Abstract
Background:
Biodegradable polyesters are candidates for the development of environmental friendly plastics. Poly-β-hydroxybutyrate (PHB) is a type of polyester from the hydroxyalkanoates family, synthesized by bacteria as an intracellular material and accumulated as granules in the cytoplasm.Objectives:
The aim of this study was to isolate Poly β-hydroxybutyrate over producing bacteria and optimize the production medium.Materials and Methods:
A variety of PHB-accumulating bacterial strains were isolated from Kermanshah oil refinery sludge in Iran. Poly-β-hydroxybutyrate-producing bacterial strains were confirmed by the gas chromatography method. The strain with the highest rate of PHB production was selected. Use of sugar cane molasses, a by-product of the sugar refinery industry, was investigated for the production of PHB. Plackett-Burman statistical method was employed to obtain factors in cell growth and PHB production. Optimization by the Response Surface Method (RSM) was done via two carbon sources, glucose and molasses.Results:
In the present study, 21 of 63 strains isolated from the refinery oil sludge produced PHB, seven of which were over producers. Poly-β-hydroxybutyrate production was analyzed by Sudan Black B staining, spectrophotometric and gas chromatography (GC) methods. The strain with the highest rate of PHB production was used to optimize the culture medium. Fifteen factors were analyzed in PHB production by the Plackett-Burman method to find the most effective factors. Five important factors were optimized by RSM. Molasses were used as a cheap source of carbon. The maximum PHB obtained from molasses was 6.62 g/L. The phenotypic and 16S rRNA biotyping tests led to the identification of the isolate as Bacillus coagulans.Conclusions:
The results suggest that B. coagulans is a good candidate for the fermentative production of PHB.Keywords
β-hydroxybutyrate Beta Hydroxybutyrate Fermentation Biodegradation Plastics
1. Background
Plastics are widely used in packaging, building materials and commodities (1). Plastics are non-biodegradable petroleum derivatives considered as environmentally harmful wastes (2). In the recent years, biodegradable plastics have received attention for environmental compatibility and can be produced from renewable resources using various procedures (3). Among these biologically originated and biodegradable polymers, the family of polyhydroxyalkanoates (PHAs) is one of the best-studied cases. Polyhydroxyalkanoates are polyesters accumulated as intracellular granules by many microorganisms and act as supplies of carbon or energy (4). Poly-β-hydroxybutyrate (PHB) is the best studied and characterized PHA isolated and characterized for the first time by French microbiologist Maurice Lemoigne in 1923 (5). Poly-β-hydroxybutyrate is a biodegradable thermoplastic polyester applicable in almost all industries such as medicine, agriculture, etc. (6). This polymer can be used to encapsulate proteins, drugs and enzymes, providing sustained release and protection of unreleased protein from degradation (7). The major problem preventing the commercial application of PHBs is their high price. One of the requirements for decreasing the production costs is the development of better PHB-producing bacterial strains (8).
During oil production and processing, significant quantities of oily sludge are generated. The oil sludge, frequently present in oil products stored in tanks, contains different concentrations of waste oil (40 - 60%), waste water (30 - 90%) and mineral particles (5 - 40%) (5). Accumulation of PHB under aerobic conditions often occurs when the carbon source is in excess, but one or several other nutrients are limited. The oil sludge governs the conditions by providing excess carbon source and therefore, can be a good source for the accumulation of this polymer by bacteria. The price of PHB depends mainly on substrate cost, which accounts for about 40% of the total production costs (3). There are plenty of low-cost, renewable, carbon-containing raw materials available around the world. Sugarcane molasses are one such renewable agricultural commodity. Molasses contain vitamins and other minor constituents that can be used as sources of carbon for PHB production (9).
One solution for the problem of high production cost is the optimization of cultivation media. Medium optimization for over production of any metabolite is an important step for its commercial use and involves a number of physicochemical parameters such as the composition of the medium, the carbon and nitrogen sources, minerals and trace metals, pH, temperature, aeration and inoculum age. Optimization has been studied widely and typical optimization methods involve changing one factor or varying several factors at the same time (10). Statistical modeling of experiments has been used in many studies. Application of statistical methods involving the Plackett-Burman Design (PBD) has gained a lot of impetus for medium optimization and understanding the interactions among various physicochemical parameters (11). Response Surface Methodology (RSM) is a collection of mathematical and statistical techniques widely used to determine the effects of several variables and to optimize different biotechnological processes (5). Some studies have reported the use of RSM by different bacterial strains such as Rhodobacter sphaeroides and R. eutropha to increase PHB production (12).
2. Objectives
Biodegradable plastics can be produced from renewable resources. Many microorganisms accumulate PHAs in the form of intracellular granules as carbon supplies, exploitation of which could contribute significantly to maintenance of clean and green environment. This study deals with the isolation of poly-β-hydroxybutyrate producing bacterial strains and use of sugar cane molasses, a by-product of the sugar refinery industry for its production.
3. Materials and Methods
3.1. Sample Collection
Oily sludge samples were collected from the oil refinery of Kermanshah, Iran. The sampling was done through the terminal valve of the petroleum reservoirs after letting the sludge settle down. The sludge samples were collected directly from 20 tanks and poured in a 20-liter can and used throughout the study. The sample was stored at 4°C in the dark.
3.2. Isolation of Bacteria
Firstly, 20 mg of the oily sludge was mixed with Tween 80 and transferred into Luria Bertani (LB) broth (Merck, Germany) and incubated at 30°C for 24 hours. After 24 hours, 0.1 mL of the growth medium was transferred to LB agar medium and bacterial isolation was carried out by repetitive culturing on LB agar plates.
3.3. Observation of Poly-β-hydroxybutyrate Granules
Sudan Black B (Merck, Germany), as a dye with particular high affinity for PHB granules, was used to screen the PHB-producing bacterial strains (presumptive test) (13).
