Abstract
Background:
Helicobacter pylori is a common cause of chronic infections worldwide. Helicobacter pylori urease is a multimeric enzyme with a molecular weight of 530 kDa, which is collected from two separate subunits of UreA (26.5 kDa) and UreB (61.7 kDa).Objectives:
This study aimed to evaluate the antigenic properties of UreB recombinant protein as a vaccine candidate for the infected sera of humans and mice.Methods:
In this study, the highly antigenic region of UreB gene (609 bp) was detected, using immunological bioinformatics for epitope mapping, amplified by polymerase chain reaction (PCR). Afterwards, the antigen was cloned, using a pBSK cloning vector and was inserted into a pET-32a expression vector; the target protein was then expressed and purified. Eventually, antigenicity was examined by immunoblotting on the sera of humans infected with H. pylori UreB recombinant protein.Results:
The sequencing results of PCR were indicative of UreB gene cloning into the recombinant plasmid. Production of the protein was induced by isopropyl β-D thiogalactopyranoside (IPTG), and the expressed protein was purified via dialysis, using the Ni-NTA kit. In addition, the recombinant protein with a molecular weight of 42 kDa was recognized by antibodies in Western blotting.Conclusions:
The evaluation of antibodies was indicative of high antigenic properties for the immunogenic fragment, predicted by immunological bioinformatics. Therefore, UreB recombinant protein might be a proper antigen for the development of vaccines against H. pylori and other diagnostic kits.Keywords
1. Background
Helicobacter pylori is a Gram-negative bacterium, which was first isolated by Marshall and Warren from the gastric mucosa of patients with gastritis and peptic ulcers (1). Helicobacter pylori is the main cause of chronic infection in gastric mucosa, affecting 50% of the world’s population (2). Recently, H. pylori has been shown to cause other complications, such as gastric ulcers, duodenal inflammation, and gastritis (3, 4). According to the literature, a considerable proportion of patients, infected with H. pylori, may not develop gastroduodenal diseases and could remain asymptomatic for a long period (2). On the other hand, long-term infections raise the risk of conditions such as adenocarcinoma and gastric lymphoma (4, 5).
Several studies have confirmed H. pylori as an ultimate carcinogenic agent. The mortality rate, associated with Helicobacter pylori infection, has been estimated at 1 case per 34 infected men and 1 case per 60 infected women (4, 6). Helicobacter pylori produces a large amount of urease (10% - 15% of the whole protein weight) (2, 3, 7), which is required for the survival and pathogenesis of H. pylori. Many enzymes reduce the acidity of the immediate environment of H. pylori by producing ammonia and carbonate from urea (4, 6).
Helicobacter pylori urease is a multimeric enzyme with a molecular weight of 550 kDa, which is collected from two separate subunits of UreA (29.5 kDa) and UreB (66 kDa) (2, 3). Recently, researchers have been concerned with the development of vaccines against H. pylori infections. Among various candidate antigens, UreB is considered to be the most effective, as immunization of mice with purified UreB results in higher immunogenicity and protection, unlike UreA (6, 8). Selection of urease as an immunization target is based on the fact that membrane proteins often elicit an immune response (4, 6, 9).
In this study, we used a prediction software program with high performance for epitope mapping. With several specific epitopes of B lymphocytes, located next to each other, this program selected the amino acid fragment of UreB gene. Moreover, the antigenic fragment was expressed and purified as a recombinant protein of Escherichia coli BL21(DE3) strain. This study aimed to present a fragment of recombinant UreB (rUreB) with significant antigenic properties, using relevant software programs.
2. Objectives
The purpose of this study was to provide a recombinant vector, comprised of the antigenic region of UreB from H. pylori, using the immunodominant epitopes of the antigen instead of the total sequence and expression in E. coli. Also, we aimed to determine its antigenicity as a vaccine candidate and assess its efficacy in the serological diagnosis of H. pylori infection in humans.
