Abstract
Background:
Organophosphorus hydrolase (OPH) is a type of organophosphate-degrading enzyme which is widely used in the bioremediation process.Objectives:
In this study, the periplasmic and cytoplasmic productions and the activity of recombinant OPH in Escherichia coli were investigated and compared using two pET systems (pET21a and pET26b).Materials and Methods:
The sequence encoding the opd gene was synthesized and expressed in the form of inclusion body using pET21a-opd and in the periplasmic space in pET26b-opd.Results:
Sodium dodecyl sulfate polyacrylamide gel electrophoresis (SDS-PAGE) analysis showed a band of about 37 kDa with a maximum expression level at 30°C from pET21a-opd.However, the obtained results of the periplasmic space extraction of OPH (pET26b-opd) showed a very weak band, while the cytoplasmic expression of OPH (pET21a-opd) produced a strong protein band.Conclusions:
The activities studied by the production of PNP were determined by following the increase at 410 nm. The maximum PNP was produced at 30°C with an optical density of 10.62 in the presence of cytoplasmic expression of OPH (pET21a-opd). Consequently, our results suggest cytoplasmic expression system as an appropriate candidate with a high amount of OPH in spite of inclusion body formation, which needs an additional refolding step.Keywords
1. Background
Environmental pollutions caused by organophosphorus compounds (OPs) are one of the major challenges faced by human, due to their widespread application over the past several decades both as chemical war agents and as pesticides, such as parathion, paraoxon, diazinon, chlorpyrifos (Cp), VX, sarin and soman (1, 2). Organophosphorus compounds irreversibly inhibit acetylcholine esterase (AChE), leading to irreparable damage to the nerve cells, resulting in symptoms such as hypotension, bradycardia, bronchoconstriction, and bronchial fluid accumulation, which results in the inability of respiratory muscles to work (3-5). Biodegradation is one of the efficient methods to deal with this fundamental problem (6-10). Organophosphorus hydrolase (OPH) is of great interest enzyme because of its ability to catalyze the hydrolysis of the P-O and P-S bonds in highly toxic organophosphates, which are produced using several approaches in various recombinant systems including multi-gene fusions, fusion with highly-soluble fusion partners, display on cell surface, and periplasmic secretion (11, 12).
Different systems expressing periplasmic OPH in Escherichia coli expression systems have been developed using twin-arginine translocation or sec pathway-driven pathways, involving many advantages including simplified downstream processing, enhanced biological activity, conducting in vivo activity assays due to greater access of the targeted protein to the substrate, higher product stability and solubility, N-terminal authenticity of the expressed protein, simpler and cost effective purification due to a lower protein content compared to the cytoplasm, and providing the oxidative environment required for correct protein folding (13-15). However, the low secretion efficiency, incomplete secretion of recombinant protein, insufficient capacity for secretion of overexpressed recombinant protein, the death of host cells, and proteolytic degradation of the product in E. coli periplasmic expression systems are problematic despite some successful examples (16). The intracellular production of recombinant proteins in E. coli cytoplasm which is widely used has several advantages over the secretion of recombinant proteins to the periplasm; however, improper folding of many target proteins may occur during this process.
Improper folding often results in the formation of inclusion bodies despite attempts to optimize the growth condition (17). No significant property was detected to recognize those proteins that have tendency to make inclusion bodies. Correct folding of proteins in cytoplasm can be achieved using several methods which are protein-specific, such as culturing at low temperatures, lowering the protein expression. Although the high protein production in the cytoplasm makes the purification of the soluble target proteins difficult, inclusion bodies have several advantages compared to soluble proteins including the higher accumulation in cytoplasm and increased protein yields as they are proteolysis-resistant, which could be isolated by a simple centrifugation step (15). Accordingly, choosing a suitable strategy to produce a recombinant protein is completely protein-specific.
2. Objectives
In this study, we are aimed to investigate the best approach to produce a high-level OPH enzyme with appropriated folding by comparing cytoplasmic and periplasmic expressions of OPH in an E. coli host cell.