3.4. Quantitative Analysis of Poly-β-hydroxybutyrate
The quantitative assessment process was performed by Ultra Violet (UV) spectrophotometry (PG Instrument, UK) for the strains (14). Positive bacterial colonies were selected for quantitative analysis. A loop of bacterial colonies was transferred to 50 mL of Mineral Salt Medium (MSM) (15) in 250 mL Erlenmeyer, and incubated on a rotary shaker incubator at 30°C and 150 rpm for 48 hours (16). In order to determine the maximum PHB production time, the dry weight of PHB production was simultaneously checked. Quantitative measurement was done for 24 - 84 hours by digesting the bacterial cells with 30% sodium hypochlorite solution at 37°C for 20 minutes, and separation of the residue by centrifugation at 8000 × g for 20 minutes. The residue was then successively washed with water, acetone and ethanol, dissolved in chloroform and was kept at 30°C for complete evaporation. Next, 5 mL of concentrated H2SO4 was added and heated for 40 minutes at 100°C in a water bath. The PHB was acidified with sulfuric acid for crotonic acid formation. Finally, the solution was analyzed by UV spectrophotometer at 235 nm.
3.5. Gas Chromatography (GC)
Gas chromatography is generally used for rapid determination of PHB present in cells. For this purpose 20 mg of PHB standard from Sigma-Aldrich was used, and the methyl esters of the 3-hydroxybutyrate were prepared as described by Lageveen et al. (16). Suspension of 20 mg of lyophilized cells was made in 2 mL of methanol (Merck, Germany) containing 7% (v/v) concentrated H2SO4 (Merck, Germany), in a tube with a Teflon-lined cap. Benzoic acid (Merck, Germany) was added as an internal standard. After addition of 4 mL of chloroform (Merck, Germany), the mixture was incubated for 2.5 hours in a water bath at 100°C. The tubes were cooled down to room temperature. Furthermore, 4 mL of distilled water was added to the tubes, shaken for one minute and rapidly transferred on ice. The bottom organic layer containing PHB was later dried over sodium sulfate (Merck, Germany) before analysis. The organic phase, i.e. methyl esters, extracted by centrifugation at 4500 × g for five minutes, was analyzed by a gas chromatograph (Varian CP3800) equipped with a Flame Ionization Detector (FID) detector. The capillary column was a HP-5 from Agilent J & W Scientific, 30 m in length with 0.25 mm internal diameter. The injection split ratio was 120:1. The injection port and the detector temperatures were 180°C and 200°C, respectively. The initial oven temperature was maintained for one minute at 90°C with an increase of 8°C/minute to a final temperature of 150°C (maintained for five minutes). The flow rate of the helium carrier gas was 1 mL/minute. The PHB polymer (Sigma) was used as an external standard.
3.6. Determination of Cell Dry Weight
The cell dry weight was measured by centrifugation with 100 mL of the culture at 5000 × g for 15 minutes at 4°C. The cell pellet was washed in deionized water and dried at 80°C until a constant weight was achieved.
3.7. Plackett-Burman Design
Plackett-Burman Design (PBD) was employed for selection of significant variables in PHB production. Application of statistical methods involving Plackett-Burman Design (PBD) has gained a lot of impetus for medium optimization (11). Each independent variable was tested at two levels, a high (+1) level and a low (-1) level. The significant principal effects of each variable on PHB production were determined via the P-value.
3.8. Optimization by Response Surface Methodology (RSM)
Design Expert 7.1.6 software was used for medium optimization using the Box Behnken design. The software Design Expert was used for regression analysis of experimental data and also to plot the response surface graphs. Analysis of Variance (ANOVA) was used to estimate the statistical parameters. The studied response was PHB (g/L).
3.9. Characterization of the Isolated Bacteria
Pure isolates containing PHB were investigated based on their microscopic, morphological and biochemical properties according to Bergey’s Manual of Determinative Bacteriology (17).
3.10. 16S rDNA 16S rRNA Gene Sequence Analysis
The genomic DNA was extracted from isolated culture using the genomic DNA Extraction kit (Bioneer, Korea). The sequences were amplified using primers 27 F (5-AGA GTT TGA TCM TGG CTC AG-3) and 1492 R (5-TAC GGY TAC CTT GTT ACG ACT T-3) (18). The Polymerase Chain Reaction (PCR) mixture contained 2 μL of purified DNA template, 1.5 μL of each primer, 2 μL of deoxynucleoside triphosphates (dNTPs), 0.2 μL of Taq DNA polymerase (5 U/μL), 2 μL of MgCl2, 2 μL PCR buffer and 9 μL deionized water. The thermal cycling program used was as follows: initial denaturation at 94°C for five minutes, 35 cycles consisting of 94°C for one minute, 61°C for one minute, and 72°C for one minute, and a final extension step at 72°C for 1.5 minutes. The amplified PCR products were analyzed by electrophoresis (Bio Rad, USA). The sequences of the partial 16S rDNA were compared with the 16S rDNA sequences available in the public nucleotide databases at the National Center for Biotechnology Information (NCBI).
4. Results
4.1. Screening of Bacteria Accumulating Polyhydroxyalkanoates
Sixty-three bacterial strains were isolated from the oily sludge sample. Twenty-one of the colonies exhibited the ability to produce PHAs inclusion bodies.
4.2. Quantitative Analysis of Poly-β-hydroxybutyrate
Comparison of cell growth and PHB content ended up in selection of seven bacterial strains with high PHB content. An effective producer of PHB was chosen based on the dry weight of the extracted PHB. Poly-β-hydroxybutyrate yields of 0.98, 1.78, 2.18 and 1.98 mg/mL were achieved after 48, 60, 72 and 84 hours. Maximum PHB synthesis occurred at 72 hours.
4.3. Gas Chromatography (GC)
Gas chromatography results (Figure 1) showed that the seven bacterial strains could accumulate PHB. Gas chromatography is used for rapid determination of the amount of PHA in cells and identification of the constituents of different hydroxyalkanoic acids. Results of GC showed that seven bacterial strains could accumulate PHB. Gas chromatography analysis confirmed that strain No. 43 produced high amounts of PHB.