3. Methods
3.1. Preparation of Bacteria, Plasmids, and Other Reagents
In this study, H. pylori was cultured from the biopsy of dyspeptic patients, undergoing routine diagnostic endoscopy. Prior to biopsy, informed consents were obtained from all the patients for participation in the study. Culture conditions were determined, based on the findings of previous reports (9). The isolated biopsy specimens were cultured on Brucella agar (Merck, Germany), containing trimethoprim (5 µg/mL), vancomycin (10 µg/mL), and amphotericin B (2.5 µg/mL), supplemented with 5% sheep blood.
After incubation under microaerophilic conditions for 3 - 5 days at 37°C (CO2: 10%), the grown bacteria were identified as H. pylori via routine microbiological tests, including Gram staining, as well as oxidase, urease, and catalase tests. For further evaluation, we used pET-32a and pBSK vectors (Novagen Inc., USA). Moreover, the pET-32a vector was used to produce fusion proteins with a 6-histidine tag and an N-terminal T7 epitope tag. These additional amino acids increased the weight of the produced protein by up to 20 kDa.
In this study, restriction enzymes and DNA ligase were purchased from Fermentas (Lithuania), and E. coli DH5α strain (Stratagene, USA) was used for the initial cloning. In addition, recombinant pET-32a (pET-32a UreB) was transformed into E. coli BL21(DE3)pLysS (Novagen, USA) as the host strain of bacteria. For routine bacterial culture, we used the Lysogeny broth (Luria-Bertani broth, LB; Sigma, USA). The required antibiotics, including ampicillin (100 µg/mL) and chloramphenicol (34 µg/mL; Sigma, USA), were added to the LB medium (10). The chemicals were purchased from Merck (Germany), and the enzymes were obtained from Fermentas (Lithuania) and SinaClon Co. (Tehran, Iran).
3.2. Detection of the Antigenic Region
For epitope mapping, we used the UreB gene sequence (1709 bp and 569 amino acids), encoding a 61.7 kDa protein from a reference strain (NCBI GenBank, USA; accession number, NC-018939.1; protein ID, P69996.1). Moreover, B-cell epitope prediction was performed, using 4 web-based programs, including IEDB, ElliPro, DiscoTope, and IgPred (11).
3.3. DNA Isolation
DNA of H. pylori was extracted from the colonies grown on Brucella agar (Merck, Germany) in accordance with the CTAB/NaCl protocol (10). The quantity and quality of the purified DNA were assessed, using 0.8% Agarose gel electrophoresis. The results were visualized via staining with ethidium bromide (1 μg/mL) on a UV transilluminator and a spectrophotometer (260 nm) (10).
3.4. Gene Amplification
In this study, 2 specific primers were designed by the AlleleID software for polymerase chain reaction (PCR), and H. pylori UreB gene was amplified from the bacterium genome, using the PCR method. The primers used for PCR were as follows: 5’CGGGATCCGCTATGGGTCGTGTGGGT 3’ as the forward primer and 5’CCGCTCGAGGAAAATGCTAAAGAGTTGCG 3’ as the reverse primer. Both primers contained BamHІ and XhoI sites (underlined), respectively.
PCR amplification was performed in a total volume of 50 μL, containing 3 μL of template, 2 μL of each primer (10 pmol), 8 μL of MgCl2 (25 mM), 1.5 μL of dNTP, 5 μL of buffer (10X), and 1 μL of the expanded DNA polymerase (SinaClon, Iran). Gene amplification was performed under the following conditions: 1) primary denaturizing at 95°C (5 minutes), followed by 35 cycles of denaturizing at 95°C (1 minute); 2) annealing at 58°C (1 minute); 3) extension at 72°C (1 minute); and 4) further extension at 72°C (5 minutes) (10). Analysis of the PCR product was conducted, using Agarose gel electrophoresis and was visualized on a UV transilluminator.
The PCR product was purified from the Agarose gel, using the High-Pure PCR Product Purification Kit (Roche, Germany) in accordance with the manufacturer's guidelines. Afterwards, it was digested with BamHI and XhoI and inserted into the pET-32a expression vector, which was itself digested with BamHI and XhoI. Then, the PCR product and plasmid were ligated with T4 DNA ligase (SinaClon, Iran) at 22°C during 1 hour of incubation.