3. Materials and Methods
3.1. Design and Optimization of pET21a-opd and pET26b-opd Construction and Transformation
The sequence encoding the OPH gene from Pseudomonas diminuta (Expasy accession No.P0A434) was designed and optimized for E. coli by the JCat software (18), Gen Script´s (http://www.genscript.com/cgi-bin/tools/rare_codon_analysis) and Mfold (19) software); then, it was synthesized (Biomatik Corp., Canada) into both pET21a and pET26b plasmids. The synthesized sequence, including a 1008-bp fragment with an additional adenine base in the pET26b plasmid, was approved using double-digestion method by 5´ BamHI and HindIII 3´enzymes. E. coli BL21 was transformed using a MicroPulser (Bio-Rad) at 1200V in a 0.1 cm electroporation cuvette. The preparation of electrocompetent cells and electroporation were carried out as described in Bio-Rad MicroPulser electroporation apparatus operating instructions and applications guide (Bio-Rad, #165 - 2100).
3.2. Bacterial Strains, Plasmids and Expression
Escherichia coli Rosetta-gami cell was used as a host cell for pET21a-opd and E. coli Bl21 was used for pET26b-opd. Cells harboring the recombinant plasmids were grown at 37°C in 15 mL LB medium with 20 mg/L kanamycin (sigma, #A1593) for pET26b-opd and 80 mg/L ampicillin (sigma, #A9393) for pET21a-opd at 150 rpm. Cells harboring the recombinant plasmids were induced with 0.5 mM isopropyl-β-D-thiogalactopyranoside (IPTG) (Sigma, #R1171) after the cells grew to an OD600 = 0.7 and then were cultured for seven hours at 18°C, 30°C and 37°C overnight.
3.3. Protein Extraction From the Periplasmic Space
Protein expression was performed based on Khodabakhsh et al. method with a little modification (20). The cultured E. coli cells containing recombinant pET26b-opd plasmid were centrifuged at 4000 × g for 20 minutes and the obtained pellet was resuspended in 3750 µL of 30mM Tris containing 20% sucrose at pH 8.0, followed by adding 100 mM ethylene diamine tetra acetic acid (EDTA) to a final concentration of 1 mM and incubation on ice for 10 minutes. Cells were then centrifuged at 8000×g for 20 minutes at 4°C; then, the supernatant was discarded. The pellet was resuspended in 5 mM MgSO4, stirred for 10 minutes in an ice bath, and centrifuged at the previous condition. The supernatant and the pellet were stored at 4°C.
3.4. Protein Extraction From the Cytoplasmic Space
For protein extraction, about 25 g of the cells harboring pET21a-opd was resuspended in 580 mL of 0.1 mol/L Tris and 20 mmol/L EDTA and homogenized with a shearing rod. Lysozyme (0.25 mg/mL) was added, followed by incubation on ice (30 minutes). Thereafter, centrifugation was carried out for 50 minutes at 4°C (13000 rpm). The pellets were resuspended in 300 mL of 0.1 mol/L Tris, 20 mmol/L EDTA, and 2.5% v/v Triton X-100 and were homogenized. After the centrifugation, the pellets were resuspended in 300 mL buffer containing 0.1 mol/L Tris, 20 mol/L EDTA, and 0.5% v/v Triton X-100 and were homogenized. The samples were then centrifuged (30 minutes, 4°C, 13000 rpm), and the pellets were resuspended in 250 mL of 0.1 mol/L Tris and 20 mmol/L EDTA. The prepared inclusion body was stored at 20°C. Sodium dodecyl sulfate polyacrylamide gel electrophoresis (SDS-PAGE) was performed on a 12% running gel using the method of Laemmli (21) and the protein bands were visualized by staining with Coomassie Brilliant Blue R250 (Merck, Darmstadt, Germany).
3.5. Activity Assay
The OPH activity assay was based on a method developed by Tang et al. (22). For each assay, 200 µL of the produced OPH in a solution fractions was added to 700 µL of 75 mM Tris-HCl buffer with 50 M CoCl2 (pH 8.0) and 100 µL of 20 mM paraoxon in 20% methanol. The reaction was conducted in a 1.5-mL plastic tube for 10 minutes at 37°C; 200 µL of the reaction medium was added to a 96-deep-well plate and the production of PNP was determined by following the increase at 410 nm (ε410 = 16500 M-1 cm-1) and one unit of OPH activity was defined as mmol PNP produced per minute. The calculation method was described by Bisswanger (23).
