CD8+ T cells. This suggests the involvement of these regulatory molecules in the apoptosis of antigen-activated T cells during COVID-19, resulting in a decrease in CD4+ T cell numbers and a reduction in the proportion of naive T cells (29). On the other hand, there is evidence indicating a rise in the population of monocytes, neutrophils, and natural killer (NK) cells, triggering a cytokine storm (30, 31). Additionally, MCP-1 is implicated in the pathogenesis of diseases characterized by the infiltration of monocytes (32). Based on these findings, it is plausible to speculate that MCP-1 and PD-1 may be associated with the severity of COVID-19.
5.1. Conclusions
The study has limitations due to a small sample size and the lack of analysis of inflammatory mediators over time. More multi-center studies are needed to verify the results and provide a comprehensive interpretation. However, the study supports the association of IL-8, TNF-α, PD-1, and MCP-1 with the severity of COVID-19. It is important to understand the specific functions of these cytokines and evaluate treatment consequences before considering modulating therapy. Our data suggest that modulators of elevated cytokines/chemokines may offer potential precision medical treatments for COVID-19. The results suggest that MCP-1, PD-1, TNF-α, and IL-8 may be biomarkers for disease severity in COVID-19-infected patients. These findings are expected to enhance our comprehension of the immunopathological mechanisms associated with this emerging disease.
References
-
1.
World Health Organization. Number of COVID-19 cases reported to WHO. 2023. Available from: https://data.who.int/dashboards/covid19/cases?n=c.
-
2.
Yang X, Yu Y, Xu J, Shu H, Xia J, Liu H, et al. Clinical course and outcomes of critically ill patients with SARS-CoV-2 pneumonia in Wuhan, China: a single-centered, retrospective, observational study. Lancet Respir Med. 2020;8(5):475-81. [PubMed ID: 32105632]. [PubMed Central ID: PMC7102538]. https://doi.org/10.1016/S2213-2600(20)30079-5.
-
3.
Ruan Q, Yang K, Wang W, Jiang L, Song J. Clinical predictors of mortality due to COVID-19 based on an analysis of data of 150 patients from Wuhan, China. Intensive Care Med. 2020;46(5):846-8. [PubMed ID: 32125452]. [PubMed Central ID: PMC7080116]. https://doi.org/10.1007/s00134-020-05991-x.
-
4.
Broman N, Rantasarkka K, Feuth T, Valtonen M, Waris M, Hohenthal U, et al. IL-6 and other biomarkers as predictors of severity in COVID-19. Ann Med. 2021;53(1):410-2. [PubMed ID: 33305624]. [PubMed Central ID: PMC7935117]. https://doi.org/10.1080/07853890.2020.1840621.
-
5.
Zhang B, Zhou X, Zhu C, Song Y, Feng F, Qiu Y, et al. Immune phenotyping based on the neutrophil-to-lymphocyte ratio and IgG level predicts disease severity and outcome for patients with COVID-19. Front Mol Biosci. 2020;7:157. [PubMed ID: 32719810]. [PubMed Central ID: PMC7350507]. https://doi.org/10.3389/fmolb.2020.00157.
-
6.
Huang C, Wang Y, Li X, Ren L, Zhao J, Hu Y, et al. Clinical features of patients infected with 2019 novel coronavirus in Wuhan, China. Lancet. 2020;395(10223):497-506. [PubMed ID: 31986264]. [PubMed Central ID: PMC7159299]. https://doi.org/10.1016/S0140-6736(20)30183-5.
-
7.
McGonagle D, Sharif K, O'Regan A, Bridgewood C. The role of cytokines including interleukin-6 in COVID-19 induced pneumonia and macrophage activation syndrome-like disease. Autoimmun Rev. 2020;19(6):102537. [PubMed ID: 32251717]. [PubMed Central ID: PMC7195002]. https://doi.org/10.1016/j.autrev.2020.102537.
-
8.
Khalil BA, Elemam NM, Maghazachi AA. Chemokines and chemokine receptors during COVID-19 infection. Comput Struct Biotechnol J. 2021;19:976-88. [PubMed ID: 33558827]. [PubMed Central ID: PMC7859556]. https://doi.org/10.1016/j.csbj.2021.01.034.
-
9.