The competent cells of E. coli DH5α and E. coli BL21(DE3)pLysS were prepared, using CaCl2 and were used for plasmid transformation (12). Furthermore, plasmid pET-32a-UreB was transformed into competent E. coli DH5α as the primary host in order to amplify the recombinant plasmid. Subsequently, the recombinant plasmid was transformed into E. coli BL21(DE3)pLysS as the host expression protein (10). The sequence was analyzed, using dideoxy chain termination, as described by Sanger et al. (13).
3.5. Protein Production and Purification
Escherichia coli BL21(DE3)pLysS (containing UreB-pET32a) was grown overnight on 2 mL of LB medium (Merck, Germany), supplemented with ampicillin (100 µg/mL) and chloramphenicol (35 µg/mL) in a shaking incubator at 37°C. On the following day, 500 µL of the bacterial suspension was inoculated in 50 mL of the enrichment broth (containing 0.5 g of yeast extract, 1 g of Bacto-peptone, 0.1 g of glucose, 0.5 g of NaCl, 0.05 g of KCl, 0.025 g of MgCl2.6H2O, 0.025 g of CaCl2, 0.25 g of nutrient broth, 15 μL of ampicillin, and 15 μL of chloramphenicol) at 37°C with vigorous agitation at 220 rpm. The cultured cells were grown until the optical density at 600 nm reached 0.5 - 0.8.
Expression of UreB recombinant protein was induced by adding 50 µg of isopropyl-β-D thiogalactopyranoside (IPTG) to the final concentration of 1 mM. After 4 hours, it was harvested by centrifugation at 8000 rpm for 20 minutes, and the whole cell lysates were examined, using 15% sodium dodecyl sulfate-polyacrylamide gel electrophoresis (SDS-PAGE). The expressed recombinant protein was purified with Ni-NTA Agarose resin (Qiagen, USA) in accordance with the manufacturer's instructions. The purified protein was dialyzed overnight with phosphate-buffered saline (PBS; pH = 7.5) at 4°C. The quantity and quality of the purified protein were analyzed, using SDS-PAGE (15%) and spectrophotometry (260/280 nm), respectively (10).
3.6. Western Blot Analysis
In this study, Western blot analysis was used to confirm the integrity of the product. Blot analysis was performed according to the standard protocol, using the sera obtained from 15 patients with seropositive H. pylori, detected via urease test, pathological analysis, and culture studies. In addition, 15 H. pylori-negative human sera, which were detected using the aforementioned methods, were used as the negative control (1:200 dilution). After reaction with the primary antibody, the blot was washed 3 times with tris-buffered saline (TBS; 0.5 M of NaCl, 0.02 M of tris, and 0.05% Tween 20, pH = 8.5) and incubated with anti-human IgG (Abcam, UK) at 1:1000 dilution. The blots were then washed 3 times with TBS, and reactions were developed by diaminobenzidine (DAB) solution (Roche, Germany) (10).
4. Results
4.1. Mapping of the Antigenic Fragment
In the present study, UreB protein sequence could predict the antigenic fragment of B lymphocytes through epitope mapping in 5 main steps. For B-cell epitope prediction, we used the IEDB software. Initially, linear epitopes from the protein sequence were predicted, using a set of prediction methods, based on linear B-cell epitopes. Moreover, we used Emini surface accessibility prediction method, and regions with the highest availability of antigenic epitopes were identified on the surface of the protein (Table 1 and Figure 1).