Chromatogram of Strain 43 Confirming High Production of Poly-β-hydroxybutyrate
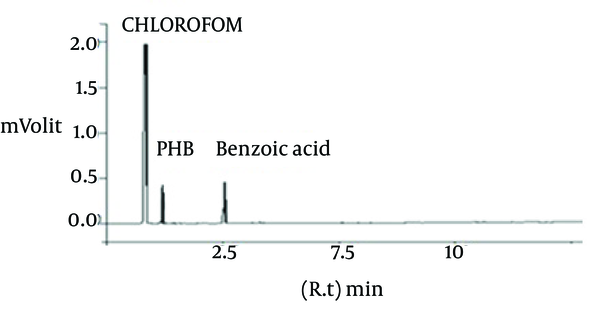
4.4. Plackett-Burman (PB) Experimental Design
Plackett-Burman design was used to identify significant variables effective on PHB production. The PB experimental design for 20 trials with two levels of each variable is shown in Table 1. The significant factors and levels (g/L) of variables for Box Behnken design, each of which were assessed at three coded levels of -1, 0 and +1, were 10, 20 and 30 g/L for utilized carbon sources, 1, 2 and 3 g/L for (NH4)2SO4, 0.1, 0.2 and 0.3 g/L for FeSO4., 0.1, 0.35 and 0.6 g/L for CuSO4, and 1, 2 and 3 g/L for KH2PO4.
Twenty-Trial Plackett-Burman Design Matrix for Fifteen Variables Predicted for Poly-β-hydroxybutyrate Production
Experimental Values | ||||||||||||||||
---|---|---|---|---|---|---|---|---|---|---|---|---|---|---|---|---|
Run | pH | Temperature, °C | Centrifugation rate, rpm | Inoculum age, h | Inoculum, mL | MgSO4 a | CuSO4 a | FeSO4 a | CaCl2 a | Glucose a | KH2PO4 a | Na2HPO4 a | (NH4)2SO4 a | ZnSO4 a | Volume, mL | PHB a |
1 | 9 | 25 | 200 | 12 | 10 | 0.0 | 0.0 | 0.0 | 0.1 | 10 | 1 | 0 | 2 | 0.1 | 100 | 0.090 |
2 | 9 | 35 | 100 | 24 | 10 | 0.0 | 0.0 | 0.0 | 0.0 | 30 | 0 | 2 | 0 | 0.1 | 100 | 0.870 |
3 | 7 | 35 | 200 | 24 | 5 | 0.5 | 0.0 | 0.0 | 0.0 | 10 | 1 | 0 | 2 | 0.0 | 100 | 0.940 |
4 | 7 | 25 | 200 | 12 | 10 | 0.5 | 0.1 | 0.0 | 0.0 | 10 | 0 | 2 | 0 | 0.1 | 50 | 0.070 |
5 | 9 | 25 | 100 | 24 | 10 | 0.0 | 0.1 | 0.2 | 0.0 | 10 | 0 | 0 | 2 | 0.0 | 100 | 1.350 |
6 | 9 | 35 | 100 | 24 | 5 | 0.5 | 0.0 | 0.2 | 0.1 | 10 | 0 | 0 | 0 | 0.1 | 50 | 0.050 |
7 | 9 | 35 | 200 | 12 | 5 | 0.5 | 0.1 | 0.0 | 0.1 | 30 | 0 | 0 | 0 | 0.0 | 100 | 1.890 |
8 | 9 | 35 | 200 | 12 | 10 | 0.0 | 0.1 | 0.2 | 0.0 | 30 | 1 | 0 | 0 | 0.0 | 50 | 3.090 |
9 | 7 | 35 | 200 | 24 | 10 | 0.0 | 0.0 | 0.2 | 0.1 | 10 | 1 | 2 | 0 | 0.0 | 50 | 1.660 |
10 | 9 | 25 | 200 | 24 | 10 | 0.5 | 0.0 | 0.0 | 0.1 | 30 | 0 | 2 | 2 | 0.0 | 50 | 1.950 |
11 | 7 | 35 | 100 | 24 | 10 | 0.5 | 0.1 | 0.0 | 0.0 | 30 | 1 | 0 | 2 | 0.1 | 50 | 4.350 |
12 | 9 | 25 | 200 | 24 | 5 | 0.5 | 0.1 | 0.2 | 0.0 | 10 | 1 | 2 | 0 | 0.1 | 100 | 1.280 |
13 | 7 | 35 | 100 | 12 | 10 | 0.5 | 0.1 | 0.2 | 0.1 | 10 | 0 | 2 | 2 | 0.0 | 100 | 1.650 |
14 | 7 | 25 | 200 | 24 | 5 | 0.0 | 0.1 | 0.2 | 0.1 | 30 | 0 | 0 | 2 | 0.1 | 50 | 3.670 |
15 | 7 | 25 | 100 | 12 | 10 | 0.5 | 0.0 | 0.2 | 0.1 | 30 | 1 | 0 | 0 | 0.1 | 100 | 1.990 |
16 | 7 | 25 | 100 | 24 | 5 | 0.0 | 0.1 | 0.0 | 0.1 | 30 | 1 | 2 | 0 | 0.0 | 100 | 2.340 |
17 | 9 | 25 | 100 | 12 | 5 | 0.5 | 0.0 | 0.2 | 0.0 | 30 | 1 | 2 | 2 | 0.0 | 50 | 3.350 |
18 | 9 | 35 | 100 | 12 | 5 | 0.0 | 0.1 | 0.0 | 0.1 | 10 | 1 | 2 | 2 | 0.1 | 50 | 1.870 |
19 | 7 | 35 | 200 | 12 | 5 | 0.0 | 0.0 | 0.2 | 0.0 | 30 | 0 | 2 | 2 | 0.1 | 100 | 2.570 |
20 | 7 | 25 | 100 | 12 | 5 | 0.0 | 0.0 | 0.0 | 0.0 | 10 | 0 | 0 | 0 | 0.0 | 50 | 0.009 |
The experimental results of PHB production by a complete five factors three levels factorial experiment design with six central points are shown in Table 2. The experiment was done with glucose and then with molasses. The adequacy of the model was calculated, and the effects of the variables on the response and significant levels were screened via Student’s t-test for ANOVA (Table 3). Factors with P-values of less than 0.05 (P < 0.05) were considered to have significant effects and were therefore selected for further optimization studies. Based on the statistical analysis, the factors with the greatest positive impacts on the production of PHB were identified as CuSO4, FeSO4, Glucose, KH2PO4, and (NH4)2SO4. A P-value of 0.001 indicated that glucose had the most significant positive effect on PHB production followed by (NH4)2SO4 (P = 0.011), FeSO4 (P = 0.014), CuSO4 (P = 0.023) and KH2PO4 (P = 0.031). Factors such as MgSO4, temperature, inoculum, inoculum age, CaCl2, Na2HPO4, ZnSO4, aeration, pH and the liquid volume did not have significant effects on PHB production. In the Pareto chart, the most important factors are presented in the upper portion while lower rows indicate the least important factors. The optimum levels of the five variables, i.e. Glucose, (NH4)2SO4, FeSO4, CuSO4 and KH2PO4, were further determined by the RSM design.