4. Results
4.1. Plasmid Sequencing
The molecular lengths of opd in pET21a and pET26b were 1008 bp and 1009 bp, respectively. The released fragments from pET21a-opd and pET26b-opd digestion by BamHI and HindIII restriction enzymes approved the successful cloning of the opd gene. The plasmid sequencing was performed for further confirmation of correct cloning (Figure 1).
Double-Digestion of Recombinant Plasmids
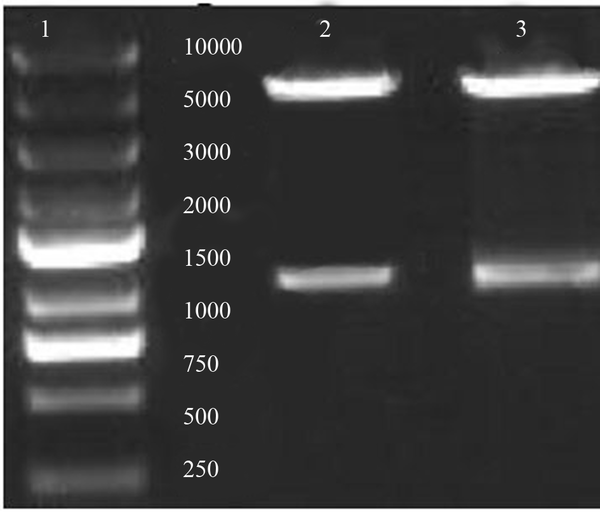
4.2. Protein Expression Analysis by Sodium Dodecyl Sulfate Polyacrylamide Gel Electrophoresis
The obtained results of cytoplasmic and periplasmic extractions of soluble and inclusion body fractions from both constructions using SDS-PAGE showed a band of about 37 kD (Figure 2). The maximum expression level was observed in fractions obtained at 30°C. The results revealed an increased expression level in both fractions in the produced OPH by the pET21a-opd construct compared to that of the pET26b, which showed high-level production and appropriated folding.
Sodium Dodecyl Sulfate Polyacrylamide Gel Electrophoresis Analysis of pET21a-opd and pET32a-opd Soluble and Insoluble Fractions
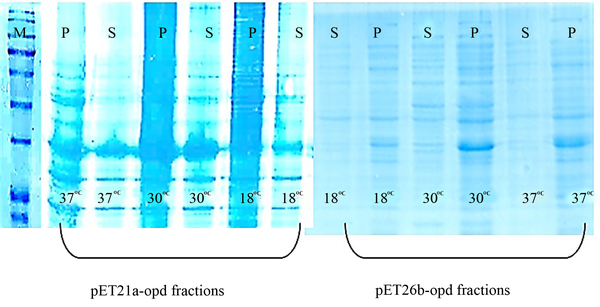
4.3. Activity Assay
The change in the PNP absorbance in the reaction solution is shown in Figure 3. By investigating the activity of the produced OPH in the solution fractions against paraoxon, most of the actively was expressed in soluble samples from the pET21a-opd construction (Figure 3). This increase in the activity was predictable due to the strong bands in the SDS-PAGE (Figure 2). The highest level of activity was observed in the produced OPH at 30°C with maximum activity of 1563.63 U/mL in the presence of the soluble fractions obtained from pET21a-opd and the lowest level of activity was at 18°C in the presence of the soluble fractions from pET26b-opd.