The Results of the IEDB Server
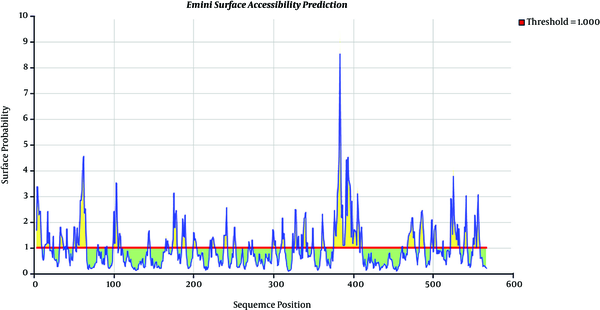
Emini Surface Accessibility Prediction of Epitope Fragments
No. | Start Position | End Position | Peptide | Peptide Length |
---|---|---|---|---|
1 | 3 | 9 | KISRKEY | 7 |
2 | 13 | 19 | YGPTTGD | 7 |
3 | 54 | 65 | GMSQSNNPSKEE | 12 |
4 | 99 | 104 | GGNKDM | 6 |
5 | 173 | 179 | TPGRRNL | 7 |
6 | 185 | 190 | AAEEYS | 6 |
7 | 375 | 407 | RTWQTADKNKKEFGRLKEEKGDNDNFRIKRYLS | 33 |
8 | 468 | 477 | PTPQPVYYRE | 10 |
9 | 483 | 488 | GKAKYD | 6 |
10 | 522 | 532 | ITKKDMQFNDT | 11 |
11 | 550 | 558 | KEVTSKPAN | 9 |
In Figure 1, peaks are above the epitopic threshold in the software, which is indicative of the desired availability of the protein. As it can be seen in this region region 374 - 407 depicts a reasonable peak in the total sequence of the protein. Dominant epitope peaks in Figure 1 indicate region 375-558 as a more favorable epitopic fragment, which is also observed in the amino acid sequence of the desired area (Table 1).
In the second stage of B-cell epitope prediction, IEDB software was used. In addition, we used Kolaskar and Tongaonkar antigenicity scale to predict and evaluate the epitopes, based on antigenicity. As presented in Figure 2, antigenic region 403 - 444 contains a peak. Considering the overlap of this region with the consensus amino acid region (observed in the first method), the results of the previous evaluation were confirmed (Table 2 and Figure 2).
The Results of the IEDB Server
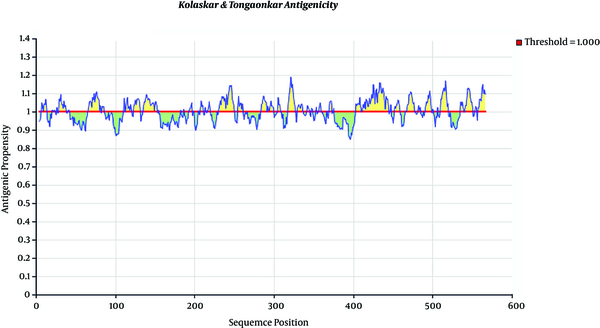
Tongaonkar and Kolaskar Antigenicity Scale of Peptides
No. | Start Position | End Position | Peptide (Sequence) | Peptide length, N. |
---|---|---|---|---|
12 | 329 | 336 | KEDVQFAD | 8 |
13 | 403 | 444 | KRYLSKYTINPAIAHGISEYVGSVEVGKVADLVLWSPAFFGV | 42 |
14 | 452 | 458 | GGFIALS | 7 |
In the third stage of B-cell epitope prediction, we used the IEDB software. Moreover, ElliPro epitope prediction was used, according to structural protrusion through which ElliPro predicts linear and discontinuous antibody epitopes, based on the 3D structure of protein antigen. In the ElliPro software, acceptable inputs could be the protein structure in the PDB format (preferred) or protein sequence. If the protein sequence is used, ElliPro initially runs the MODELLER software to model the 3D structure of the protein through homology modeling (14) (Table 3). Also, this software confirmed the consensus amino acid region.