Box Behnken Design Matrix for the Experimental Design of Poly-β-hydroxybutyrate (PHB) Production Media With Corresponding Results
Trails | Coded Factors | Responses PHB, g/L | |||||||
---|---|---|---|---|---|---|---|---|---|
A | B | C | D | E | Observed Glucose | Predicted Glucose | Observed Molasses | Predicted Molasses | |
1 | 0 | 0 | -1 | -1 | 0 | 1.41 | 1.97 | 2.9 | 3.81 |
2 | 0 | 1 | 1 | 0 | 0 | 1.09 | 1.60 | 3.5 | 3.18 |
3 | -1 | 0 | 1 | 0 | 0 | 0.55 | 0.83 | 0.89 | 1 |
4 | 0 | 0 | 0 | 0 | 0 | 4.02 | 4.22 | 6.62 | 6.36 |
5 | 0 | -1 | 0 | 0 | 1 | 1.12 | 1.88 | 3.9 | 3.94 |
6 | 1 | 0 | 1 | 0 | 0 | 0.29 | 0.83 | 0.58 | 1.96 |
7 | 0 | 0 | 0 | 0 | 0 | 3.85 | 4.22 | 5.98 | 6.36 |
8 | 0 | -1 | 0 | 1 | 0 | 1.98 | 1.33 | 4.02 | 3.13 |
9 | -1 | 0 | 0 | -1 | 0 | 0.09 | 0.30 | 0.17 | 0.11 |
10 | 1 | 0 | 0 | 0 | 1 | 0.61 | 0.19 | 0.97 | 0.78 |
11 | 0 | 0 | 0 | 1 | 1 | 0.1 | 0.65 | 0.29 | 1.53 |
12 | -1 | 0 | 0 | 1 | 0 | 0.11 | 0.38 | 0.28 | 0.99 |
13 | 0 | 0 | 0 | 1 | -1 | 1.01 | 1.35 | 3.2 | 2.79 |
14 | 0 | 0 | 1 | 1 | 0 | 1.02 | 1.29 | 3.04 | 2.71 |
15 | 1 | 0 | 0 | 1 | 0 | 0.27 | 0.38 | 0.56 | 0.03 |
16 | -1 | -1 | 0 | 0 | 0 | 0.09 | 0.87 | 0.02 | 1.42 |
17 | 0 | 0 | -1 | 1 | 0 | 0.32 | 1.29 | 0.63 | 2.71 |
18 | 0 | 1 | -1 | 0 | 0 | 2.28 | 1.60 | 4.8 | 3.18 |
19 | 0 | -1 | 0 | 0 | -1 | 2.48 | 2.60 | 4.9 | 5.2 |
20 | 0 | -1 | 0 | -1 | 0 | 2.93 | 2.1 | 5.01 | 4.23 |
21 | 1 | 0 | -1 | 0 | 0 | 0.71 | 0.83 | 1.08 | 3.62 |
22 | 1 | 0 | 0 | 0 | -1 | 0.95 | 0.89 | 1.71 | 2.04 |
23 | -1 | 0 | 0 | 0 | -1 | 0.36 | 0.89 | 0.77 | 1.08 |
24 | 0 | 0 | 0 | -1 | 1 | 0.92 | 1.33 | 1.82 | 2.63 |
25 | 0 | -1 | 1 | 0 | 0 | 2.08 | 2.54 | 4.3 | 5.12 |
26 | 0 | 0 | 0 | -1 | -1 | 1.98 | 2.03 | 4.1 | 3.89 |
27 | 0 | 0 | -1 | 0 | -1 | 3.74 | 2.56 | 5.85 | 4.78 |
28 | 1 | 1 | 0 | 0 | 0 | 0.65 | 0.07 | 0.92 | 0.44 |
29 | 0 | 0 | -1 | 0 | 1 | 3.12 | 1.89 | 5.12 | 3.52 |
30 | 0 | -1 | -1 | 0 | 0 | 3.04 | 2.54 | 5.04 | 5.12 |
31 | 0 | 1 | 0 | -1 | 0 | 0.78 | 1.07 | 1.61 | 2.29 |
32 | 0 | 0 | 0 | 0 | 0 | 4.41 | 4.22 | 6.56 | 6.36 |
33 | 0 | 1 | 0 | 1 | 0 | 0.68 | 0.39 | 1.02 | 1.19 |
34 | 0 | 1 | 0 | 0 | 1 | 0.62 | 0.96 | 0.91 | 2.08 |
35 | 0 | 1 | 0 | 0 | -1 | 1 | 1.31 | 2.28 | 3.26 |
36 | -1 | 0 | -1 | 0 | 0 | 0.18 | 0.83 | 0.39 | 1 |
37 | 1 | -1 | 0 | 0 | 0 | 1.03 | 0.87 | 3.3 | 2.38 |
38 | 0 | 0 | 1 | 0 | 1 | 2.11 | 1.86 | 4.41 | 3.52 |
39 | -1 | 1 | 0 | 0 | 0 | 0.003 | 0.07 | 0.01 | 0.52 |
40 | 0 | 0 | 0 | 0 | 0 | 4.39 | 4.22 | 6.64 | 6.36 |
41 | 0 | 0 | 0 | 0 | 0 | 4.35 | 4.22 | 6.16 | 6.36 |
42 | -1 | 0 | 0 | 0 | 1 | 0.22 | 0.19 | 0.38 | 0.18 |
43 | 0 | 0 | 0 | 0 | 0 | 4.28 | 4.22 | 6.22 | 6.36 |
44 | 0 | 0 | 1 | 0 | -1 | 3.01 | 2.56 | 5.01 | 4.78 |
45 | 0 | 0 | 1 | -1 | 0 | 2.02 | 1.97 | 4.7 | 3.81 |
46 | 1 | 0 | 0 | -1 | 0 | 0.85 | 0.30 | 1.53 | 1.07 |
Estimated Effect, Regression Coefficient and Corresponding “t” and “P” Values for Poly-β-hydroxybutyrate Production in Fifteen Variable Plackett-Burman Design Experiments
Term | Effect | Coef | SE Coef | T | P |
---|---|---|---|---|---|
Constant | 17519 | 0.09627 | 18.20 | 0.000 | |
pH | -0.3459 | -0.17729 | 0.09627 | -1.80 | 0.147 |
Temperature | 0.2841 | 0.1421 | 0.09627 | 1.48 | 0.214 |
Aeration | -0.0619 | -0.0310 | 0.09627 | -0.32 | 0.764 |
Inoculum | -0.0899 | -0.0450 | 0.09627 | -0.47 | 0.665 |
Inoculum age | 0.1881 | 0.0940 | 0.09627 | 0.98 | 0.384 |
MgSO4 | 0.0001 | 0.0001 | 0.09627 | 0.00 | 1.000 |
FeSO4 | 0.8081 | 0.4041 | 0.09627 | 4.20 | 0.014 |
KH2PO4 | 0.6281 | 0.3141 | 0.09627 | 3.26 | 0.031 |
CaSO4 | -0.0719 | -0.0359 | 0.09627 | -0.37 | 0.728 |
Carbon source | 1.7101 | 0.8550 | 0.09627 | 8.88 | 0.001 |
CuSO4 | 0.6881 | 0.3440 | 0.09627 | 3.57 | 0.023 |
Na2HPO4 | 0.0181 | 0.0090 | 0.09627 | 0.09 | 0.930 |
(NH4)2SO4 | 0.8541 | 0.4271 | 0.09627 | 4.44 | 0.011 |
ZnSO4 | -0.1419 | 0.0710 | 0.09627 | -0.74 | 0.502 |
Volume | -0.5099 | -02550 | 0.09627 | -2.65 | 0.057 |
4.5. Response Surface Methodology