The Activity Assay of Soluble Fractions of pET21a-opd and pET26b-opd Enzymes
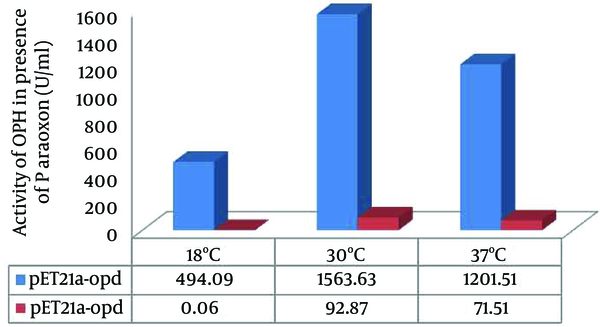
5. Discussion
In this study, cytoplasmic and periplasmic extractions of the expression of recombinant OPH protein in E. coli were investigated and compared using the pET system. pET26b bearing the N-terminal PelB signal sequence was applied for potential periplasmic localization (24). The pelB leader sequence consists of 22 N-terminal amino acid residues which can be attached to any other protein (on the DNA level), resulting in a transfer of such fused protein to the periplasmic space of Gram-negative bacteria such as E. coli, where the sequence is removed by a signal peptidase (25). pET26b vector with an N-terminal His-tag and an IPTG-inducible T7 promoter was used for the intracellular expression in E. coli. The obtained results of protein extraction from both plasmids using SDS-PAGE showed a band about 37 kD.
The periplasmic secretion of E. coli contains several advantages over intracellular production such as separation from cytoplasmic proteins, enhanced biological activity, enhanced product solubility and the ease of protein purification (26). Moreover, it is accepted that proteins with disulfide bonds could only be properly folded in the periplasm due to the existence of alkaline phosphatase, which is active only in the periplasm (27). In a study by Tang et al. methyl parathion hydrolase (MPH) was targeted to the periplasm by fusing MPH to the twin-arginine signal peptide of trimethylamine N-oxide reductase (TorA), which showed only 35% activity in the periplasm fraction and indicated that this barrier of substrate transport could be eliminated by periplasmic secretion. Although, in a study by Kang et al. the Tat-driven periplasmic secretion of OPH was presented as a potential strategy to overcome traditional substrate diffusion limitations in whole cell biocatalyst systems for detoxification of organophosphate compound (28).
The reason was the fact that in the Tat pathway, proteins are at least partially folded prior to export, without the requirement of ATP. The Tat pathway has been successfully used for periplasmic secretion of several foreign proteins such as cofactor-containing, multimeric, and disulfide-containing proteins (28). Of course, the production of many proteins such as pancreatic prekallikrein, human epidermal growth factor, protease inhibitors and t-PA in the periplasm of E. coli BL21 have shown low concentrations, misfolding and consequently inactivation in the primary trials. However, the periplasmic space extraction of pET26b plasmid showed a very weak band, which is triggered by the fact that misfolded proteins in the periplasm rapidly are degraded through stress-related degradation pathway of misfolded proteins in the periplasm by DegP protease (27, 29). Consequently, SDS-PAGE analysis and activity assay results demonstrated that cytoplasmic expression system is efficient for the production of OPH protein in prokaryotic systems; however, high amount of OPH was obtained in the form of inclusion bodies.
Cytoplasmic production also involved several advantages such as no need for outer-membrane disruption to recover target proteins and avoiding intracellular proteolysis by periplasmic proteases, leading to continuous recombinant proteins production (29). In our study, the fractions obtained by pET26b showed over-expression of the OPH protein in inclusion body forms with a weak band on SDS-PAGE for the soluble fraction. Such form is mostly composed of recombinant proteins, is protected from proteolytic degradation, and can be easily isolated from the cell debris. In addition, lower temperature significantly increases the total amount of protein due to the slower growth, which provides enough time for protein folding and reduces the rate of cell lysis and the proteolytic activity of the proteases. Conclusively, recombinant protein targeting can be performed at any compartments of cytoplasm, periplasm and the extracellular milieu. Considering the balance between advantages and disadvantages of each compartment based on the properties of the target protein is needed to determine the proper one for targeting recombinant proteins (15).Based on our results, no considerable protein production was detected in the periplasmic OPH fraction and the cytoplasmic expression of OPH showed a more efficient system for high-level expression and appropriat folding, in spite of inclusion body formation; therefore, an additional refolding step is eligible.
Acknowledgements
References
-
1.
Balali-Mood M, Saber H. Recent advances in the treatment of organophosphorous poisonings. Iran J Med Sci. 2012;37(2):74-91. [PubMed ID: 23115436].
-
2.
Colovic M, Krstic D, Petrovic S, Leskovac A, Joksic G, Savic J, et al. Toxic effects of diazinon and its photodegradation products. Toxicol Lett. 2010;193(1):9-18. [PubMed ID: 19948211]. https://doi.org/10.1016/j.toxlet.2009.11.022.