The 4 Top Scores Predicted for B-Cell Epitopes by ElliPro Web Server
No. | Chain | Start Position | End Position | Peptide (Sequence ) | Number of Residues | Score |
---|---|---|---|---|---|---|
1 | B | 460 | 479 | MGDANASIPTPQPVYYREMF | 20 | 0.792 |
2 | B | 98 | 120 | KGGNKDMQDGVKNNLSVGPATEA | 23 | 0.778 |
3 | B | 322 | 342 | HHLDKSIKEDVQFADSRIRPQ | 21 | 0.764 |
4 | B | 494 | 511 | VSQAAYDKGIKEELGLER | 18 | 0.751 |
As presented in Table 3, the epitopic regions of UreB were scored by ElliPro software; accordingly, epitopes 479 - 460 and 511 - 494, which showed the highest scores, overlapped with the consensus amino acids, observed in the previous method. In the fourth step of B-cell epitope prediction, IEDB software was used. In addition, we used the DiscoTope method, which consists of solvent-accessible surface area calculations, as well as contact interval, in the prediction of B-cell epitope potential along the protein sequence. The antigenic regions 383 - 398 and 462 - 474 contained peaks, and the consensus amino acid regions were also confirmed in this software.
In the last stage of B-cell epitope prediction, we used IgPred software, which is a web server developed for predicting several types of B-cell epitopes that could induce different classes of antibodies, such as IgG, IgE, and IgA. In this process, with 33 amino acid sequences in region 375 - 407, the consensus amino acid area in the previous methods was predicted; this region showed the highest predicted antigenic residue scores throughout the target protein sequence; therefore, it was evaluated in the IgPred web server. The results obtained by this method are presented in Table 4, which indicate that this epitope consists of interaction scores for IgA and IgG antibodies. Between these 2 antibodies, IgA was predicted by the software, suggesting that the desired epitopic region could be the appropriate immune response, produced by the mucosal surface.
The Prediction Results of IgPred Server for Epitope 1 Consisting of Interaction Scores for IgA and IgG Antibodies
No. | Seq. ID | Peptide Sequence | IgG Score | IgE Score | IgA Score | Prediction |
---|---|---|---|---|---|---|
1 | Epitope-1 | RTWQTADKNKKEFGRLKEELGDNDNFRIKRYLS | 0.642 | -0.457 | 0.973* | IgA epitope |
2 | Epitope-2 | PTPQPVYYRE | -0.945 | -0.534 | -0.024 | Non-epitope |
According to the analysis of data obtained by high-performance bioinformatics of the server, 365 - 568 amino acid sequence contained proper dominant epitopes, and the consensus was confirmed by 5 specialized software applications, used for B-cell epitope prediction; therefore, this area was selected with utmost confidence. Therefore, based on the results, the nucleotide sequence 1092 to 1707 was considered as the region with the greatest antigenic property.
4.2. DNA Amplification
The chromosome of H. pylori was extracted for the amplification of UreB gene. The amplified DNA had the expected size of 615 bp, compared to the 100 bp DNA ladder. Then, the recombinant plasmid (pET32a-UreB) was sequenced. The results were confirmed through comparison with databases and BLAST software (data not shown).
4.3. Cloning of the Selected Antigenic Fragment
To clone the recombinant protein, the antigenic region of UreB gene was transformed into E. coli DH5α host clone by the pET-32a expression vector. Afterwards, the targeted expression was transformed into E. coli BL21(DE3)pLysS host expression, using the pET-32a expression vector. To ensure the cloning of target genes in the vectors, BamHІ and XhoI enzyme digestion sites and PCR with specific primers were used.
4.4. Expression and Purification of the Recombinant Protein
In this study, the greatest volume of the protein was produced through the expression of UreB recombinant protein in a final concentration of 1mM IPTG. The molecular weight of the recombinant protein was estimated at 22.33 kDa due to the addition of a fusion protein by the pET-32a vector. The molecular protein weight was 41/91 kDa on gel, as shown in Figure 3.