Zhang Z, Ai G, Chen L, Liu S, Gong C, Zhu X, et al. Associations of immunological features with COVID-19 severity: a systematic review and meta-analysis. BMC Infect Dis. 2021;21(1):738. [PubMed ID: 34344353]. [PubMed Central ID: PMC8329624]. https://doi.org/10.1186/s12879-021-06457-1.
-
10.
Chen Y, Wang J, Liu C, Su L, Zhang D, Fan J, et al. IP-10 and MCP-1 as biomarkers associated with disease severity of COVID-19. Mol Med. 2020;26(1):97. [PubMed ID: 33121429]. [PubMed Central ID: PMC7594996]. https://doi.org/10.1186/s10020-020-00230-x.
-
11.
Meftahi GH, Jangravi Z, Sahraei H, Bahari Z. The possible pathophysiology mechanism of cytokine storm in elderly adults with COVID-19 infection: the contribution of "inflame-aging". Inflamm Res. 2020;69(9):825-39. [PubMed ID: 32529477]. [PubMed Central ID: PMC7289226]. https://doi.org/10.1007/s00011-020-01372-8.
-
12.
Ferrari SM, Fallahi P, Elia G, Ragusa F, Camastra S, Paparo SR, et al. Novel therapies for thyroid autoimmune diseases: An update. Best Pract Res Clin Endocrinol Metab. 2020;34(1):101366. [PubMed ID: 31813786]. https://doi.org/10.1016/j.beem.2019.101366.
-
13.
Fallahi P, Elia G, Ragusa F, Ruffilli I, Camastra S, Giusti C, et al. The aggregation between AITD with rheumatologic, or dermatologic, autoimmune diseases. Best Pract Res Clin Endocrinol Metab. 2019;33(6):101372. [PubMed ID: 31932147]. https://doi.org/10.1016/j.beem.2019.101372.
-
14.
Russo RC, Garcia CC, Teixeira MM, Amaral FA. The CXCL8/IL-8 chemokine family and its receptors in inflammatory diseases. Expert Rev Clin Immunol. 2014;10(5):593-619. [PubMed ID: 24678812]. https://doi.org/10.1586/1744666X.2014.894886.
-
15.
Ma A, Zhang L, Ye X, Chen J, Yu J, Zhuang L, et al. High levels of circulating IL-8 and soluble IL-2R are associated with prolonged illness in patients with severe COVID-19. Front Immunol. 2021;12:626235. [PubMed ID: 33584733]. [PubMed Central ID: PMC7878368]. https://doi.org/10.3389/fimmu.2021.626235.
-
16.
Sacchi A, Grassi G, Bordoni V, Lorenzini P, Cimini E, Casetti R, et al. Early expansion of myeloid-derived suppressor cells inhibits SARS-CoV-2 specific T-cell response and may predict fatal COVID-19 outcome. Cell Death Dis. 2020;11(10):921. [PubMed ID: 33110074]. [PubMed Central ID: PMC7590570]. https://doi.org/10.1038/s41419-020-03125-1.
-
17.
Cesta MC, Zippoli M, Marsiglia C, Gavioli EM, Mantelli F, Allegretti M, et al. The role of interleukin-8 in lung inflammation and injury: Implications for the management of COVID-19 and hyperinflammatory acute respiratory distress syndrome. Front Pharmacol. 2021;12:808797. [PubMed ID: 35095519]. [PubMed Central ID: PMC8790527]. https://doi.org/10.3389/fphar.2021.808797.
-
18.
Wong CK, Lam CW, Wu AK, Ip WK, Lee NL, Chan IH, et al. Plasma inflammatory cytokines and chemokines in severe acute respiratory syndrome. Clin Exp Immunol. 2004;136(1):95-103. [PubMed ID: 15030519]. [PubMed Central ID: PMC1808997]. https://doi.org/10.1111/j.1365-2249.2004.02415.x.
-
19.
Cheung CY, Poon LL, Ng IH, Luk W, Sia SF, Wu MH, et al. Cytokine responses in severe acute respiratory syndrome coronavirus-infected macrophages in vitro: possible relevance to pathogenesis. J Virol. 2005;79(12):7819-26. [PubMed ID: 15919935]. [PubMed Central ID: PMC1143636]. https://doi.org/10.1128/JVI.79.12.7819-7826.2005.
-
20.