Chosen factors were set based on the PB analysis. Five-factor was studied at three-levels and 46 trials were done at pH 7 and temperature of 37°C. The statistical significance of the model equation was evaluated by the F-test for Analysis of Variance (ANOVA), which showed that (NH4)2SO4, CuSO4 and KH2PO4 were significant (P < 0.05) for glucose (Table 4). P-values of less than 0.05 indicated factors, which are significant at the probability level of 95%. The F-value of 27.26 implies that the model is significant (P < 0.0001). Lack of fit (P = 0.0167) also suggested that the obtained experimental data had a good fit with the model. The second order polynomial equation was obtained based on the regression analysis. For glucose the Equation is:is:

Analysis of Variance for the response surface quadratic model, presented in Table 4 for molasses, showed that effects of (NH4)2SO4 (P = 0.0002) were more significant than the those of the other variables. KH2PO4 (P = 0.0095), CuSO4 (P = 0.0213) and molasses (P = 0.0412) had significant effects on PHB production. The F-value of 25.13 is significant (P < 0.0001). The second order polynomial Equation for for molasses is:

Where A is the carbon source (g/L); B is (NH4)2SO4 (g/L); C is FeSO4 (g/L), D is CuSO4 (g/L) and E is KH2PO4 (g/L).
According to the regression equation obtained from the ANOVA the R2 for glucose was 0.8549, indicating that the model could explain 85.49% of the variability in the production of PHB. The R2 value was always between zero and one. The closer the R2 to one, the stronger is the model and the better it predicted the response. The amount for the carbon source, molasses was 0.8627. The “adjusted R2” was 0.8284 and the “predicted R2” was 0.7770, which indicated that the model as good. The “adequate precision value” of the present model was 17.271, the “adequate precision value” is an index of the signal-to-noise ratio, and values of higher than four are essential prerequisites for a model to be a good fit (Table 4).
Analysis of Variance Comparative Results of Box Behnken Design Using Glucose and Molasses as Carbon Sources a
Source | Carbon Source | Sum of Squares | df | Mean Square | F Value | P-Value, Prob > F | Note |
---|---|---|---|---|---|---|---|
Model | G | 75.39 | 8 | 9.429 | 27.26 | < 0.0001 | S |
M | 188.80 | 9 | 20.98 | 25.13 | < 0.0001 | S | |
B-(NH4)2SO4 | G | 3.58 | 1 | 3.58 | 10.35 | 0.0027 | |
M | 14.90 | 1 | 14.90 | 17.85 | 0.0002 | ||
D-CuSO4 | G | 1.88 | 1 | 1.88 | 5.45 | 0.0251 | |
M | 4.84 | 1 | 4.84 | 5.80 | 0.0213 | ||
E-KH2PO4 | G | 2.02 | 1 | 2.02 | 5.83 | 0.0208 | |
M | 6.28 | 1 | 6.28 | 7.52 | 0.0095 | ||
A2 | G | 55.74 | 1 | 55.74 | 161.23 | < 0.0001 | |
M | 143.15 | 1 | 16.74 | 20.06 | < 0.0001 | ||
B2 | G | 14.50 | 1 | 14.50 | 41.93 | < 0.0001 | |
M | 16.74 | 1 | 16.74 | 20.06 | < 0.0001 | ||
C2 | G | 6.39 | 1 | 6.39 | 18.48 | 0.0001 | |
M | 5.96 | 1 | 5.96 | 7.15 | 0.0112 | ||
D2 | G | 26.14 | 1 | 26.14 | 75.61 | < 0.0001 | |
M | 45.10 | 1 | 45.10 | 54.04 | < 0.0001 | ||
E2 | G | 11.64 | 1 | 11.64 | 33.67 | < 0.0001 | |
M | 16.58 | 1 | 16.58 | 19.86 | < 0.0001 | ||
Residual | G | 12.79 | 37 | 0.35 | |||
M | 30.05 | 36 | 0.83 | ||||
Lack of fit | G | 12.53 | 32 | 0.39 | 7.46 | 0.0167 | S |
M | 29.66 | 31 | 0.96 | 12.27 | 0.0054 | S | |
Pure error | G | 0.26 | 5 | 0.052 | |||
M | 0.39 | 5 | 0.078 | ||||
Cor total | G | 88.18 | 45 | ||||
M | 218.85 | 45 | |||||
R-squared | G | o.8549 | AdjR-Squared | 0.8236 | |||
M | 0.8627 | 0.8284 | |||||
Pred R-squared | G | o.7731 | Adeq precision | 17.691 | |||
M | 0.7770 | 17.271 |
The effect of interaction of various nutrients on PHB production by molasses and glucose as the carbon sources was studied by plotting three-dimensional response surface curves. With the optimized medium, the production of PHB was found to be 6.36 g/L of molasses and 4.21 g/L of glucose. To sum up, the solution for an optimization-based regression equation via glucose as the carbon source was as follows: glucose: 19.03 g/L, (NH4)2SO4: 1.35 g/L, FeSO4: 0.19 g/L, CuSO4: 0.50 g/L and KH2PO4: 1.23 g/L. The optimum levels of each variable with molasses as the carbon source were as follows: Molasses: 19.20 g/L, (NH4)2SO4: 1.85 g/L, FeSO4: 0.14 g/L, CuSO4: 0.13 g/L, and KH2PO4: 2.43 g/L.