SDS PAGE Analysis: Line 1, Protein Marker (Fermentas); Lines 2 and 3, Expression of UreB Recombinant Protein at 2 and 4 Hours, Respectively; and Line 4, Purification of UreB Recombinant Protein
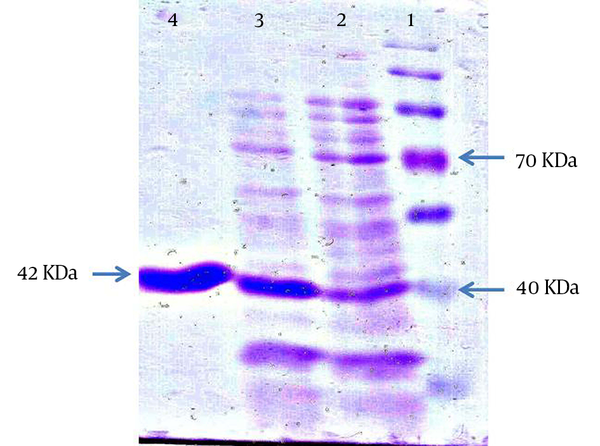
4.5. Western Blotting
In this study, antigenicity of the recombinant protein, containing the antigenic fragment of UreB gene, was determined by Western blotting with the serum obtained from patients with H. pylori infection. Bands were revealed since the purified recombinant protein reacted with the infected sera of the patients. Therefore, the recombinant protein was confirmed to have antigenic and immunogenic properties and could have specific reactions with the infected sera of humans (Figure 4).
Western Blot Analysis of Recombinant UreB: Line 1, Protein Marker (Fermentas); Line 2, 3, 4, 5 and 6, Serum of Patients with H. Pylori Infection (Positive); and Line 7, Serum of the Negative Control
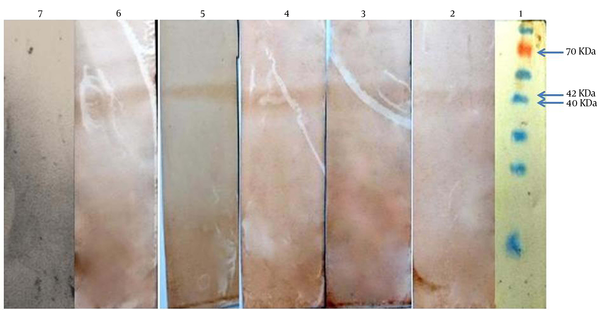
5. Discussion
Helicobacter pylori is a Gram-negative bacterium and a major cause of several gastrointestinal diseases, such as gastric and duodenal ulcers, chronic gastritis, and gastric lymphomas. Helicobacter pylori could trigger infections in the epithelial layer of human gastrointestinal tract, particularly in the gastric antrum. This bacterium is rarely isolated from the rectum, esophagus, bowel, or blood (15). Urease is considered a significant pathogenic indicator of H. pylori. This enzyme is composed of two A and B subunits and plays a pivotal role in the prevention of bacterial infections within early phases. According to the literature, antigenicity of UreB is significantly higher than UreA. As such, the majority of studies concerning the immunogenicity of urease against H. pylori have focused on UreB (12, 16). On the other hand, this antigen tends to be structurally more stable, compared to other strains of H. pylori.
UreB is a protein with a molecular weight of 66 kDa. Production and purification of this antigen from H. pylori are complicated processes due to its structure and substantial molecular weight. Consequently, most studies focusing on the production of this subunit involve the use of recombinant methods. In general, recombinant production may increase the protein weight by adding certain parts to the plasmid (eg, purification sequences and identification). Therefore, production of this protein via recombinant methods could result in fracture and lower protein yield (16). In the present study, we attempted to avoid such shortcomings to produce the protein antigenic zone, entailing the highest antigenic capacity. It is noteworthy that a smaller size of the protein is likely to increase the output and prevent fractures.
In the stimulation of immune responses for increasing vaccine effectiveness, application of different antigens of a microorganism will certainly result in stronger immune responses against the microorganism. Due to the substantial weight of proteins, it is impractical to produce different bacterial antigens in the recombinant form. Therefore, immunization against infectious agents may involve the use of antigenic indicators for these antigens instead of multiple antigens.
Given the fact that certain antigenic zones may suppress immune responses, elimination of such zones will lead to the induction of excitatory responses. However, if antigenic indicators are specified, recombinant production will be unchallenging due to the lower molecular weight; therefore, it is necessary to first specify the antigenic zone of the protein. Bioinformatic methods, especially epitope mapping, are mainly based on mathematical modeling and design of algorithms. These methods could be used to mathematically model the behavior of molecules in nature. Also, the data obtained by these methods could predict the natural reactions of molecules, such as binding antigens and antibodies (3, 7).