-
3.
Bardin PG, van Eeden SF, Moolman JA, Foden AP, Joubert JR. Organophosphate and carbamate poisoning. Arch Intern Med. 1994;154(13):1433-41. [PubMed ID: 8017998].
-
4.
Dormanesh B, Safarpoor Dehkordi F, Hosseini S, Momtaz H, Mirnejad R, Hoseini MJ, et al. Virulence factors and o-serogroups profiles of uropathogenic Escherichia coli isolated from Iranian pediatric patients. Iran Red Crescent Med J. 2014;16(2). eee14627. [PubMed ID: 24719745]. https://doi.org/10.5812/ircmj.14627.
-
5.
Sirin GS, Zhang Y. How is acetylcholinesterase phosphonylated by soman? An ab initio QM/MM molecular dynamics study. J Phys Chem A. 2014;118(39):9132-9. [PubMed ID: 24786171]. https://doi.org/10.1021/jp502712d.
-
6.
Blum MM, Chen JC. Structural characterization of the catalytic calcium-binding site in diisopropyl fluorophosphatase (DFPase)--comparison with related beta-propeller enzymes. Chem Biol Interact. 2010;187(1-3):373-9. [PubMed ID: 20206152]. https://doi.org/10.1016/j.cbi.2010.02.043.
-
7.
Aubert SD, Li Y, Raushel FM. Mechanism for the hydrolysis of organophosphates by the bacterial phosphotriesterase. Biochemistry. 2004;43(19):5707-15. [PubMed ID: 15134445]. https://doi.org/10.1021/bi0497805.
-
8.
Cheng T, Liu L, Wang B, Wu J, DeFrank JJ, Anderson DM, et al. Nucleotide sequence of a gene encoding an organophosphorus nerve agent degrading enzyme from Alteromonas haloplanktis. J Ind Microbiol Biotechnol. 1997;18(1):49-55. [PubMed ID: 9079288].
-
9.
Dong YJ, Bartlam M, Sun L, Zhou YF, Zhang ZP, Zhang CG, et al. Crystal structure of methyl parathion hydrolase from Pseudomonas sp. WBC-3. J Mol Biol. 2005;353(3):655-63. [PubMed ID: 16181636]. https://doi.org/10.1016/j.jmb.2005.08.057.
-
10.
Tian J, Wang P, Gao S, Chu X, Wu N, Fan Y. Enhanced thermostability of methyl parathion hydrolase from Ochrobactrum sp. M231 by rational engineering of a glycine to proline mutation. FEBS J. 2010;277(23):4901-8. [PubMed ID: 20977676]. https://doi.org/10.1111/j.1742-4658.2010.07895.x.
-
11.
Laothanachareon T, Champreda V, Sritongkham P, Somasundrum M, Surareungchai W. Cross-linked enzyme crystals of organophosphate hydrolase for electrochemical detection of organophosphorus compounds. World J Microbiol Biotechnol. 2008;24(12):3049-55. https://doi.org/10.1007/s11274-008-9851-y.
-
12.
Khodi S, Latifi AM, Saadati M, Mirzaei M, Aghamollaei H. Surface display of organophosphorus hydrolase on E. coli using N-terminal domain of ice nucleation protein InaV. J Microbiol Biotechnol. 2012;22(2):234-8. [PubMed ID: 22370355].
-
13.
Murby M, Uhlen M, Stahl S. Upstream strategies to minimize proteolytic degradation upon recombinant production in Escherichia coli. Protein Expr Purif. 1996;7(2):129-36. [PubMed ID: 8812844]. https://doi.org/10.1006/prep.1996.0018.
-
14.
Mergulhao FJ, Summers DK, Monteiro GA. Recombinant protein secretion in Escherichia coli. Biotechnol Adv. 2005;23(3):177-202. [PubMed ID: 15763404]. https://doi.org/10.1016/j.biotechadv.2004.11.003.
-
15.
Choi JH, Keum KC, Lee SY. Production of recombinant proteins by high cell density culture of Escherichia coli. Chem Eng Science. 2006;61(3):876-85. https://doi.org/10.1016/j.ces.2005.03.031.