Figure 2 A shows a direct relationship between PHB production and concentrations of CuSO4 and glucose. Maximum PHB production was achieved when these two factors were at an intermediate level. Maximum response for PHB production occurred at KH2PO4 and glucose concentrations of about 0.35 and 20 g/L, respectively (Figure 2 B). (NH4)2SO4 and molasses affected PHB production at concentrations of slightly less than mid-level (Figure 2 C).
Three-Dimensional Response Surface Curves Showing the Effect of Interaction of Various Components on the Production of Poly-β-hydroxybutyrate by the Isolated Strain
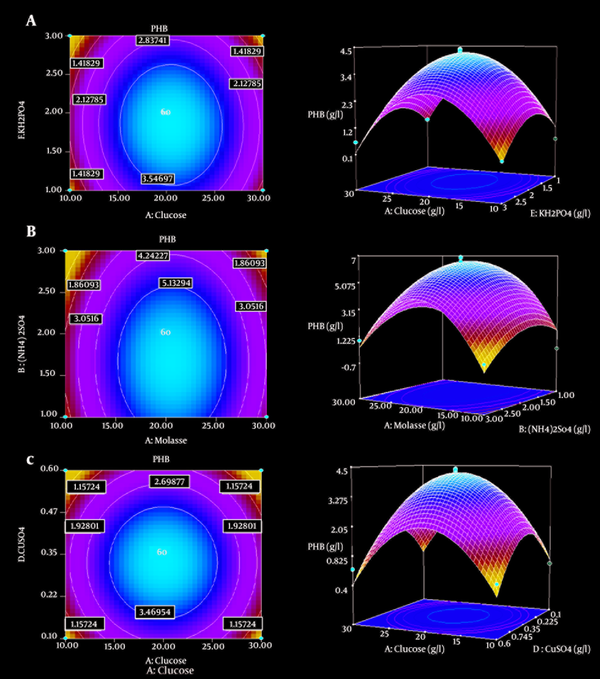
4.6. Characterization of the Isolated Bacteria
Motility test, catalase, amylase, nitrate reduction, Methyl Red (MR), growth in 2% NaCl, growth at 10, 37 and 45 - 55°C, sugar (glucose, lactose, sucrose, fructose and maltose) fermentation tests, were found to be positive. Indole, VP (Voges-Proskauer) and SH2 tests, lipase, oxidase, gelatinase and urease production, growth in 5 - 10% NaCl, and growth at 5°C were negative for the isolate.
4.7. Identification and Characterization of the Strain
The strain isolated from the oil sludge was a gram-positive rod-shaped bacteria. The biochemical characterization of the isolate revealed that the strain belonged to the genus Bacillus. 16S rRNA 16SrDNA sequence (Figure 3) and BLAST at NCBI database revealed 99.96% likelihood of the strain to Bacillus coagulans.
Polymerase Chain Reaction With Universal Primers
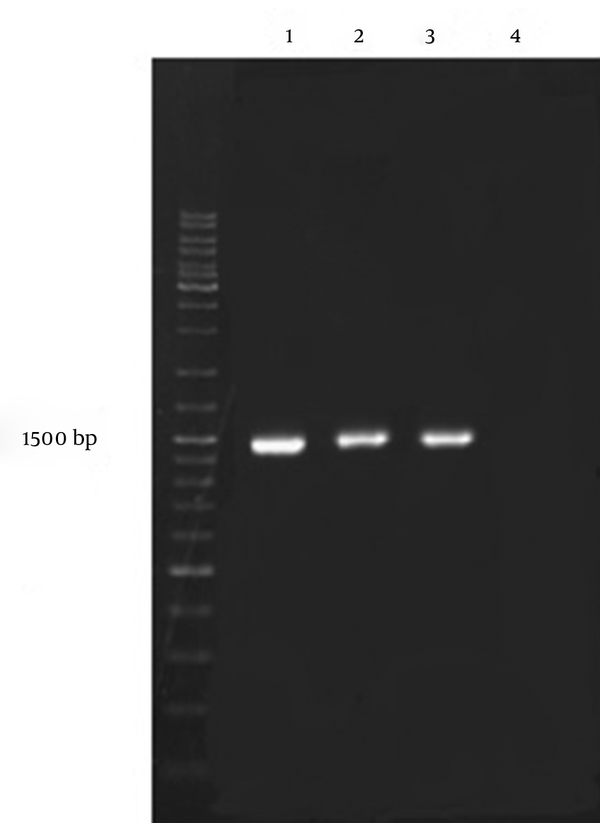
5. Discussion
Environmental pollution as a result of petrochemical plastics has created renewed interest in biologically derived polymers, and PHB has been suggested to be a suitable candidate for the solution to the problem of petroleum, based non-degradable polymers. Poly-β-hydroxybutyrate can be degraded by various microorganisms (4). Furthermore, PHBs are now employed as drug carriers to release drug slowly during degradation, providing sustained effect of the drug, thereby reducing the doses of the drug required (19). Amongst different environments including soils with different properties, sea-water and deep-sea mud have been ports of PHB-producing bacteria isolation (20). Isolation of PHB-producing bacteria from oil sludge has not yet been reported. In this study, during the initial step, 63 bacterial strains were isolated from oil sludge. Pseudomonas citronellolis, Xanthobacter, Beijerinckia, Pseudomonas, Bacillus sp. and Acinetobacter sp. are among the bacteria isolated from activated sludge oil (21).