Epitope mapping has several mathematical modeling practices, including artificial neural networks (ANNs), quantitative matrices, decision trees, hidden Markov model (HMM), support vector machine (SVM), and scattering-matrix method (SMM). Among these approaches, ANN, SVM, and HMM are capable of analyzing linear and non-linear data. In many software applications, used to analyze the prediction of epitope zones, the aforementioned methods could identify linear and non-linear (spatial) epitopes through T and B lymphocytes; these methods were accordingly adopted in the current study (17).
Additional predictive approaches could be used for the identification of spatial epitopes on B lymphocytes. These methods include homology modeling, docking, and 3D threading techniques, which are widely used along with ANN, SVM, and HMM to detect spatial epitopes. In the present study, we applied these techniques as major methods of identification (7, 18). In general, methods used to identify antigenic epitopes should be structurally flexible on the protein surface, exposed to solvent, and contain charged amino acids and epitope hydrophilic amino acids (3, 18-20).
In the current study, we selected a zone with the highest antigenicity and reduced non-specific immune responses through detecting UreB gene fragment, containing important epitopes. This zone was confirmed by 5 software applications, as mentioned earlier; this fragment was selected so that it would contain all the epitopes. According to the obtained results, the recombinant protein had sufficient antigenicity and capacity to evoke immunogenicity.
In the present research, the antigenic ability of the specified zone was examined through the production of recombinant protein in UreB antigenic zone, using pET-32a plasmid expression. In the next stage, antigenicity of the protein was evaluated by Western blotting, performed on the sera collected from patients with H. pylori infection and peptic ulcers. Furthermore, Western blotting could identify the protein produced by active H. pylori-infected human sera. Therefore, it can be concluded that this protein contains epitopes similar to natural forms and could be effectively used for the development of vaccines. Also, it could be applied as a diagnostic measure for H. pylori infections in different diagnostic kits such as ELISA.
Acknowledgements
References
-
1.
Dunn BE, Vakil NB, Schneider BG, Miller MM, Zitzer JB, Peutz T, et al. Localization of Helicobacter pylori urease and heat shock protein in human gastric biopsies. Infect Immun. 1997;65(4):1181-8. [PubMed ID: 9119449].
-
2.
Labigne A, Cussac V, Courcoux P. Shuttle cloning and nucleotide sequences of Helicobacter pylori genes responsible for urease activity. J Bacteriol. 1991;173(6):1920-31. [PubMed ID: 2001995]. https://doi.org/10.1128/jb.173.6.1920-1931.1991.
-
3.
Morihara F, Fujii R, Hifumi E, Nishizono A, Uda T. Effects of vaccination by a recombinant antigen ureB138 (a segment of the beta-subunit of urease) against Helicobacter pylori infection. J Med Microbiol. 2007;56(Pt 6):847-53. [PubMed ID: 17510273]. https://doi.org/10.1099/jmm.0.47061-0.
-
4.
Begue RE, Moll A. Immunogenicity of Recombinant Helicobacter pylori Urease B Administered by Various Routes and with Different Adjuvants. Open Vaccine J. 2009;2:28-32. [PubMed ID: 20640237]. https://doi.org/10.2174/1875035400902010028.
-
5.
Lam R, Romanov V, Johns K, Battaile KP, Wu-Brown J, Guthrie JL, et al. Crystal structure of a truncated urease accessory protein UreF from Helicobacter pylori. Proteins. 2010;78(13):2839-48. [PubMed ID: 20635345]. https://doi.org/10.1002/prot.22802.
-
6.
Hirota K, Nagata K, Norose Y, Futagami S, Nakagawa Y, Senpuku H, et al. Identification of an antigenic epitope in Helicobacter pylori urease that induces neutralizing antibody production. Infect Immun. 2001;69(11):6597-603. [PubMed ID: 11598027]. https://doi.org/10.1128/IAI.69.11.6597-6603.2001.