-
16.
Wang YY, Fu ZB, Ng KL, Lam CC, Chan AK, Sze KF, et al. Enhancement of excretory production of an exoglucanase from Escherichia coli with phage shock protein A (PspA) overexpression. J Microbiol Biotechnol. 2011;21(6):637-45. [PubMed ID: 21715971].
-
17.
Soares CR, Gomide FI, Ueda EK, Bartolini P. Periplasmic expression of human growth hormone via plasmid vectors containing the lambdaPL promoter: use of HPLC for product quantification. Protein Eng. 2003;16(12):1131-8. [PubMed ID: 14983096]. https://doi.org/10.1093/protein/gzg114.
-
18.
Grote A, Hiller K, Scheer M, Munch R, Nortemann B, Hempel DC, et al. JCat: a novel tool to adapt codon usage of a target gene to its potential expression host. Nucleic Acids Res. 2005;33(Web Server issue):W526-31. [PubMed ID: 15980527]. https://doi.org/10.1093/nar/gki376.
-
19.
Zuker M. Mfold web server for nucleic acid folding and hybridization prediction. Nucleic Acids Res. 2003;31(13):3406-15. [PubMed ID: 12824337].
-
20.
Khodabakhsh F, Zia MF, Moazen F, Rabbani M, Sadeghi HM. Comparison of the cytoplasmic and periplasmic production of reteplase in Escherichia coli. Prep Biochem Biotechnol. 2013;43(7):613-23. [PubMed ID: 23768109]. https://doi.org/10.1080/10826068.2013.764896.
-
21.
Laemmli UK. Cleavage of structural proteins during the assembly of the head of bacteriophage T4. Nature. 1970;227(5259):680-5. [PubMed ID: 5432063].
-
22.
Tang X, Liang B, Yi T, Manco G, Palchetti I, Liu A. Cell surface display of organophosphorus hydrolase for sensitive spectrophotometric detection of p-nitrophenol substituted organophosphates. Enzyme Microb Technol. 2014;55:107-12. [PubMed ID: 24411452]. https://doi.org/10.1016/j.enzmictec.2013.10.006.
-
23.
Bisswanger H. Enzymes in Technical Applications. Practical Enzymology.: Wiley-VCH Verlag GmbH & Co. KGaA;. 2011. p. 297-336.
-
24.
Jappelli R, Perrin MH, Lewis KA, Vaughan JM, Tzitzilonis C, Rivier JE, et al. Expression and functional characterization of membrane-integrated mammalian corticotropin releasing factor receptors 1 and 2 in Escherichia coli. PLoS One. 2014;9(1). eee84013. [PubMed ID: 24465390]. https://doi.org/10.1371/journal.pone.0084013.
-
25.
Kusters-van Someren M, Flipphi M, de Graaff L, van den Broeck H, Kester H, Hinnen A, et al. Characterization of the Aspergillus niger pelB gene: structure and regulation of expression. Mol Gen Genet. 1992;234(1):113-20. [PubMed ID: 1495474].
-
26.
Gholipour A, Moosavian M, Galehdari H, Makvandi M, Memari HR, Alvandi A. Cloning and periplasmic expression of peptidoglycan-associated lipoprotein (PAL) protein of Legionella pneumophila in Escherichia coli. Jundishapur J Microbiol. 2010;3(1):1-9.
-
27.
Cornelis P. Expressing genes in different Escherichia coli compartments. Curr Opin Biotecchnol. 2000;11(5):450-4. https://doi.org/10.1016/S0958-1669(00)00131-2.
-
28.
Kang DG, Lim GB, Cha HJ. Functional periplasmic secretion of organophosphorous hydrolase using the twin-arginine translocation pathway in Escherichia coli. J Biotechnol. 2005;118(4):379-85. [PubMed ID: 15993968]. https://doi.org/10.1016/j.jbiotec.2005.05.002.
-
29.
Choi JH, Lee SY. Secretory and extracellular production of recombinant proteins using Escherichia coli. Appl Microbiol Biotechnol. 2004;64(5):625-35. [PubMed ID: 14966662]. https://doi.org/10.1007/s00253-004-1559-9.