Suitable flocs of PHB yield can be obtained via optimizing the ex-situ operating strategy (22). Sustaining a controlled residual growth improves the PHB specific production rate without altering production yield (23). In the present study, the significant variables necessary for enhanced PHB production were selected using the Plackett-Burman design. Of the 15 variables under study, five effective variables were selected by the Plackett-Burman design experiment, including glucose, (NH4)2SO4, FeSO4, CuSO4 and KH2PO4 concentrations. Mokhtari and co-workers (5) reported (NH4)2SO4 and KH2PO4 as influential factors in increasing production efficiency. Our results of PHB production by B. coagulans are in agreement with their report. Trace elements such as iron and copper play an important role in the production of PHB in B. coagulans. The validity of the RSM model is proved by fitting the values of the variables into a model equation (24).
Box-Behnken design was used to obtain optimum production of PHB by the isolated strain and the contour plots in 3D response depicted the variation in PHB yield, as a function of interaction of variables. Initially, glucose was used as the carbon source. The PHB producer, B. megaterium R11, was isolated in Singapore and could accumulate PHB up to 51.3% of its cell dry weight (CDW) from both glucose and xylose. Tryptone was identified as its best nitrogen source. Poly-β-hydroxybutyrate content and production reached 58.5% and 9.32 g/L, respectively, for an overall Oil Palm Empty Fruit Bunch (OPEFB) sugar concentration of 45 g/L. These respectively reached 51.6% and 12.48 g/L for OPEFB hydrolysate containing 60 g/L sugar with a productivity of 0.260 g/L/hour (25). In order to find a cheap carbon source, molasses was also used and the results were compared. Analysis of variance showed that the molasses was more effective than glucose in PHB production by this strain. Molasses had a greater influence on PHB concentration than glucose (P = 0.0412).
Gouda and co-workers (10) reported that glucose and molasses as carbon sources, had great effects on PHB production. Since PHB is produced under high carbon source concentrations, molasses as a good glucose and sucrose source can enhance PHB production. Lee et al. (26) tested various carbon sources from wastes such as whey, cane and sugar beet molasses for production of PHB. They also used a mixture of different salts as mineral source. (NH4)2SO4 is a good nitrogen source and a growth factor in PHB production (P = 0.002). (NH4)2SO4 has been used as the principal nitrogen source for optimal microbial growth and PHB production by Alcaligenes eutrophus and Halomonas boliviensis (27). It has been observed that the composition of trace elements in solutions also play important roles in the metabolic regulation of PHB accumulation in bacterial cells (28). Maximum PHB concentration could be achieved using molasses as carbon and (NH4)2SO4 as nitrogen sources, and KH2PO4 and CuSO4 at medium concentrations. Poly-β-hydroxybutyrate obtained using molasses in this study was 6.36 g/L. This is comparable to maximum PHB production of 5.41 g/L of B. megaterium (12) using molasses in batch culture.
A statistical design combining PB with RSM offers an efficient and feasible approach for PHB medium optimization. Furthermore, the optimum culture medium obtained in this experiment provided the basis for further studies in culture conditions with batch or fed-batch cultivation in a bioreactor for large-scale production. Finally, our results suggest that B. coagulans is a good candidate for the fermentative production of this biopolymer.
Acknowledgements
References
-
1.
Bucci DZ, Tavares LBB, Sell I. PHB packaging for the storage of food products. Polymer Testing. 2005;24(5):564-71. https://doi.org/10.1016/j.polymertesting.2005.02.008.
-
2.
Suresh Kumar M, Mudliar SN, Reddy KM, Chakrabarti T. Production of biodegradable plastics from activated sludge generated from a food processing industrial wastewater treatment plant. Bioresour Technol. 2004;95(3):327-30. [PubMed ID: 15288276]. https://doi.org/10.1016/j.biortech.2004.02.019.
-
3.
Choi J, Lee SY. Process analysis and economic evaluation for Poly(3-hydroxybutyrate) production by fermentation. Biopr Engin. 1997;17(6):335. https://doi.org/10.1007/s004490050394.
-
4.
Verlinden RA, Hill DJ, Kenward MA, Williams CD, Radecka I. Bacterial synthesis of biodegradable polyhydroxyalkanoates. J Appl Microbiol. 2007;102(6):1437-49. [PubMed ID: 17578408]. https://doi.org/10.1111/j.1365-2672.2007.03335.x.
-
5.
Mokhtari-Hosseini ZB, Vasheghani-Farahani E, Heidarzadeh-Vazifekhoran A, Shojaosadati SA, Karimzadeh R, Khosravi Darani K. Statistical media optimization for growth and PHB production from methanol by a methylotrophic bacterium. Bioresour Technol. 2009;100(8):2436-43. [PubMed ID: 19121581]. https://doi.org/10.1016/j.biortech.2008.11.024.
-
6.
Lee SY. Plastic bacteria? Progress and prospects for polyhydroxyalkanoate production in bacteria. Trend Biotech. 1996;14(11):431-8. https://doi.org/10.1016/0167-7799(96)10061-5.
-
7.
Tamber H, Johansen P, Merkle HP, Gander B. Formulation aspects of biodegradable polymeric microspheres for antigen delivery. Adv Drug Deliv Rev. 2005;57(3):357-76. [PubMed ID: 15560946]. https://doi.org/10.1016/j.addr.2004.09.002.
-
8.
Holmes PA. Applications of PHB - a microbially produced biodegradable thermoplastic. Phys Technol. 1985;16(1):32-6. https://doi.org/10.1088/0305-4624/16/1/305.