-
7.
Rizos K, Lattemann CT, Bumann D, Meyer TF, Aebischer T. Autodisplay: efficacious surface exposure of antigenic UreA fragments from Helicobacter pylori in Salmonella vaccine strains. Infect Immun. 2003;71(11):6320-8. [PubMed ID: 14573651]. https://doi.org/10.1128/IAI.71.11.6320-6328.2003.
-
8.
Ha NC, Oh ST, Sung JY, Cha KA, Lee MH, Oh BH. Supramolecular assembly and acid resistance of Helicobacter pylori urease. Nat Struct Biol. 2001;8(6):505-9. [PubMed ID: 11373617]. https://doi.org/10.1038/88563.
-
9.
Hasanzadeh L, Ghaznavi-Rad E, Soufian S, Farjadi V, Abtahi H. Expression and Antigenic Evaluation of VacA Antigenic Fragment of Helicobacter Pylori. Iran J Basic Med Sci. 2013;16(7):835-40. [PubMed ID: 23997913].
-
10.
Mirjamali NA, Soufian S, Molaee N, Abbasian SS, Abtahi H. Cloning and expression of the enzymatic region of Streptococcal hyaluronidase. Iran J Basic Med Sci. 2014;17(9):667-72. [PubMed ID: 25691943].
-
11.
Tong JC, Tan TW, Ranganathan S. Methods and protocols for prediction of immunogenic epitopes. Brief Bioinform. 2007;8(2):96-108. [PubMed ID: 17077136]. https://doi.org/10.1093/bib/bbl038.
-
12.
Farjadi V, Abtahi H, Zolfaghari MR, Soufian S, Hasanzadeh L. Expression, purification and evaluation of antigenicity of caga antigenic fragment of helicobacter pylori. Jundishapur J Microbiol. 2013;6(9). https://doi.org/10.5812/jjm.7367.
-
13.
Sanger F, Nicklen S, Coulson AR. DNA sequencing with chain-terminating inhibitors. Proc Natl Acad Sci. 1977;74(12):5463-7. https://doi.org/10.1073/pnas.74.12.5463.
-
14.
Ponomarenko J, Bui HH, Li W, Fusseder N, Bourne PE, Sette A, et al. ElliPro: a new structure-based tool for the prediction of antibody epitopes. BMC Bioinformatics. 2008;9:514. [PubMed ID: 19055730]. https://doi.org/10.1186/1471-2105-9-514.
-
15.
Ladner RC. Mapping the epitopes of antibodies. Biotechnol Genet Eng Rev. 2007;24(1):1-30. https://doi.org/10.1080/02648725.2007.10648092.
-
16.
Tsurui H, Takahashi T. Prediction of T-cell epitope. J Pharmacol Sci. 2007;105(4):299-316. [PubMed ID: 18094522]. https://doi.org/10.1254/jphs.CR0070056.
-
17.
Li Pira G, Ivaldi F, Moretti P, Manca F. High throughput T epitope mapping and vaccine development. J Biomed Biotechnol. 2010;2010:325720. [PubMed ID: 20617148]. https://doi.org/10.1155/2010/325720.
-
18.
Haste Andersen P, Nielsen M, Lund O. Prediction of residues in discontinuous B-cell epitopes using protein 3D structures. Protein Sci. 2006;15(11):2558-67. [PubMed ID: 17001032]. https://doi.org/10.1110/ps.062405906.
-
19.
Kringelum JV, Lundegaard C, Lund O, Nielsen M. Reliable B cell epitope predictions: impacts of method development and improved benchmarking. PLoS Comput Biol. 2012;8(12). ee1002829. [PubMed ID: 23300419]. https://doi.org/10.1371/journal.pcbi.1002829.
-
20.
Abtahi H, Salmanian AH, Rafati S, Behzadian Nejad G, Mohammad Hassan Z. High level expression of recombinant ribosomal protein (L7/L12) from Brucella abortus and its reaction with infected human sera. Iran Biomed J. 2004;8(1):13-8.