Cheng C, Zhang D, Dang D, Geng J, Zhu P, Yuan M, et al. The incubation period of COVID-19: a global meta-analysis of 53 studies and a Chinese observation study of 11 545 patients. Infect Dis Poverty. 2021;10(1):119. [PubMed ID: 34535192]. [PubMed Central ID: PMC8446477]. https://doi.org/10.1186/s40249-021-00901-9.
-
21.
Chen L, Liu HG, Liu W, Liu J, Liu K, Shang J, et al. Analysis of clinical features of 29 patients with 2019 novel coronavirus pneumonia. Zhonghua jie he he hu xi za zhi= Zhonghua jiehe he huxi zazhi= Chinese journal of tuberculosis and respiratory diseases. 2020;43:E005.
-
22.
Deng X, Yu X, Pei J. Regulation of interferon production as a potential strategy for COVID-19 treatment. arXiv preprint arXiv:2003.00751. 2020.
-
23.
Clark J, Vagenas P, Panesar M, Cope AP. What does tumour necrosis factor excess do to the immune system long term? Ann Rheum Dis. 2005;64 Suppl 4(Suppl 4):iv70-6. [PubMed ID: 16239393]. [PubMed Central ID: PMC1766919]. https://doi.org/10.1136/ard.2005.042523.
-
24.
Guo Y, Hu K, Li Y, Lu C, Ling K, Cai C, et al. Targeting TNF-alpha for COVID-19: Recent advanced and controversies. Front Public Health. 2022;10:833967. [PubMed ID: 35223745]. [PubMed Central ID: PMC8873570]. https://doi.org/10.3389/fpubh.2022.833967.
-
25.
Diao B, Wang C, Tan Y, Chen X, Liu Y, Ning L, et al. Reduction and functional exhaustion of T cells in patients with coronavirus disease 2019 (COVID-19). Frontiers in immunology. 2020. https://doi.org/10.1101/2020.02.18.20024364.
-
26.
Zheng HY, Zhang M, Yang CX, Zhang N, Wang XC, Yang XP, et al. Elevated exhaustion levels and reduced functional diversity of T cells in peripheral blood may predict severe progression in COVID-19 patients. Cell Mol Immunol. 2020;17(5):541-3. [PubMed ID: 32203186]. [PubMed Central ID: PMC7091621]. https://doi.org/10.1038/s41423-020-0401-3.
-
27.
Beserra DR, Alberca RW, Branco A, de Mendonca Oliveira L, de Souza Andrade MM, Gozzi-Silva SC, et al. Upregulation of PD-1 expression and high sPD-L1 levels associated with COVID-19 severity. J Immunol Res. 2022;2022:9764002. [PubMed ID: 35971391]. [PubMed Central ID: PMC9375698]. https://doi.org/10.1155/2022/9764002.
-
28.
Sakuishi K, Apetoh L, Sullivan JM, Blazar BR, Kuchroo VK, Anderson AC. Targeting Tim-3 and PD-1 pathways to reverse T cell exhaustion and restore anti-tumor immunity. J Exp Med. 2010;207(10):2187-94. [PubMed ID: 20819927]. [PubMed Central ID: PMC2947065]. https://doi.org/10.1084/jem.20100643.
-
29.
Chen Z, John Wherry E. T cell responses in patients with COVID-19. Nat Rev Immunol. 2020;20(9):529-36. [PubMed ID: 32728222]. [PubMed Central ID: PMC7389156]. https://doi.org/10.1038/s41577-020-0402-6.
-
30.
Fajgenbaum DC, June CH. Cytokine Storm. N Engl J Med. 2020;383(23):2255-73. [PubMed ID: 33264547]. [PubMed Central ID: PMC7727315]. https://doi.org/10.1056/NEJMra2026131.
-
31.
Blanco-Melo D, Nilsson-Payant BE, Liu WC, Uhl S, Hoagland D, Moller R, et al. Imbalanced host response to SARS-CoV-2 drives development of COVID-19. Cell. 2020;181(5):1036-1045 e9. [PubMed ID: 32416070]. [PubMed Central ID: PMC7227586]. https://doi.org/10.1016/j.cell.2020.04.026.
-
32.
Deshmane SL, Kremlev S, Amini S, Sawaya BE. Monocyte chemoattractant protein-1 (MCP-1): an overview. J Interferon Cytokine Res. 2009;29(6):313-26. [PubMed ID: 19441883]. [PubMed Central ID: PMC2755091]. https://doi.org/10.1089/jir.2008.0027.