-
9.
Beaulieu M, Beaulieu Y, Melinard J, Pandian S, Goulet J. Influence of Ammonium Salts and Cane Molasses on Growth of Alcaligenes eutrophus and Production of Polyhydroxybutyrate. Appl Environ Microbiol. 1995;61(1):165-9. [PubMed ID: 16534900].
-
10.
Gouda MK, Swellam AE, Omar SH. Production of PHB by a Bacillus megaterium strain using sugarcane molasses and corn steep liquor as sole carbon and nitrogen sources. Microbiol Res. 2001;156(3):201-7. [PubMed ID: 11716209]. https://doi.org/10.1078/0944-5013-00104.
-
11.
Yu L, Lei T, Ren X, Pei X, Feng Y. Response surface optimization of l-(+)-lactic acid production using corn steep liquor as an alternative nitrogen source by Lactobacillus rhamnosus CGMCC 1466. Biochem Engin J. 2008;39(3):496-502. https://doi.org/10.1016/j.bej.2007.11.008.
-
12.
Kim BS. Production of poly(3-hydroxybutyrate) from inexpensive substrates. Enzym Microbial Tec. 2000;27(10):774-7. https://doi.org/10.1016/s0141-0229(00)00299-4.
-
13.
Burdon KL. Fatty Material in Bacteria and Fungi Revealed by Staining Dried, Fixed Slide Preparations. J Bacteriol. 1946;52(6):665-78. [PubMed ID: 16561232].
-
14.
Slepecky RA, Law JH. SYNTHESIS AND DEGRADATION OF POLY-beta-HYDROXYBUTYRIC ACID IN CONNECTION WITH SPORULATION OF BACILLUS MEGATERIUM. J Bacteriol. 1961;82(1):37-42. [PubMed ID: 16561914].
-
15.
Law KH, Cheng YC, Leung YC, Lo WH, Chua H, Yu HF. Construction of recombinant Bacillus subtilis strains for polyhydroxyalkanoates synthesis. Biochem Engin J. 2003;16(2):203-8. https://doi.org/10.1016/s1369-703x(03)00039-1.
-
16.
Lageveen RG, Huisman GW, Preusting H, Ketelaar P, Eggink G, Witholt B. Formation of Polyesters by Pseudomonas oleovorans: Effect of Substrates on Formation and Composition of Poly-(R)-3-Hydroxyalkanoates and Poly-(R)-3-Hydroxyalkenoates. Appl Environ Microbiol. 1988;54(12):2924-32. [PubMed ID: 16347790].
-
17.
Holt JG, Krieg NR, Sneath PHA, Staley JT, Williams ST. Endospore-forming gram-positive rods and cocci. Bergey's Manual of Determinative Bacteriology. Baltimore USA: William and Wilkins; 1994. 559 p.
-
18.
Reid NM, Bowers TH, Lloyd-Jones G. Bacterial community composition of a wastewater treatment system reliant on N2 fixation. Appl Microbiol Biotechnol. 2008;79(2):285-92. [PubMed ID: 18368406]. https://doi.org/10.1007/s00253-008-1413-6.
-
19.
Pouton CW, Akhtar S. Biosynthetic polyhydroxyalkanoates and their potential in drug delivery. Advanced Drug Delivery Rev. 1996;18(2):133-62. https://doi.org/10.1016/0169-409x(95)00092-l.
-
20.
Arun A, Arthi R, Shanmugabalaji V, Eyini M. Microbial production of poly-beta-hydroxybutyrate by marine microbes isolated from various marine environments. Bioresour Technol. 2009;100(7):2320-3. [PubMed ID: 19101142]. https://doi.org/10.1016/j.biortech.2008.08.037.
-
21.
Verma S, Bhargava R, Pruthi V. Oily sludge degradation by bacteria from Ankleshwar, India. I Biodeterioration & Biodegra. 2006;57(4):207-13. https://doi.org/10.1016/j.ibiod.2006.02.004.
-
22.
Ruan YJ, Zhu L, Xu XY. Study on the flocs poly-beta-hydroxybutyrate production and process optimization in the bio-flocs technology system. Bioresour Technol. 2011;102(16):7599-602. [PubMed ID: 21664127]. https://doi.org/10.1016/j.biortech.2011.05.028.
-
23.
Grousseau E, Blanchet E, Deleris S, Albuquerque MG, Paul E, Uribelarrea JL. Impact of sustaining a controlled residual growth on polyhydroxybutyrate yield and production kinetics in Cupriavidus necator. Bioresour Technol. 2013;148:30-8. [PubMed ID: 24035890]. https://doi.org/10.1016/j.biortech.2013.08.120.
-
24.
Deepak V, Kalishwaralal K, Ramkumarpandian S, Babu SV, Senthilkumar SR, Sangiliyandi G. Optimization of media composition for Nattokinase production by Bacillus subtilis using response surface methodology. Bioresour Technol. 2008;99(17):8170-4. [PubMed ID: 18430568]. https://doi.org/10.1016/j.biortech.2008.03.018.
-
25.
Zhang Y, Sun W, Wang H, Geng A. Polyhydroxybutyrate production from oil palm empty fruit bunch using Bacillus megaterium R11. Bioresour Technol. 2013;147:307-14. [PubMed ID: 24001560]. https://doi.org/10.1016/j.biortech.2013.08.029.
-
26.
Lee YH, Park JS, Huh TL. Biotech Letters. 1997;19(8):771-4. https://doi.org/10.1023/a:1018340310160.
-
27.
Quillaguaman J, Doan-Van T, Guzman H, Guzman D, Martin J, Everest A, et al. Poly(3-hydroxybutyrate) production by Halomonas boliviensis in fed-batch culture. Appl Microbiol Biotechnol. 2008;78(2):227-32. [PubMed ID: 18097660]. https://doi.org/10.1007/s00253-007-1297-x.
-
28.
Grothe E, Moo-Young M, Chisti Y. Fermentation optimization for the production of poly(β-hydroxybutyric acid) microbial thermoplastic. Enzyme and Microbial Tec. 1999;25(1-2):132-41. https://doi.org/10.1016/s0141-0229(99)00023-x.